1. Introduction
Aflatoxins are secondary metabolites produced by fungi of the genus Aspergillus, mainly by species A. flavus, A. parasiticus and A. nomius. These fungi naturally grow in food products and are able to initiate a variety of toxic effects in vertebrates, including humans [1]. The prevalence of tropical climate in Brazil creates ideal conditions for the development of these fungi, mainly in cereal products [2]. The main types of aflatoxins that can be found in plant substrates are identified as B1, B2, G1 and G2. Aflatoxin B1 is the most toxic compound, and its primary biotransformation in the liver undergoes detoxification reactions, which generate aflatoxins M1, Q1, B2a, P1 and aflatoxicol (AFL) [3]. These metabolites and their parent, non-metabolized compounds can ultimately be excreted in urine and feces [4,5]. Aflatoxin M1 (AFM1) is also found in milk of lactating cows that have consumed feeds contaminated with AFB1. In 1987, the International Agency for Research on Cancer classified AFB1 in Group 1-human carcinogen [6]. In past decades, several studies have demonstrated that residues of aflatoxins and their metabolites in human urine are valuable biomarkers of short-term aflatoxin exposure through the diet. Correlations between ingestion of AFB1 and excretion of AFM1 in urine were observed in Guangxi province, People’s Republic of China, Egypt and in Gambia [7–9].
The occurrence of aflatoxins in Brazilian foodstuffs has been frequently reported, mainly in peanut and corn grains at rates from up to 50% of the samples analyzed [10,11]. However, there is little information on the human exposure to aflatoxins as assessed by aflatoxin biomarkers in human urine in Brazil, except for two reports, one of them by Romero et al. [12], who observed AFM1 in 65% out of 65 urine samples from inhabitants of the city of Piracicaba, state of São Paulo with values ranging from 1.8 to 39.9 pg·mL−1 and mean concentration of 5.96 pg·mL−1 . In another recent study, Giolo et al. [13] detected AFM1 in 37.2% of 43 urine samples from chronic carriers of hepatitis B virus (HBV), and 17.2% of 29 samples from a HBV non-carriers control group. Determination of residual aflatoxins in urine in these previous works has been carried out using high performance liquid chromatography (HPLC) with fluorescence detection, and at a single sampling time. The objective of the present study was to assess the human exposure to aflatoxins in Pirassununga region, state of São Paulo, Brazil, through the determination of AFM1, AFL, and residues of AFB1, AFB2, AFG1 and AFG2 in urine using a validated method by LC coupled to mass spectrometry (MS/MS), at four sampling times over a 10-month period.
2. Results and Discussion
2.1. Method Performance
Table 1 shows the recovery and precision results estimated for all aflatoxins analyzed in urine samples. LOD and LOQ values for AFB2 and AFG1 were 0.03 and 0.10 pg·mL−1 , respectively. For AFB1, AFM1, AFG2 or AFL, the LOD and LOQ values were 0.075 and 0.25 pg·mL−1 , respectively. Calibration curves of all the aflatoxins showed coefficients of determination higher than 0.99, and residuals plot did not show tendency or deviation from linearity. Recoveries values were higher than 67% for all analytes and coefficient of variation ranged from 2% to 28%. Figure 1 shows a chromatogram of a standard mixture containing 5.0 pg·mL−1 of each aflatoxin. Taking into account that different quantification and confirmatory transitions were used for AFB2 and AFG1, there were no interferences related to co-migration of these two aflatoxins in any chromatographic run.
Table 1. Performance characteristics of the analytical method for determination of aflatoxins B1, B2, G1, G2, M1 and aflatoxicol in urine samples.
Figure 1. Chromatogram (quantification transitions) of a standard mixture containing 5 pg·mL−1 of aflatoxins B1, B2, G1, G2, M1, and aflatoxicol.
The analytical method developed was successfully validated, providing satisfactory results for the determination of residues of AFB1, AFB2, AFG1 and AFG2 and the metabolites AFM1 and aflatoxicol in urine. Special attention was given to reduce the limit of detection without decreasing recovery and precision, making feasible the first investigation of residues of AFB1, AFB2, AFG1, AFG2 and aflatoxicol in urine from Brazilian population. Furthermore, in Brazil this is the first assessment of AFM1 in urine by LC-MS/MS, where unequivocal identification of target analytes is provided.
One precursor ion and two fragment ions were used for the confirmation of each analyte, resulting in four identification points. This is higher than the minimum requirement of three identification points for the confirmation of mycotoxins, as stated by Directive 96/23/EC [14].
2.2. Analysis of Urine Samples from Volunteers
Results of AFM1 determined for each volunteer are presented in Table 2, along with mean values, standard deviation and variations of AFM1 concentration for positive samples in each sampling event, calculated and expressed as pg·mg−1 creatinine. Figure 2 shows a chromatogram of a positive urine sample for AFM1. None sample had quantifiable concentrations of AFB1, AFB2, AFG1, AFG2 and AFL. Residues of AFB1, AFB2, AFG1, and AFG2 are not commonly found in human urine samples, however, quantifiable concentrations were found in individuals from some regions of China, Egypt and Guinea with high exposure to dietary aflatoxins [8,15]. Data reported in China did not mention the mean or range of AFB1 concentrations found in urine samples [15]. However, the mean levels of urinary aflatoxins found in Egyptian children (n = 50) were 189, 1.4, 76.6 and 2.2 pg·mL−1 , for AFB1, AFB2, AFG1 and AFG2, respectively [8]. In another survey conducted in Guinea, children (n = 50) also shown AFB1 levels in urine up to 18,000 pg·mL−1 , with mean concentrations of 2.6, 5.7, 26.6 and 19.0 pg·mL−1 for AFB1, AFB2, AFG1 and AFG2, respectively [8]. The absence of AFL residues in urine in the present study is consistent with a previous report describing undetectable levels of AFL in urine samples from children in Egypt [16]. Moreover, AFL residues have been found in feces from children with Kwarshiokor [17] in human serum [16] and human placenta [18].
AFM1 was found in 39 samples (61%) at levels ranging from 0.19 to 12.7 pg·mg −1 creatinine (mean: 1.2 ± 2.0 pg·mg −1 creatinine). The mean AFM1 level found in human urine samples from Pirassununga region is much lower than the concentrations reported in early assessments conducted in areas with high incidence of aflatoxins in foods, especially in China [7,15]. Tang et al. [19] conducted an intervention study with green tea in China, and found AFM1 levels ranging from 0.42 to 141.9 pg·mg −1 creatinine at the beginning of the study. Mykkanen et al. [5] found AFM1 concentrations in urine ranging from 10 to 33 pg·mL−1 in China. In a recent evaluation accomplished in Malaysia, AFM1 mean concentration of 23.4 ±17.7 pg·mL−1 was reported by Redzwan et al. [20]. These values are much lower than former studies on the incidence of AFM1 in human urine in those countries, usually up to 5200 pg·mL−1 [7,15], but still higher than those observed in Brazil in the present study.
Table 2. Aflatoxin M1 levels in urine samples collected from volunteers 1 .
Figure 2. Chromatogram of a urine sample naturally contaminated with 0.44 pg·mL−1 . Quantification transition (329 > 273) is represented by the major signal and confirmatory transition (329 > 243) by the minor signal, respectively.
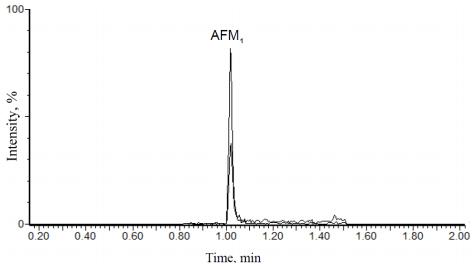
The mean concentration of AFM1 found in urine samples in the present assessment (1.3 ±1.5 pg·mL−1) was lower than previous assessments carried out in Brazil. Romero et al. [12] analyzed 69 urine samples and observed that 65% of them were positive for AFM1, with a mean value of 5.96 ±6.39 pg·mL−1 . Giolo et al. [13] found the AFM1 mean concentration for positive samples of 2.74 ± 1.98 pg·mL−1 (N = 72; range for positive samples: 0.67–7.87 pg·mL−1 ). These previous works reported data from single sampling times, while in the present study samplings were carried out four times every three months during a 10-month period. No difference (p > 0.05) was found between mean AFM1 levels in urine collected in the four sampling events. Although the mean AFM1 levels in urine samples from males in 3rd sampling (C) were higher than females (p = 0.0227), no important variations regarding to the gender in the human exposure to aflatoxins were observed in the present study.
Experimental evidence from studies with animals indicates that the excretion of AFM1 in urine is proportional to the amount of AFB1 ingested in the contaminated diet [21,22]. The correlation between AFB1 ingested and AFM1 excreted urine has also been demonstrated for humans. An epidemiological study conducted in China found AFM1 concentration in the range of 0 to 3200 pg·mL−1 in urine from volunteers with a daily intake in the range of 4 to 221 µg·day−1 [7]. Zhu et al. [7] also estimated that AFM1 excreted in urine was in the range of 1.23%–2.18% of total AFB1 ingested for male population, and 1.30%–1.78% for the female population, respectively. Taking into account the mean values of carry-over rates as estimated by Zhu et al. [7] (1.7% for males and 1.5% for females), and the mean AFM1 levels excreted in urine by volunteers in the present study (1.28 and 1.27 pg·mL−1 for males and females, respectively), Probably Daily Intake (PDI) values were estimated considering the mean volume of urine collected from volunteers (330 mL for both males and females) and the mean body weight (75 kg and 64 kg for males and females, respectively). The resulting PDI for males and females were 0.34 ng·kg−1 body weight (b.w.) day−1 and 0.42 ng·kg−1 b.w. day−1 , respectively. These PDI values are lower than the PDI value of 1.58 ± 2.5 ng·kg−1 b.w. day−1 estimated by Jager et al. [23], based on Food Frequency Questionnaires and the analysis of food products in the same region of the present study. The comparison between PDI values obtained from different methods is difficult, especially because in the present study one early morning urine sample was collected, hence representing a limited period of urine volume excreted by volunteers. However, when using the highest AFM1 value found in urine for males (6.9 pg·mL−1 ) and females (4.2 pg·mL−1 ) groups, the worst cases of aflatoxin ingestion would be 1.8 ng·kg−1 b.w. day−1 and 1.4 ng·kg−1 b.w. day−1 , respectively, which are similar to the mean PDI value as described by Jager et al. [23].
Although the carry-over of AFB1 in foods to AFM1 in urine may vary according to the level of toxin ingested and physiological differences, among other factors [21,24], the low levels of AFM1 found in the present study indicate a low human exposure to aflatoxins in the population studied. Therefore the low levels of aflatoxins in the diet of volunteers may be a consequence of annual variation in the aflatoxin occurrence in foodstuffs, or better quality control practices in the manufacture of food products in the state of São Paulo, to comply with the regulations for aflatoxins established in 2011 [25]. In Brazil, good manufacturing practices (GMP) have become compulsory for peanut industries since 2003, when the National Health Surveillance Agency issued the regulation [26]. Accordingly, low levels of up to 36.7 µg·kg−1 of total aflatoxins (B1 + B2 + G1 + G2) in peanut and corn based products and 0.069 µg·L −1 of AFM1 in liquid milk consumed in the same region studied has been reported before [23,27].
However, the fact that AFB1 and AFM1 are potent hepatocarcinogens warrants concern about the human exposure levels through the diet, as assessed by other long-term biomarkers of exposure to AFB1 such as serum AFB1-lysine adduct in the population studied.
3. Experimental Section
3.1. Sampling Design
The study was conducted in households at Pirassununga region, state of São Paulo, Brazil from June 2011 to March 2012. Residents (N = 16; 8 males and 8 females) with ages ranging from 14 to 55 years old were invited to participate as volunteers, providing urine samples four times every three months, totaling 64 samples. Volunteers were instructed to collect the early morning first urine in a polyethylene vessel previously supplied. Samples were maintained in a cool box until transport to the laboratory, where they were separated in 50 mL aliquots and kept frozen at −20 °C until analysis. The total volume of urine was measured and the body weight of each individual was recorded. The experiment was approved by the ethics committee of the School of Medicine at Ribeirão Preto, University of São Paulo.
3.2. Chemicals and Materials
All reagents were of analytical grade and water was purified by deionization (Milli-Q system, Millipore, Bedford, MA, USA). HPLC-grade acetonitrile and methanol (JT Baker, Xalostoc, Mexico) were used for chromatographic analyses. The aflatoxin standards AFB1, AFB2, AFG1, AFG2, AFM1 and AFL were purchased from Sigma (Sigma, St. Louis, MO, USA). Individual stock solutions were prepared in acetonitrile, evaluated according to Scott [28] (AOAC method 971.22), and stored in an amber glass vial at −20 °C. Individual aflatoxins B1, B2, G1, G2, M1 and AFL solutions were mixed in convenient volumes of methanol:water (50:50, v/v) to produce working solutions at concentrations starting from limit of quantitation determined for each aflatoxin and 25, 50, 75 and 100 pg·mL−1 , of each aflatoxin.
3.3. Determination of Aflatoxins in Urine
Samples by UPLC-MS/MS Samples were thawed at room temperature and centrifuged at 3500 rpm for 10 min. Twenty milliliter of sample was diluted with 20 mL of sodium acetate buffer (pH 5.0), and the mixture eluted through an immunoaffinity column Aflatest, (Vicam, Watertown, MA, USA) which was washed with 40 mL of distilled water and the retained aflatoxins eluted with 1 mL of methanol. Extract was evaporated at 40 °C until dryness and dissolved in 1 mL methanol:water (50:50, v/v). Extracts were analyzed on a Waters Acquity I-Class UPLC system (Waters, Milford, MA, USA) equipped with a BEH C18 column (2.1 × 50 mm, 1.7 μm) and coupled to a Xevo TQ-S mass spectrometer (Waters, Milford, MA, USA). The column was kept at 40 °C during analyses, and samples were maintained at 10 °C. Ten microliters of samples extracts and standards were injected. Gradient elution was employed with mobile phase composed by water (eluent A) and acetonitrile (eluent B), both containing 0.05% of aqueous ammonia. After an initial period of 0.2 min at 90% A, the percentage of B was linearly raised to 60% over 1.1 min (1.3 min). Then, eluent B was increased to 90% over 0.05 min, followed by a hold time of 0.25 min (1.60 min). Following this, the percentage of B was reduced to 10% over 0.1 min (1.7 min) and the column re-equilibrated to initial conditions for 0.8 min. Total chromatographic run time was 2.5 min and the mobile phase flow rate was maintained at 0.6 mL·min−1 . The mass spectrometer was operated in Multi Reaction Monitoring (MRM) mode using electrospray ionization in positive ion mode, with a capillary voltage of 0.75 kV, a source temperature of 150 °C and a desolvation temperature of 650 °C. Desolvation gas flow and cone gas flow were maintained at 500 L·h −1 and 150 L·h −1 , respectively. Cone voltage, collision energy and MRM transitions (major precursor ion > fragment ion) were automatically optimized for each compound individually using Intellistart program (Waters, Milford, MA, USA) of Acquity UPLC console. The most intense transition was set for quantification and the second most intense was used as confirmatory transition. Quantification MRM transitions were m/z 313 > 285, 315 > 287, 329 > 243, 331 > 245, 329 > 273, 297 > 269 for AFB1, AFB2, AFG1, AFG2, AFM1 and AFL, respectively. Confirmatory transitions were m/z 313 > 241, 315 > 259, 329 > 200, 331 > 189, 329 > 229, 297 > 115 for AFB1, AFB2, AFG1, AFG2, AFM1 and AFL. Table 3 contains the mass spectrometer conditions of analytes. Data collection and processing was performed using software MassLynx version 4.1 (Waters, Milford, MA, USA).
Table 3. Mass spectrometer conditions for detection of analytes by Multi Reaction Monitoring (MRM) method.
3.4. Method Validation for Determination of Aflatoxins Residues in Urine
Calibration curves of the aflatoxins were prepared at concentration ranges of 5.0 to 100 pg mL−1 for AFB1, AFG2, AFM1 and AFL, and 2.0 to 100 pg mL−1 for AFB2 and AFG1. These calibration levels were prepared considering the 20-fold pre-concentration of samples during the extraction procedures, hence corresponding to concentrations in urine samples of 0.25 to 5.0 pg·mL−1 for AFM1, AFB1, AFL and AFG2, and 0.1 to 5.0 pg·mL−1 for AFB2 and AFG1. The limits of detection (LOD) and quantification (LOQ) were calculated for each method of analysis based on signal: noise ratio of 3:1 and 10:1, respectively. Linearity was evaluated by verifying the coefficient of determination (r 2 ) and visual inspection of residuals plot. Triplicate injection of each concentration level was used. Recovery and precision were assessed by adding a mixture of standards to blank urine to generate fortified samples with concentrations of 0.25, 2.5 and 5.0 pg·mL−1 of each aflatoxin. All fortified samples were prepared and extracted in six replicates and final concentration was calculated by triplicate injection of each extract.
3.5. Determination of Creatinine in Urine Samples
Urinary creatinine levels were determined by a kinetic alkaline picrate (Jaffe reaction) method, using a commercial Test Kit (Labtest, Lagoa Santa, Brazil). Photometric measurements were performed using Spectrumlab 22 PC (Leng Quang Tech., Shanghai, China) at 510 nm.
3.6. Statistical Analysis
Results obtained in each sampling period were submitted to variance analysis by using the SAS® proc mixed (SAS, 2004, Cary, NC, USA), in order to verify differences among AFM1 concentrations in urine at different sampling times. The statistically significant difference was calculated with a probability level of 0.05 (p < 0.05).
4. Conclusions
Results of this trial indicate a high incidence of AFM1 at low levels in urine samples in Pirassununga region, state of São Paulo, Brazil, while AFB1, AFB2, AFG1, AFG2, and AFL were not detected in any sample. The concentrations of AFM1 found in urine samples of volunteers indicate a low short-term exposure of the population studied to aflatoxins through the diet over a 10-month period. However, further investigations are needed to assess other long-term biomarkers of exposure to AFB1 in the population under study.
Acknowledgments
The authors thank the São Paulo Research Foundation (FAPESP) and the National Council for Scientific and Technological Development (CNPq) of Brazil for financial support and fellowships.
Author Contributions
Alessandra V. Jager and Carlos A. F. Oliveira designed the project and experiments. Fernando G. Tonin optimized UPLC-MS/MS parameters. Alessandra V. Jager, Pollyana C. M. C. Souto and Rafaela T. Privatti conducted the experiments. Alessandra V. Jager and Carlos A. F. Oliveira analyzed the data and wrote the manuscript. Carlos A. F. Oliveira performed the final edition of the manuscript.
Conflicts of Interest
The authors declare no conflict of interest.
This article was originally published in Toxins 2014, 6, 1996-2007; doi:10.3390/toxins6071996. This is an Open Access article distributed under the terms and conditions of the Creative Commons Attribution license (http://creativecommons.org/licenses/by/3.0/).
References
1. Wild, C.P.; Turner, P.C. The toxicology of aflatoxins as a basis for public health decisions. Mutagenesis 2002, 17, 471–481.
2. Rodriguez-Amaya, D.B.; Sabino, M. Mycotoxin research in Brazil: The last decade in review. Braz. J. Microbiol. 2002, 33, 1–11.
3. Gross-Steinmeyer, K.; Eaton, D.L. Dietary modulation of the biotransformation and genotoxicity of aflatoxin B1. Toxicology 2012, 299, 69–79.
4. Groopman, J.D.; Zhu, J.Q.; Donahue, P.R.; Pikul, A.; Zhang, L.S.; Chen, J.S.; Wogan, G.N. Molecular dosimetry of urinary aflatoxin-DNA adducts in people living in Guangxi Autonomous Region, People’s Republic of China. Cancer Res. 1992, 52, 45–52.
5. Mykkanen, H.; Zhu, H.; Salminen, E.; Juvonen, R.O.; Ling, W.; Ma, J.; Polychronaki, N.; Kemilainen, H.; Mykkanen, O.; Salminen, S.; et al. Fecal and urinary excretion of aflatoxin B1 metabolites (AFQ1, AFM1 and AFB-N 7 -guanine) in young Chinese males. Int. J. Cancer 2005, 115, 879–884.
6. IARC monographs International Agency for Research on Cancer (IARC). Some Naturally Occurring Substances: Food Items and Constituents, Heterocyclic Aromatic Amines and Mycotoxins. In IARC Monographs on the Evaluation of Carcinogenic Risks to Humans; World Health Organization, International Agency for Research on Cancer: Lyon, France, 2002; Volume 82, pp. 171–275.
7. Zhu, J.Q.; Zhang, L.S.; Hu, X.; Xiao, Y.; Chen, J.S.; Xu, Y.C.; Fremy, J.; Chu, F.S. Correlation of dietary aflatoxin B1 levels with excretion of aflatoxin M1 in human urine. Cancer Res. 1987, 47, 1848–1852.
8. Polycrhonaki, N.; Wild, C.P.; Mykkanen, H.; Amra, H.; Abdel-Wahhab, M.; Sylla, A.; Diallo, M.; El-Nezami, H.; Turner, P.C. Urinary biomarkers of aflatoxin exposure in young children from Egypt and Guinea. Food Chem. Toxicol. 2008, 46, 519–526.
9. Groopman, J.D.; Hall, A.J.; Whittle, H.; Hudson, G.J.; Wogan, G.N.; Montesano, R.; Wild, C.P. Molecular dosimetry of aflatoxin-N 7 -guanine in human urine obtained in The Gambia, West Africa. Cancer Epidemiol. Biomark. Prev. 1992, 1, 221–227.
10. Ono, E.Y.S.; Silva, M.; Ribeiro, R.M.R.; Ono, M.A.; Silva, M.; Ono, M.A.; Hayashi, L.; Garcia, G.T.; Hirooka, E.Y. Comparison of thin-layer chromatography, spectrofluorimetry and bright greenish-yellow fluorescence test for aflatoxin detection in corn. Braz. Arch. Biol. Technol. 2010, 53, 687–692.
11. Magrine, I.C.O.; Ferrari, S.S.C.; Souza, G.F.; Minamihara, L.; Kemmelmeier, C.; Bando, E.; Machinski, M. Intake of aflatoxins through the consumption of peanutproducts in Brazil. Food Addit. Contam. B 2011, 4, 99–105.
12. Romero, A.C.; Ferreira, T.R.B.; Dias, C.T.S.; Calori-Domingues, M.A.; Gloria, E.M. Occurrence of AFM1 in urine samples of a Brazilian population and association with food consumption. Food Control 2010, 21, 554–558.
13. Giolo, M.P.; Oliveira, C.M.; Bertolini, D.A.; Lonardini, M.V.C.; Gouveia, M.S.; Netto, D.P.; Nixdorf, S.L.; Machinski, M. Aflatoxin M1 in the urine of non-carriers and chronic carriers of hepatitis B virus in Maringa, Brazil. Braz. J. Pharm. Sci. 2012, 48, 447–452.
14. European Commission. Commission decision of 12 August 2002 implementing council directive 96/23/EC concerning the performance of analytical methods and the interpretation of results. Off. J. Eur. Commun. 2002, L221, 8–36.
15. Qian, G.S.; Ross, R.K.; Yu, M.C.; Yuan, J.M.; Gao, Y.T.; Henderson, B.E.; Wogan, G.N.; Groopman, J.D. A follow-up study of urinary markers of aflatoxin exposure and liver cancer risk in Shanghai, People’s Republic of China. Cancer Epidemiol. Biomark. Prev. 1994, 3, 3–10.
16. Hatem, N.L.; Hassab, H.M.; Abd Al-Rahman, E.M.; El-Deeb, S.A.; El-Sayed Ahmed, R.L. Prevalence of aflatoxins in blood and urine of Egyptian infants with protein-energy malnutrition. Food Nutr Bull. 2005, 26, 49–56.
17. De Vries, H.R.; Maxwell, S.M.; Hendrickse, R.G. Aflatoxin excretion in children with kwashiorkor or marasmic kwashiorkor-a clinical investigation. Mycopathologia 1990, 110, 1–9.
18. Partanen, H.A.; El-Nezami, H.S.; Leppanen, J.M.; Myllynen, P.K.; Woodhouse, H.J.; Vahakangas, K.H. Aflatoxin B1 transfer and metabolism in human placenta. Toxicol. Sci. 2010, 113, 216–225.
19. Tang, L.; Meng, T.; Xu, L.; Luo, H.; Huang, T.; Yu, J.; Zhang, L.; Gao, W.; Cox, S.B.; Wang, J.-S. Modulation of aflatoxin biomarkers in human blood and urine by green tea polyphenols intervention. Carcinogenesis 2008, 29, 411–417.
20. Redzwan, S.M.; Rosita, J.; Sokhini, A.M.M.; Mohd, A.M.; Aqilah, A.R.N. Association between aflatoxin M1 excreted in human urine samples with the consumption of milk and dairy products. Bull. Environ. Contam. Toxicol. 2012, 89, 1115–1119.
21. World Health Organization. Aflatoxin M1. In WHO Food Additive Series; World Health Organization: Geneva, Switzerland, 2001; p. 47.
22. Groopman, J.D.; Hasler, J.A.; Trudel, L.J.; Pikul, A.; Donahue, P.R.; Wogan, G.N. Molecular dosimetry in rat urine of aflatoxin-N 7 -guanine and other aflatoxin metabolites by multiple monoclonal antibody affinity chromatography and immunoaffinity/high performance liquid chromatography. Cancer Res. 1992, 52, 267–274.
23. Jager, A.V.; Tedesco, M.P.; Souto, P.C.M.C.; Oliveira, C.A.F. Assessment of aflatoxin intake in São Paulo, Brazil. Food Control 2013, 33, 87–92.
24. Gan, L.S.; Skipper, P.L.; Peng, X.C.; Groopman, J.D.; Chen, J.S.; Wogan, G.N.; Tannenbaum, S.R. Serum albumin adducts in the molecular epidemiology of aflatoxin carcinogenesis: Correlation with aflatoxin B1 intake and urinary excretion of aflatoxin M1. Carcinogenesis 1988, 9, 1323–1325.
25. Agência Nacional de Vigilância Sanitária. Resolução RDC n° 7, de 18 de fevereiro de 2011. Available online: http://portal.anvisa.gov.br/wps/wcm/connect/bc17db804f45fe2cbd41fdd785749fbd/ Resolu%C3%A7%C3%A3o+0–2011-GGALI.pdf?MOD=AJPERES (accessed on 20 May 2014).
26. Agência Nacional de Vigilância Sanitária. Resolução RDC n° 172, de 4 de julho de 2003. Available Online: http://portal.anvisa.gov.br/wps/wcm/connect/503f2c00474579428651d63fbc4c6735/ RDC_172_2003.pdf?MOD=AJPERES (accessed on 1 July 2014).
27. Oliveira, C.A.F.; Gonçalves, N.B.; Rosim, R.E.; Fernandes, A.M. Determination of aflatoxins in peanut products in the northeast region of São Paulo, Brazil. Int. J. Mol. Sci. 2009, 10, 174–183.
28. Scott, P.M. Natural poisons. In Official Methods of Analysis of the Association of Official Analytical Chemists, 15th ed.; Helrich, K., Ed.; AOAC: Arlington, WA, USA, 1990; pp. 1184–1213.