a Food, Environment and Health Research Group, Faculty of Health Sciences, University of Johannesburg, South Africa;
b Biosciences, CSIR, PO Box 395, Pretoria, 001, South Africa;
c Department of Biochemistry, University of Johannesburg, South Africa;
d Department of Biochemistry, Federal University of Technology, Minna, Nigeria
Mycotoxicology, 2014, 1: 1-8
Abstract
Cytotoxicity testing of aflatoxin (AF) on the viability of cells grown in cultures can be widely used to predict the potential toxic effects of AF in animals. To this end, an in vitro experimental study was conducted to ascertain the toxic effects of AF extracts obtained from compound feeds in South Africa on human lymphocytes in comparison to that of an AFB1 standard. The approach adopted was on the basis of viable cells reducing methyl tetrazolium bromide (MTT) from blue to a purple formazan dye, which was then spectrophotometrically quantified to provide the rate of cytotoxicity. Data obtained indicated no cytotoxic response in control cells, as the viability of cells without treatment with AF standard or methanolic extracts of AF extracts (negative control) using methanol as the reconstituting solvent, was 99.9% after 24 hrs of incubation. However, cell viability significantly (p<0.001) decreased upon exposure to AF extracts especially for poultry feed. This was influenced by both the dose and duration of exposure, which was much more pronounced when the cells were exposed to AFB1 standard than for all the AF extracts tested. This implies that these feeds on exposure to AF can greatly influence animal health with respect to both the contamination dose and exposure time.
Keywords: Aflatoxins, Cytotoxicity, Lymphocyte, Methyl tetrazolium bromide
1.0 Introduction
Aflatoxins (AFs) are a group of mycotoxins produced as secondary metabolites notably by Aspergillius flavus and A. parasiticus. Other species such as A. nomius, A. bombycis, A. ochraceoroseus, A. pseudotamarii, A. tamarii, and A. australis (Ito et al., 2001; Varga et al., 2009) are also recognized producers. There are four main types of aflatoxins; aflatoxin B1 (AFB1), AFB2, AFG1 and AFG2, owing to their colour (blue and green, denoted by the letters) and retardation factor (RF) when viewed under UV light. The most important of these four toxins in terms of prevalence and abundance is AFB1. Aflatoxin B1 is also the most potent of all AFs and has been classified as a Class 1 carcinogen by the International Agency for Research on Cancer, IARC. This natural toxin is commonly reported to induce various health effects in humans and animal species; which could be hepatotoxic, mutagenic, genotoxic or carcinogenic (Wu, 2010). Chronic exposure to low levels of AF may well be a risk factor involved in the etiology of hepatocellular carcinoma and immune system suppression/dysfunction (Jiang et al., 2008; Wu et al., 2011).
Typically, AFs as metabolites are not chemically similar to those essential metabolites (e.g. polyamine) found in cells. A peculiar problem with respect to their occurrence in compound feeds is the ability of the animal to assimilate high amounts into their system. This may result in decreased animal performance and even death. The presence of the toxin in the animal’s system can be further complicated when its metabolized into animal products such as eggs, meat and milk for human consumption (Veldman et al., 1992). Therefore, it is of principal importance that compound feeds (feedstuffs or animal feeds that are formulated from various raw materials and fortified with additives) are routinely screened to check for the presence and degree of AFs contamination.
There are several approaches to this, which include direct measurements of the toxins and screening of AFs-producing fungi that gives an indication of the likely AFs presence in a commodity. However, the later approach may only give an indication of the presence of the fungal strain, but not the presence of the toxin. The fact is, some potential AF producers may not produce the toxin under certain conditions (Frisvad et al., 2006). It might be that some strains may have died before they are screened, leaving the already produced toxins in the compound feed matrix (Vosough et al., 2010). Biologically, cytotoxicity testing of AFs on human lymphocytes against extracts of compound feeds is another approach to check for the presence of toxin contamination. This approach that has been used to detect toxicity in environmental systems, involves the use of target cells, rather than using animal models (Braydich-Stolle et al., 2005). The test is rapid and cytotoxicity of the test material can be quantitatively expressed against that of an untreated control. Nevertheless, limitations are experienced in terms of false positive results. Although there is substantial financial investment in growing cell lines that have to be maintained under special aseptic working conditions (Pence, 2011). Apparently, due to the high expense, cytotoxicity-testing has been modified making use of cells available to laboratory workers i.e. blood cells, and in particular, lymphocytes. These can be used on a routine basis with ethical considerations and handled under sterile conditions. An advantage of this technique is that they do not require long maintenance periods under sterile conditions, unlike the case for cell cultures, due to shorter maturation times (Harry et al., 1998).
In this study, isolated and purified human lymphocyte cells were exposed to AFs extracts from compound feeds for investigation, as a biological means for testing the safety of these feeds.
2.0 Materials and Methods
2.1 Equipment and reagents
Unless otherwise stated, all chemicals used were of analytical grade. Tetrazolium salt [3-(4, 5- dimethylthiazol -2-yl) -2,5-diphenyltetrazolium bromide (MTT)], MTT assay kit, histopaque 1077, solvents and dimethylsulphoxide (DMSO) were purchased from Sigma Aldrich (St Louis, USA). Complete tissue culture medium (RPMI-1640 supplemented with 10% foetal calf serum (FCS) and L-glutamine were purchased from Promega Corporation (Madison, USA). Penicillin (100 U/ml) and streptomycin (100 μg/ml) were also Sigma, Aldrich. A humidified incubator (Incotherm – Labotec) was used and the temperature set at 37°C. The 96-well microtitre plates were obtained from Corning Cell WellsTM (Corning, USA). Additional materials and equipment included: Hank’s Balanced Salt Solution (Adcock Ingram), AFB1 standard (ARC, South Africa), ELISA microplate Reader (Modello: A2; Rome, Italy), centrifuge (Shalom S. Africa), haemocytometer (Shalom S. Africa) a phase contrast light microscope (Olympus B061, Wirsam Scientific, S. Africa), phytohaemagglutinin-p (PHA-p) (Sigma, Aldrich), penstrep-fungizone (Adcock Ingram), phosphate buffer saline (pH 7.4), trypan blue solution and fluorometer (Modello:A2, Rome, Italy). The PO7/V15/26.01.05 aflaprep kit with immunoaffinity column (Afla prep) was obtained from R-Biopharm Rhones Ltd (AG; Darmstadt, Germany).
2.2 Selection of aflatoxin extracts from South African compound feeds
The protocols of Candlish et al. (1998), for extracting and quantification of AFs in compound feeds, were adopted with some modifications in obtaining extracts for the present study. The milled sample (12.5 g) and 1 gram NaCl were weighed into a solvent resistant blender jar into which 62.5 ml methanol and distilled water (60:40, v/v) were added and blended for 60 s. The extract was filtered and diluted with distilled H2O (62.5 ml), which was mixed thoroughly by swirling. Sample extract (25 ml) was passed through a filter paper (Whatman No. 4) and 10 ml of the filtrate obtained (equivalent to 1 g of sample) was passed through an immuno-affinity column at a flow rate of 2–3 ml/min, after which, the immuno-affinity column was washed using 10 ml of phosphate buffered saline (PBS) at a flow rate of 5 ml/min. The analytes were then eluted (1 drop/s) using 1 ml of methanol and collected in an amber vial. Back flushing was employed thrice with the eluent to ensure complete release of AFs into the solution. The extract was dried in a fume cupboard using N2 gas and stored at 0 °C until use for further analysis. Ten AF extracts from compound feeds, mainly for poultry and cattle, were used to perform the cytotoxicity study. Selection of the feeds was based upon most significant levels of AFs analysed in the study.
2.3 Cytotoxicity testing
Aflatoxin B1 standard at concentration levels of 20, 40 and 80 μl/ml as well as AFs containing feed extracts at different concentration levels were tested in vitro to evaluate their effects on the viability of human lymphocytes after 24, 48 and 72 h of exposure. A one-way analysis of variance (ANOVA) was performed on the cytotoxicity data using a pairwise multiple comparison procedures (Holm-Sidak method). Cytotoxicity testing was done using venous blood from a healthy male volunteer after the University of Johannesburg’s Clinic Ethics Committee granted ethical clearance. Blood was collected by venous puncture, using a 2 × 15 ml sterile syringe and immediately transferred into a 10 ml heparin tube. The blood was diluted with an equal volume of RPMI-1640. Ten millilitre of the mixture was layered onto 5 ml of histopaque in a 15 ml polypropylene conical tube and then centrifuged at 1,500 rpm for 30 mins at ambient temperature. The interface layer consisting of mononuclear cells was carefully removed using a sterile pipette and washed twice with Hank‘s Balanced Salt Solution (Adcock Ingram) by centrifugation at 4°C. About 20 μl of cell suspension, 80 μl of 0.2 % trypan blue solution were mixed in an Eppendorf tube and incubated for 2 mins at room temperature. Using a sterile Pasteur pipette, 10 μl of the trypan blue cell suspension mixture was then transferred to a haemocytometer chamber and covered with a cover glass slip. Viable and non-viable cells were counted: coloured (blue) cells were considered dead while uncoloured cells excluding the dye were considered viable. Viability was determined as:
% Cell viability = (viable cell counted / total number of cells) x 100
The concentration and number of cells were calculated using the formula:
Cell/ml = (n / v) x (5) x 104
Where: n = number of cells counted
v = area of big squares counted x depth (0.1)
DF = dilution factor (10 μl of blood: 40 μl of Trypan blue) = 5
Mononuclear cells were transferred into a complete culture medium containing 1.5% Lglutamine, 10% foetal calf serum (FCS) and 1% penstrep (penicillin and streptomycin). Cells were seeded into 96 well plate containing methanol (used as a negative control), pure AFB1 standard (used as a positive control) or AF extract reconstituted in methanol and incubated in a 5% buffered and humidified incubator for 48 hrs at 65 °C.
The cytotoxicity assay performed herein is a biological method to assess the quality of compound feeds as well as a confirmatory test for the AF contents in similar feeds obtained via HPLC as reported elsewhere (Iheanacho et al., 2012). In this regard, the potential cytotoxic effect of sample extracts on lymphocytes was assessed in vitro by 3, 4, 5-dimethylthiazol-2, 5-diphenyl tetrazolium bromide (MTT) assay as described by Meky et al. (2001). This method assesses the ability of cells to convert MTT to formazan crystals. 3-(4, 5-dimethylthiazol-2, 5-diphenyl tetrazolium bromide (50 mg) was dissolved in 10 ml of 0.14 M phosphate buffered saline (PBS) (pH of 7.4) and filtered through a Whatman No.1 filter paper. About 25 μl of 5 mg/ml MTT solution was added into each cell contained in the 96 well plate and gently shaken using a vibrating shaker (Wirsam Scientific, S. Africa). The contents were incubated for 2 hrs at 37°C in a 5% buffered and humidified incubator. Thereafter, 50 μl DMSO was added to each reaction and further incubated for 4 hrs to solubilize the formazan crystals formed. A microplate reader set at a wavelength of 620 nm was used to measure optical density (OD) values. The percentage of viable cells obtained after the assay was calculated as follows:
% Cell viability = [Mean OD values of treated cells / Mean OD values of Control] × 100%.
2.4 Statistical analysis of data
A linear regression analysis was done on SigmaPlot 10.0 for Windows (Systat Inc., 2006). Data were then graphically represented. Among treatment groups, mean values were estimated to be different if the level of probability (p) was <0.05.
3.0. Results
It was observed that the viability of cells without any treatment with AFB1 standard or extracts was 99.9%, 99.7% and 99.7% after 24, 48 and 72 h, respectively, of incubation (Figures 1, 2 and 3). The viability of cells after 24 hrs exposure was strongly influenced by the concentration of AFB1 standard and sample extracts. This showed a higher reduction in cell viability when compared to the control or those of extracts with lower AFs contents as seen in Figure 1.
Fig 1. Toxic effects of aflatoxin extracts from compound feed samples and aflatoxin standard on human lymphocytes at 24 hours of exposure
Fig 2. Toxic effects of aflatoxin extracts from compound feed samples and aflatoxin standard on human lymphocytes at 48 hours of exposure
Fig 3. Toxic effects of aflatoxin extracts from compound feed samples and aflatoxin standard on human lymphocytes at 72 hours of exposure
Cell viability decreased significantly (p<0.001) over time due to continued exposure and increased AF dosage under the same conditions. The AFB1 standard (80 μl/ml) used as a point of reference exhibited the greatest cytotoxic effect in causing cell mortality (73% cell viability recorded after 24 hrs of exposure), which increased over time (59% cell viability recorded after 72 hrs of exposure). The AFs extract concentrations from compound feeds, as determined by HPLC results which have already been submitted in another study (Iheanacho et al., 2014) shows a relative correlation in respect to the determined cytotoxicity seen in this study, hence the relative cell percentage viabilities seen in Figure 1 and Tables 1, 2, 3.
4.0. Discussion
In this study, the cytotoxic effect of feed extracts containing AFs on cell viability of human lymphocytes cells in comparison to that of standard AFB1 was performed in vitro. In vitro cytotoxicity testing initially described by Jelinek (1977) on chick embryos toxicity screening test was against toxic potentials of different chemicals but not on lymphocytes against AFs. Reports of Henry and Wyatt (2001) and Sehata et al. (2004) also established the toxicity of different chemicals which include anti-tumour drugs, antipyretics, antibiotics and ergot alkaloids on chick embryo and rat brain but not on lymphocytes against AFs. The toxico-pathological potential of AFs, both for the AFB1 standard and AFs extracts from different compound feeds, to the lymphocytes was relatively high. This was observed as increased cytotoxicity (measured as decreased viability) as compared to the very low cytotoxicity posed to cells that received no treatment with AF standard or extract. A further decrease was observed over time as exposure dose increased. In overall, AFB1 standard revealed the highest toxicity as expressed by the lowest percentage lymphocyte viability when compared to that for feed extracts which also exhibited some but lower cytotoxicity tendencies. This agrees with the reports of Bünger et al. (2004) and Meky et al. (2001) in which human cell mortality increased with increasing AFs levels. Our study further reveals the implication of duration in exposure. Given the high dietary intake of AFs which led to many outbreaks of aflatoxicosis in animals reported in South Africa (Arnot et al., 2012; Otto, 2011; Van Halderen et al., 2000) and other parts of the world (Akinrinmade and Akinrinde, 2012; Thomas et al., 2010). This possible cause might be linked to the ability of this toxin causing immunosuppression as an initial step of immunomodulation due to loss of cells (Forsell et al., 1985). This immunosuppressive effect can render the animals susceptible to secondary infections; pre-disposing chickens to candidiasis, Marek‘s disease, coccidiosis and salmonellosis (Robens and Richard, 1992), pigs to erysipelas and salmonellosis and cattle to fascioliasis, intramammary and clostridial infections (Smith and Moss, 1985).
Table 1. Toxicity on Human Lymphocytes after 24 hrs
Table 2. % Cell viability of human lymphocytes induced by AF extracts in feeds after 48 hrs of exposure
Table 3. Toxicity on Human lymphocytes after 72 hrs
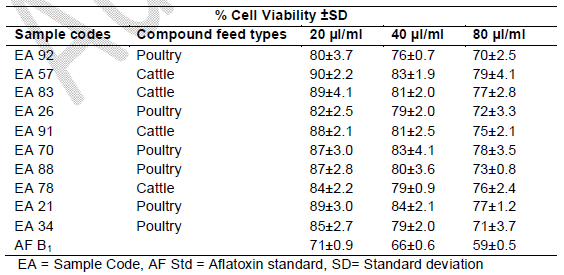
Generally, aflatoxins, mainly AFB1, are metabolized by the enzyme cytochrome P-450 enzyme (CYP450) to a toxic AFB1-epoxide that binds to cellular DNA, RNA and protein forming an adduct that possibly results in the carcinogenic effect of AFB1 in both animals and humans (Ayub and Sachan, 1997). Another mechanism of action of AFs involves the inhibition of DNA synthesis (El Khoury et al., 2011), which may result in aflatoxicosis in animals with vague initial presentations (Dixon et al., 2008). It is known, that T lymphocytes play a pivotal role in the immune system, being responsible for immune responses, acting as a natural defence mechanism against host invasion of diseases (Størmer and Lea, 1995). This may lead to a gradual but harmful onset of a disease condition. Some signs and symptoms due to such secondary infections may be lethargy and anorexia. Sometimes expressions like haematochezia, melena and haematemesis (Tedesco et al., 2004) are present as disease condition, and widespread petechiae and ecchymoses (Galvano et al., 2001) may occur. Some animals may present with peripheral oedema or ascites, polyuria and polydipsia (Wilson, 2010) in the progressive stages of the disease. Though fatality rate due to aflatoxicosis is high, its signs are also consistent with liver failures that are specific for AFs aetiology. However, the toxicity of the AFs extracts is lower than that of AF standard. One of the reasons may be due to the chemical compositions of the feed samples. Another reason could also be possibility of breakdown into residues. Animal feed consists of complex adjuncts and matrice which interfere with extraction and purification of the toxins to obtain up to 99.5% purity during laboratory analysis. It was therefore not unexpected that the pure aflatoxin standard would exert more damage to the lymphocytes. This does not in any sense downplay the cytotoxic potential of the crude hepatotoxin in vivo, especially over a period of time. Therefore, continued ingestion of the contaminated feeds may be risky to the animals.
5.0. Conclusion
The analysis of compound feeds from South Africa for AFs presence and contamination, using the MTT assay pre-analysis screening, showed feed samples with residual toxicity. The toxicity of AFB1 standard on human lymphocytes was compared to that of compound feed fraction extracts and adequate comparability of the results were observed. It was not, however, possible to deduce the biochemical mode of action of the AFB1 standard and AFs extract based on the observed reduction in cell viability potentiated by either the standard or extracts. Nevertheless, cyctotoxicity results obtained showed a high through-put, which confirms the sensitivity of the test performed to an extent in the detection of AFs contained in compound feeds. This makes it a useful biological screening tool to ascertain the quality of compound feeds.
Acknowledgement
We sincerely thank the Faculty of Health Sciences, University of Johannesburg and University of Johannesburg Alumni Network for financial support. We appreciate the effort of Njobeh, Patrick for his input from the department of Food Technology, University of Johannesburg. We sincerely thank Warren Muller for editing the English words and sentences of this work.
References
Akinrinmade, J.F. and Akinrinde, A.S. (2012). Aflatoxin status of some commercial dry dog foods in Ibadan, Nigeria. African Journal of Biotechnology, 11: 11463– 11467.
Arnot, L. F., Duncan, N. M., Coetzer, H and Botha, C. J. (2012). An outbreak of canine aflatoxicosis in Gauteng Province, South Africa. Journal of South Africa Vetinary Association, 832, 4 pages. http://dx.doi.org/10.4102/jsava.v83i1.2.
Ayub, M. Y. and Sachan, D. S. (1997). Dietary factors affecting aflatoxin Bi carcinogenicity. Malaysian Journal of Nutrition, 3: 161 - 179.
Bünger, J., Westphal, G., Mönnich, A., Hinnendahl, B., Hallier, E. and Müller, M. (2004). Cytotoxicity of occupationally and environmentally relevant mycotoxins. Toxicology, 202: 199-211.
Braydich-Stolle, L., Hussain, S., Schlager, J.J. and Hofmann, M. (2005). In vitro Cytotoxicity of Nanoparticles in mammalian Germline Stem Cells. Toxicological Sciences 88: 412-419.
Candlish, A. G., Haynes, C. A. and Stimson, W. H. (1998). Detection and determination of aflatoxins using affinity chromatography. International Journal of Food Science and Technology, 23: 479-485.
Dixon, J. B., Kannewischer, I., Tenorio arvide, M. G. and Barrientos velazquez, A. L. (2008). Aflatoxin sequestration in animal feeds by quality-labeled smectite clays: An introductory plan. Applied Clay Science, 40: 201–208.
El khoury, A., Atoui, A., Rizk, T., Lteif, R., Kallassy, M. and Lebrihi, A. (2011). Differentiation between Aspergillus flavus and Aspergillus parasiticus from pure culture and aflatoxin-contaminated grapes using PCR-RFLP analysis of aflR-aflJ intergenic spacer. Journal of Food Science, 76: 247-253.
Frisvad, J. C., Thrane, U., Samson, R. A. and Pitt, J. I. (2006). Important mycotoxins and the fungi which produce them. Adv. Exp. Med. Biol., 571: 3 - 31.
Galvano, F., Piva, A. and Ritieni, A. (2001). Dietary strategies to counteract the effects of mycotoxins: a review. Journal of food Protection, 64: 120-131.
Harry, J. G., Billingsley, M., Bruinink, A., Campbell, I. L., Classen, W., Dorman, D. C., Galli, C., Ray, D., Smith, R. A. and Tilson, H. A. (1998) Reviews in Environmental Health: Environmental Health Perspectives 106, Supplement 1, February 1998
Henry, M. H. and Wyatt, R. D. (2001). The Toxicity of Fumonisin B1, B2, and B3, Individually and in combination, in chicken embryos. Poultry Science, 80.
Iheanacho, H.E., (2012). The expression of the Nor~1 gene of Aspergillus spp. and aflatoxin production in compound feeds from South Africa in relation to animal health disorders. MTech Thesis, University of Johannesburg, South Africa. https://ujdigispace.uj.ac.za/handle/10210/ 8678
Iheanacho H.E., Dutton M.F., Steenkamp P.A., Steenkamp L., Makun H.A., Swart A. and Mthombeni, J. Q. (2014). Real time PCR of Nor~1 (a??flD) gene of aflatoxin producing fungi and its correlative quantization to aflatoxin levels in South African compound feeds. Journal of Microbiological Methods, 97: 63–67.
Ito, Y., Peterson, S. W., Wicklow, D. T. and Goto, T. (2001). Aspergillus pseudotamarii, a new aflatoxin producing species in Aspergillus section Flavi. Journal of Mycological Research, 105: 233-239.
Jelinek, R. (1977). The Chick Embryotoxicity screening test (CHEST). Methods in prenatal toxicology, Georg Thieme Publishers Stuttgart.Pp. 381-386.
Jiang Y, Jolly PE, Preko P, Wang JS, Ellis WO, Phillips TD, and Williams JH. Aflatoxin related immune dysfunction in health and in human immunodeficiency virus disease. Clin Dev Immunol 2008;doi: 10.1155/2008/790309.
Meky, F. A., Hardie, L. J., Evans, S. W. and Wild, C. P. (2001). Deoxynivalenolinducedimmunomodulation of human lymphocyte proliferation and cytokine production. Food Chemical Toxicology, 39: 827-836.
Otto, H. 2011. Tainted food kills 16 dogs. News24. Available at http://www.news24.com (Accessed June 2011).
Pence, V.C. (2011). Evaluating costs for the in vitropropagation and preservation of endangered plants. In Vitro Cellular & Developmental Biology – Plant. 47: 176-197.
Robens, J. F. and Richard, J. L. (1992). Aflatoxins in Animal and Human Health. In: WARE, G. (ed.) Reviews of Environmental Contamination and Toxicology. Springer US.
Sehata, S., Kiyosawa, N., Makino, T., Atsumi, F., Ito, K., Yamoto, Y., Teranishi, M., Baba, Y., Uetsuka, K., Nakayama, H. and Doi, K. (2004). Morphological and microarray analysis of T-2 toxin-induced rat fetal brain lesion. Food and Chemical Toxicology, 42: 1727-1736.
Smith, J. E. and Moss, M. O. (1985). Structure and formation of mycotoxins. In: Mycotoxins. Formation, analysis and significance. John Willey and Sons, Toronto, Canada, 31-49.
Systat INC. (2006). SigmaPlot 10.0 for Windows. Systat Software, Inc., Point Richmond, CA, 94804-2028, USA.
Tedesco, D., Steidler, S. and Galletti, S. (2004). Efficacy of silymarin-phospholipid complex in reducing the toxicity of aflatoxin B1 in broiler chicks. Poultry Science, 83: 1839-1843.
Thomas, W. K., Bill, D. R., Gerald, N. W. and John, D. G. (2010). Aflatoxin: A 50-Year odyssey of mechanistic and translational Toxicology. Oxford Journals of Toxicological Sciences, 120: 28-48.
Van Halderen, A., Garthwaite, I. and Wessels, J. C. (2000). Outbreak of bovine aflatoxicosis in South Africa. World Mycotoxin Journal, 4: 87-100.
Varga, J., Frisvad, J. C. and Samson, R. A. (2009). A reappraisal of fungi producing aflatoxins. World Mycotoxin Journal, 2: 263-277.
Veldman, V., Meijst, J., Borgreve, J. and Heers- Van der tol, J. (1992). Carry over of aflatoxin from cow’s food to milk. Animal Production 55: 163 - 168.
Vosough, M., Bayat, M. and Salemi, A. (2010). Matrix-free analysis of aflatoxins in pistachio nuts using parallel factor modeling of liquid chromatography diodearray detection data. Analytica chimica acta, 663: 11 - 18.
Wilson, D. (2010). Clinical Veterinary Advisor: The Horse, Elsevier Health Sciences.
Wu, F. (2010). The global burden of disease caused by foodborne aflatoxin. WHO Commissioned Report, Food
Wu, F., Narrod, C., Tiongco, M. and Liu, Y. (2011). The Health Economics of Aflatoxin: Global Burden of disease: Afla control, Working Paper 4.