INTRODUCTION
In the United States and most parts the world, the biggest portion of poultry diets is comprised of carbohydrates, with corn being the main cereal grain used in the feed industry (Moran, 1982; Ai and Jane, 2016; Agristats, 2018; IGC, 2018). Corn is dried on the field or usually dried either by constant flow or batch drying after harvesting, so it could be included in poultry feeds. It has been reported that temperatures while drying could reach more than 100C mainly to reduce energy consumption in grain dryers (Larbier et al., 1971; Barrier-Guillot et al., 1993; Li et al., 2014; Odjo et al., 2015). However, either using high temperatures and long time exposure to heat regardless the temperature while drying could result in the production of undigestible complexes that will impair the digestibility of nutrients thus depressing broiler live performance (Larbier et al., 1971; Iji et al., 2003; Cowieson, 2005; Malumba et al., 2008; Huart et al., 2018).
On the other hand, grain particle size is an important factor as it could influence several aspects of broiler gastrointestinal physiology (Celi et al., 2017). These include nutrient utilization (Benedetti et al., 2011; Kheravii et al., 2018), growth performance (Nir and Hillel, 1995; Amerah et al., 2007; Zang et al., 2009; Xu et al., 2015), feed passage rate (Hetland et al., 2002; Svihus et al., 2002), digestive tract development (Zang et al., 2009; Pacheco et al., 2014), and microbiota profile (Amerah et al., 2009). Traditionally in poultry diets, fine particles have been associated with a larger relative surface area, possibly resulting in greater digestibility due to interactions with digestive enzymes in the gastrointestinal tract (GIT) (Hetland et al., 2002; Amerah et al., 2007). This improvement in the digestibility may be by promoting enzymatic digestion and absorption (Duke, 1992; Amerah et al., 2007). In contrast, coarse particles are known to enhance GIT motility, stimulate gizzard function, and reduce pathogen population in the lower intestine, resulting in better overall digestive efficiency (Pacheco et al., 2013; Xu et al., 2015).
Modern fast-growing meat chickens require adequate amounts of energy to supply through the diet alongside with protein, particularly essential amino acids (Dozier et al., 2008; Kidd and Tillman, 2016). Most of the energy needed for fast growth of broilers comes from starch, which is quantitatively the major nutrient in broiler diets. The endosperm of corn is almost entirely composed of reserve carbohydrate, principally starch, which is present in about 65% DM basis (Jiang et al., 2007; Zaefarian et al., 2015). Alpha-amylase is the main enzyme responsible for starch digestion in mammals (Osman, 1982; Gray, 1992; Bach Knudsen et al., 2006). Although there is some alpha-amylase activity present in the saliva of humans and pigs, in poultry, salivary amylase has limited activity (Denbow, 2015; Vieira and Stefanello, 2017); therefore, pancreatic α-amylase is the only enzyme that chickens could use to degrade starch (Moran, 1982; Wiseman, 2006). Amylase has been reported to be the most abundant enzyme in the pancreas of chicks and young turkeys, nevertheless its activity specially during the first few days of life after hatch can be limited (Krogdhal and Sell, 1989; Pubols, 1991). Chickens are considered well able to digest diets containing high starch levels. Moreover, physiological differences according to age have influenced the performance of broilers chickens when comparing the starter and finisher dietary phases. Thus, as corn is included at higher levels in the finisher diets for broilers, the ratio of nonstarch polysaccharides and starch increases when compared to the starter feed (Svihus, 2014). In addition, modern broiler chickens have greater feed intake which may produce a physiological limitation to starch digestion as the feed passage rate could be increased, consequently reducing and limiting nutrient digestion and absorption (Croom et al., 1999; Sklan and Noy, 2003). Previous studies confirmed the effectiveness of exogenous amylase to improve nutrient digestibility and live performance in broiler chickens while feeding corn-based diets (Gracia et al., 2003). However, other researchers did not detect these beneficial effects (Stefanello et al., 2015). Factors such as particle size may explain the lack of consistency in results while supplementing amylase. In addition, corn may fragment different during milling depending on its postharvest drying temperature, resulting in different particle size distribution as it has been proposed in an early study (Kaczmarek et al., 2014). The possible interaction effects among postharvest drying temperature, particle size, and exogenous amylase inclusion have been poorly investigated. Therefore, the aim of the present studies was to evaluate the effects of corn-drying temperature, particle size, and amylase supplementation on broiler live performance, gastrointestinal organ development, nutrient digestibility, and energy utilization in broiler chickens.
MATERIALS AND METHODS
All procedures involving broiler chicken used in the present experiment were approved by the North Carolina State University Institutional Animal Care and Use Committee.
Chicken Husbandry
A total of 2,400 Cobb-500 day-old male Cobb 500 slow-feathering chicks were hatched, feather-sexed, and separated for 2 parallel experiments.
Experiment 1
On the day of hatching, a total of 1,920 chicks were randomly placed in 96 floor pens (2 m2) in groups of 20 birds per pen. Chicks were randomly assigned to 12 treatments with 8 replicates for each treatment. Each pen was equipped with one tubular feeder and one belt drinker. Supplemental feeders and drinkers were placed for the first 7 D of the study. Average temperature was 33°C at placement, which was reduced gradually until 20.6°C at 21 D and kept until 42 D to provide chicken environmental comfort. Used litter was reused as bedding in the pens, and broilers were exposed to continuous light on a 23L:1D (30 lux light intensity) program during the first 3 D of age. Day length was then gradually reduced to 17L:7D (10 lux) up to 28 D of age. From 28 D until the end of the experiment at 42 D, the light program was maintained at 17L:7D with an intensity of 5 lux.
Experiment 2
A total of 480 day-old chicks were distributed among 4 Petersime battery brooder units, 96 cages, with 5 chicks per cage of 0.47 m2, equipped with trough-type feeders and waterers. Feed and water were available for ad libitum consumption. Chicks were then randomly assigned to 12 treatments with 8 replicates for each treatment. The average temperature at placement was 33.0°C and gradually reduced to 26°C at 13 D according to thermal comfort of the chickens. The lighting program consisted of continuous 23 h light and 1 h of darkness (30 lux light intensity) throughout the whole experimental period of 16 D.
Grain Production, Drying, and Grinding Management
Seeds from a corn hybrid (DEKALB 65-20) with hard kernel (vitreousness value of 62.87%; Table 1) were obtained from a commercial supplier. Agricultural practices such as seed disinfection, herbicide application, and fertilization were performed to optimize on-field productivity. After 135 D of plantation, corn cobs were harvested and degrained mechanically when onfield grain moisture content was 21.95% and density 54.60 lb/bu (Table 1). Thereafter, harvested volume was split into 2 batches and transported to a GSI single-fan, natural gas, open flame, forced-air dryer (GSI, Competitor Series Dryers, model competitor 112; Newton, IL) with a capacity of 325 bushels (8.3 tons) approximately, and batch drying method was applied. The first batch was dried at 35°C and the next batch at 120°C. Temperature was monitored using 4 digital probe thermometers inserted in different locations of the dryer. The 2 batches of corn were dried separately until their moisture content was reduced for storage (Table 1). To reach this humidity in the grain, the drying process at 35°C took 9 h and 5 h at 120°C, respectively. Thereafter, the dried material was bagged in bulk bags and transported to a covered house. Grain moisture was determined using a Dickey-John grain moisture meter (Dickey-John Co. model GAC 2100b; Raleigh, NC), and vitreousness content by NIRS (DS2500, FOSS, Hillerod, Denmark). For grinding purposes, the coarse-corn (CC) and fine-corn (FC) were obtained using a hammermill (Model 1522; Roskamp Champion, Waterloo, IA) equipped with 2 #12 screens. Grinding speeds of 3,600; 3,400; and 3,400 rpm for starter, grower, and finisher, respectively, were used for the FC, whereas 1,400 rpm was used for dietary phases based on CC. These speeds were considered targeting particle size geometric mean (dgw) between 800-900 μm for the CC and 400-500 μm for the FC.
Diets and Experimental Design
The experimental design consisted of a 2 x 2 x 3 factorial arrangement in a randomized complete block design to determine the live performance, organ development, and nutrient digestibility of broiler chickens throughout both parallel studies. Corn was dried at 35°C or 120°C, grinded to obtain 2 particle sizes (varying in ˜400 μm, dgw), and labelled as either CC or fine FC. When corn was used in broiler diets, 3 dietary inclusion levels of an exogenous amylase (0, 133, and 266 g ton-1) were considered to obtain a total of 12 dietary treatments with 8 replications each for the 2 studies.
Dietary treatments (Table 2) were formulated and manufactured to meet or exceed the recommended Cobb 500 nutritional requirements (Cobb Vantress, 2018). The basal diet contained all other ingredients but corn and amylase, to reduce variation in ingredient and nutrient composition among batches. Each one of the 4 corn types (combination of drying at 35°C or 120°C, and CC or FC) were then assigned to the 3 enzyme levels (0, 133, and 266 g ton-1) for further mixing with the basal diet at the same percentage of inclusion resulting in 12 treatments for each dietary phase (Table 2). After mixing, all diets were conditioned at 85°C for 30 s in a single pass conditioner (model C18LL4/F6; California Pellet Mill, Crawfordsville, IN) and then pelleted using a 30 HP CPM pellet mill (model PM1112-2; California Pellet Mill, Crawfordsville, IN) equipped with a 4.4 x 35.2-mm die with 548 cm2 of working surface area at a production rate of 980 kg/h. The steam pressure was 207 kPa. The pellet mill die was warmed with 455 kg of feed before pelleting with the experimental feeds. After pelleting, pellets were cooled in a counter flow cooler (Model VK09X09 KL; Geelen Counterflow, Inc, Orlando, FL) and then crumbled (Model 624S; Roskamp Champion, Waterloo, IA) for the starter diets only, while grower and finisher diets were offered to chickens in pellet form. The final experimental feeds were produced from the lowest to the highest dietary inclusion level of exogenous amylase.
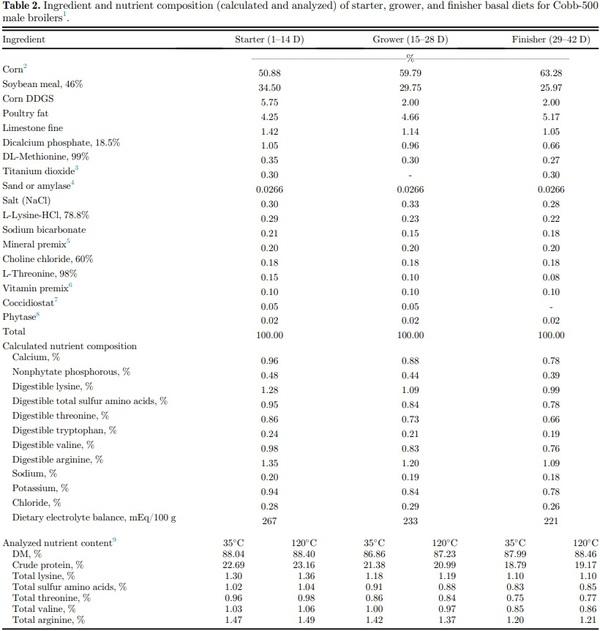
Abbreviation: DDGS, distillers dried grains with solubles. 1 Starter diet was used for both experiments conducted in parallel. 2 Corn was added and mixed in the same inclusion level for all dietary treatments according to its phase. All other ingredients were mixed in a basal diet devoid of corn. 3 Insoluble marker (Titanium dioxide, Venator, Hombitan AFDC101, CAS 13463-67-7, Duisburg, Germany). 4 Either sand or amylase (Ronozyme HiStarch CT, batch ID: AU360086), 133g ton-1 were included according to each dietary treatment to supply 80 kilo-Novo alpha amylase units (KNU). Depending on treatments, the inclusion of amylase and sand were 266 g ton-1 of sand; 133 g ton-1 sand 1133 g ton-1 amylase; and 266 g ton-1 of amylase. 5 Trace minerals provided per kg of premix: manganese (Mn SO4), 60 g; zinc (ZnSO4), 60 g; iron (FeSO4), 40 g; copper (CuSO4), 5 g; iodine (Ca(IO3)2), 1.25 g. 6 Vitamins provided per kg of premix: vitamin A, 13,227,513 IU; vitamin D3, 3,968,253 IU; vitamin E, 66,137 IU; vitamin B12, 39.6 mg; riboflavin, 13,227 mg; niacin, 110,229 mg; d-pantothenic acid, 22,045 mg; menadione, 3,968 mg; folic acid, 2,204 mg; vitamin B6, 7,936 mg; thiamine, 3,968 mg; biotin, 253.5 mg. 7 Coban 90 (Monensin), Elanco Animal Health, Greenfield, IN, at 500 g ton-1 in the starter and grower diets. 8 Ronozyme HiPhos GT, 200 g ton-1 to supply 1,000 FYT (Novozymes) delivering 0.14% of available P, and 0.10% of calcium. 9 Analyzed values in each column are means of 12 samples per feeding phase. 2 samples per each experimental diet were analyzed in duplicate. Evonik Industries, Evonik Degussa GmbH, Hanau-Wolfgang, Germany.
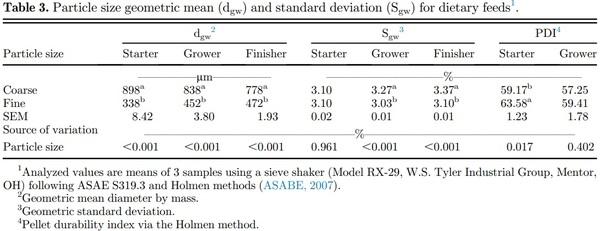
The inclusion levels for the enzymes used in the studies described herein were 200 g ton-1 phytase of Ronozyme HiPhos GT to supply 1,000 phytase units (FYT) and either 133 or 266 g ton-1 of alpha amylase Ronozyme HiStarch CT to supply 80 and 160 kilo-Novo alpha amylase units (KNU), respectively. Phytase enzyme was included in the basal diet and mixed with the rest of ingredients, whereas amylase was included depending on the specification for each dietary treatment. The supplemental enzymes used in the present experiment are commercially available (Novozymes A/S, Bagsvaerd, Denmark). For the digestibility evaluation, all experimental diets contained 0.3% titanium dioxide as an inert marker.
Particle Size Analysis and Pellet Durability Test
The distribution of particles was determined by ASAE S319.3 method (ASABE, 2007). A split 100-g sample was placed in a sieve shaker (Model RX-29; W.S. Tyler Industrial Group, Mentor, OH) for 10 min accompanied by 0.5 g of silicone dioxide dispersion agent and sieve agitators. Particle size results are presented in Table 3, and values are expressed as the particle size geometric mean (dgw). Results confirmed the targeted differences between coarse and fine diets. In addition, pellet samples from starter and grower diets were subjected to pellet durability testing via the Holmen method (Model NHP100; TekPro, North Walsham, United Kingdom) for 30 s (ASAE 2003b).
Experimental Procedure
Experiment 1 Chickens and feeders were weighed at 1, 14, 28, and 40 D of age to obtain BW and feed leftovers. BW gain, feed intake and FCR were calculated thereafter, and at 40 D, individual BW of broilers were obtained to determine flock uniformity (CV%). In addition, at 42 D, 2 chickens from each pen were selected and sampled. Proventriculus, gizzard, and liver were removed, and empty organs were weighed. Meanwhile, the length of the small intestine and ceca was measured. Results were expressed as either relative weight or length to their corresponding individual BW. Moreover, ileal digesta from these chickens were collected for subsequently nutrient digestibility analysis and energy utilization.
Experiment 2 Chicks and feeders were weighed to obtain BW and feed leftovers at 14 D, and BW gain, feed intake, and FCR were calculated. At 14 D, excreta samples were collected on waxed paper in 2 shifts, being immediately mixed and pooled by cage and stored in a freezer at −15°C. Ileal digesta contents were obtained from all chicks at 16 D after performing cervical dislocation, and digesta samples were collected from approximately 10 cm after the Meckel’s diverticulum to approximately 5 cm before the ileocecal junction. Ileal digesta contents were flushed with deionized water into plastic containers, pooled by cage, and immediately stored in a freezer at −15°C. Excreta and ileal digesta samples were lyophilized using FreeZone 6 (Labconco Corp., Kansas City, MO), and subsequently samples were ground to be able to pass through a 0.5-mm screen in a grinder.
Chemical Analysis and Calculations
Dietary treatments (12 Finisher feeds for Exp. 1, and 12 Starter feeds for Exp. 2), excreta (Exp. 2), and ileal digesta (Exp. 1 and 2) samples were analyzed for DM, CP, and titanium dioxide content. Fecal samples were further analyzed for gross energy (GE), and ileal digesta samples for starch. All dietary treatments (12 for finisher and starter respectively) were also analyzed for total starch. In addition, amylose content within the starch, in vitro starch gelatinization, and protein solubility analyses were performed in corn dried either at 35°C or 120°C. DM was determined according to method 934.01 of AOAC International (2006); CP (N x 6.25) was determined by combustion method (LECO, AOAC International, 2010; method 968.06). The GE analysis was conducted using an oxygen bomb calorimeter (IKA C5003; IKA Labortechnik), and AME was corrected to 0N retention (AMEn) using a factor of 8.22 kcal/g (Hill and Anderson, 1958). Total starch, amylose content, and in vitro starch gelatinization analyses were performed using the Megazyme Total Starch HK, K-AMYL, and K-SDAM assay procedures following manufacturer’s recommendations (Megazyme Inc., Chicago, IL). Protein solubility was determined by NIRS (DS2500; FOSS, Denmark). Amylase activity in pelleted feed was determined by the assay of alpha-amylase using red-starch S-RSTAR 03/06 (Megazyme, Bray, Wicklow, Ireland). Titanium dioxide concentrations were measured in triplicate for dietary feeds and duplicates for fecal and ileal digesta samples on a UV spectrophotometer following the method described by Myers et al., 2004. Apparent ileal digestibility, total tract utilization, and AMEn were calculated using the following equations (Kong and Adeola, 2014):
Digestibility (%) = [1 = (Mi / Mo) X (Eo / Ei)] X 100
AMEn (kcal / kg) = GEi = [GEo X Mi / Mo)] = 8.22 X {Ni = [No X Mi / Mo]}
where Mi represents the concentration of titanium dioxide for each dietary treatment in g/kg DM; Mo represents the concentration of titanium dioxide in the excreta and ileal digesta in g/kg DM output; Ei represents the concentration of DM, CP, energy, or starch on each dietary treatment in mg/kg of DM; and Eo represents the concentration of DM, CP, starch, and energy in the excreta and ileal digesta, in mg/kg DM. The GEi is GE (kcal/kg) for each dietary treatment; GEo is the GE (kcal/kg) in the excreta; Ni represents nitrogen concentration in the diet; and No represents nitrogen concentration in the excreta in g/kg DM. All analyses were conducted in 5 samples for feed and 3 samples for ileal and excreta samples.
Statistical Analysis
The experimental design was a randomized complete block design with a factorial arrangement of corn dried at 2 temperatures, grinded to obtain 2 particle sizes, and 3 amylase supplementation levels (2 x 2 x 3) for a total of 12 dietary treatments. In Exp. 1 and Exp. 2, each treatment had 8 replicates distributed equally either in 96 floor pens (Exp. 1) or 96 cages (Exp. 2). In addition, location of the floor pen inside the broiler house and location of cage in the batteries were considered blocks and random effects. Data for both experiments were analyzed separately and submitted to a three-way ANOVA in a mixed model using JMP 14 (SAS Institute. Inc., Cary, NC, 2016). Means were separated by the LS means procedure using Tukey’s or Student t tests at a significance level of alpha = 0.05.
RESULTS
The difference in corn humidity between kernels dried at 35°C and 120°C was 0.94% (P < 0.001) before grinding the corn to mix it in each experimental diet (Table 1). The average analyzed DM, CP, and total amino acid contents of diets based either on corn dried at 35°C or 120°C were similar (P > 0.10) within all 3 dietary phases (Table 2). The maximum difference between these diets was less than 0.5% for DM and CP and in general 0.02% points in total amino acids. These results indicated that initial differences in corn moisture after applying drying temperatures did not affect nutrient concentration of experimental diets. Enzyme recovery analysis showed that the contents were in agreement with the expected values for each inclusion level (Table 4). Enzyme concentration of Ronozyme HiStarch CT in the feeds was similar to the activity targeted.
Live Performance
Experiment 1 Results of growth performance are presented in Tables 5–7. At 14 D (Table 5), interaction effects (P < 0.05) were detected between drying temperature and particle size and drying temperature with amylase supplementation on BW gain and BW. Chickens fed FC-based diets and corn dried at 35°C gained up to 16 g more than broilers of other treatments, consequently, they were the heaviest group. Moreover, amylase supplementation improved BW gain and BW up to 10 g for chickens that consumed the diets containing corn dried at 35°C only. No interaction effects were observed (P > 0.05) for FCR at this age. However, feeding with FC-based diets improved the FCR (P < 0.01) by 3 points (1.239 vs. 1.267 g:g). On the other hand, FCR was not affected (P > 0.05) by either drying temperature or amylase supplementation at this age. At 28 D of age (Table 6), interaction effects were observed between drying temperature and particle size and between particle size with enzyme inclusion on BW gain (P < 0.01) and BW (P < 0.01). Broilers fed FC-based diets and corn dried at 35°C had the greatest BW gain and were the heaviest up to 46 g. In addition, feeding with CC-based and nonsupplemented diets resulted in lighter chickens. Interaction effects between particle size and amylase supplementation were observed on FCR (P < 0.01). At CC particle size level, the supplementation with amylase improved the FCR compared to chickens fed nonsupplemented diets. Moreover, broilers that consumed corn dried at 120°C had better (1.398 vs. 1.414 g:g) FCR than the ones that ate feeds containing corn dried at 35°C. Exploration of the data at 40 D of age (Table 7) showed no interaction effects (P > 0.05) among treatments. However, feeding with FC-based diets increased BWG (P < 0.05) and BW (P < 0.05) up to 37 g and enhanced (P < 0.01) FCR by w1 point. In addition, supplementing 266 g ton-1 of amylase improved (P < 0.05) FCR by 1.1 point compared to supplementing 133 g ton-1 or no supplementation.
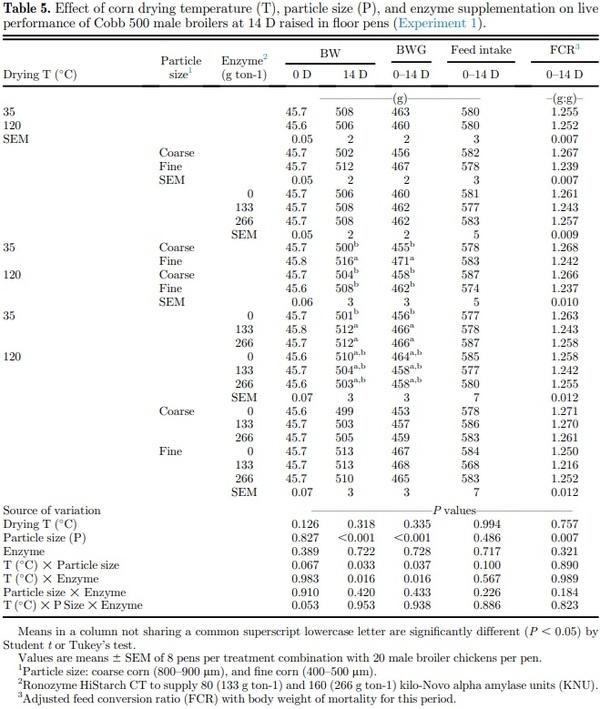
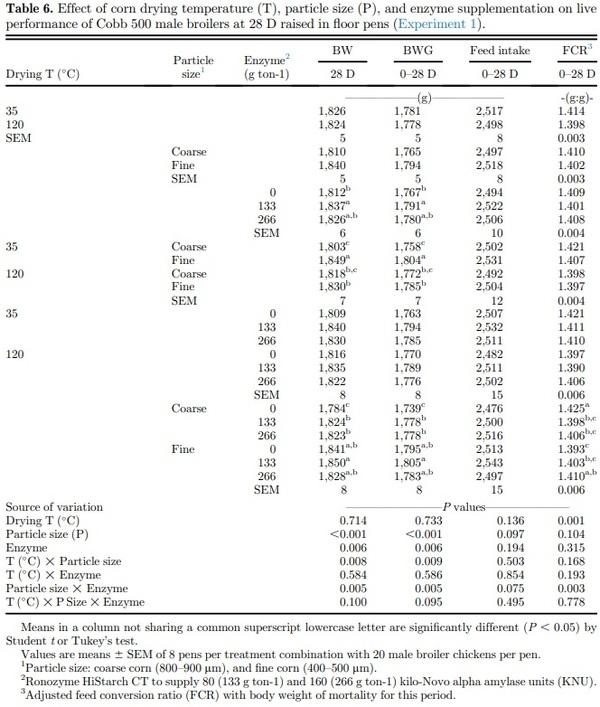
Experiment 2 Results of the study conducted in cages (Table 8) showed interaction effects between drying temperature and amylase supplementation on FCR (P < 0.01) and particle size and amylase supplementation on feed intake (P < 0.05). In feeds based on corn dried at 35°C, the supplementation with amylase improved FCR by 3 and 6 points for 133 and 266 g ton-1, respectively, when compared to chickens fed nonupplemented diets. Diets with CC amylase supplementation at 266 g ton-1 reduced feed intake compared to nonsupplemented diets and those with 133 g of amylase/ton. However, no differences on feed intake due to enzyme supplementation were observed on FC diets. Although no interaction effects (P > 0.05) were found on BW and BW gain, chickens that consumed FC-based diets gained more weight (P < 0.01), were heavier (P < 0.01), and had better FCR (P < 0.01) than chickens fed CC-based treatments.
Organ Development
Organ development results (Table 9) showed interaction effects between particle size and amylase supplementation for gizzard relative weight (P = 0.07) and ceca relative length (P < 0.001). Broilers fed with CC-based diets had greater gizzard development than chickens fed FC-based diets while comparing at the same amylase supplementation level. In addition, supplementing with 266 g ton-1 of exogenous amylase resulted in longer ceca for broilers that consumed FC-based diets, whereas an opposite response was observed for chickens fed CC-based diets. Furthermore, an interaction effect was detected between drying temperature and amylase supplementation on gizzard relative weight (P < 0.05). Broilers fed nonsupplemented diets and based on corn dried at 35°C had heavier gizzards than chickens fed diets with corn dried at the same temperature and supplemented with 266 g ton-1 of amylase. Likewise, these chickens had greater gizzard development than broilers fed diets containing corn dried at 120°C and no amylase supplementation or 133 g ton-1 of amylase supplementation. No interaction effects (P > 0.05) were detected for intestine relative length, nor relative weight of proventriculus, and liver. Curiously, supplementing with 266 g ton-1 of exogenous amylase resulted in longer (P < 0.01) intestines than in the nonsupplemented group. Moreover, particle size affected the relative weight of proventriculus (P < 0.01) and liver (P < 0.01). While feeding with FC-based diets, heavier proventriculus (0.27 vs. 0.25%) and lighter liver (2.29 vs. 2.40%) were found than when feeding CC-based diets.
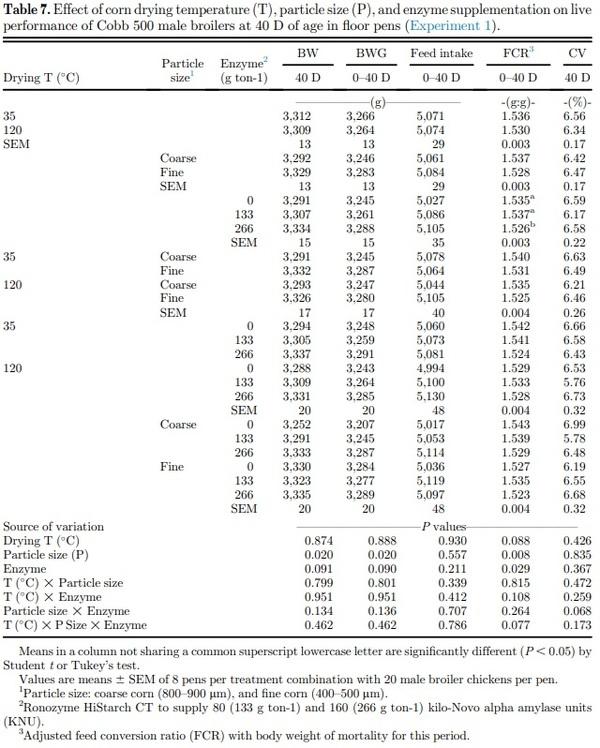
Nutrient Digestibility
Experiment 1 An interaction effect between particle size and amylase inclusion (P < 0.05) was detected for CP apparent ileal digestibility while feeding the finisher feed at 42 D of age (Table 10). Among broilers that consumed CC-based diets, the supplementation with 133 g ton-1 of amylase resulted in 16% greater digestibility than 0 or 266 g ton-1 inclusion of the enzyme. The addition of 133 g ton-1 of amylase increased (P < 0.05) starch digestibility by 2.26% compared to no amylase supplementation, whereas including 266 g ton-1 resulted in an intermediate response. In addition, feeding CC-based diets improved (P = 0.061) the starch digestibility by 1.42% compared to chickens that consumed FC-based diets.
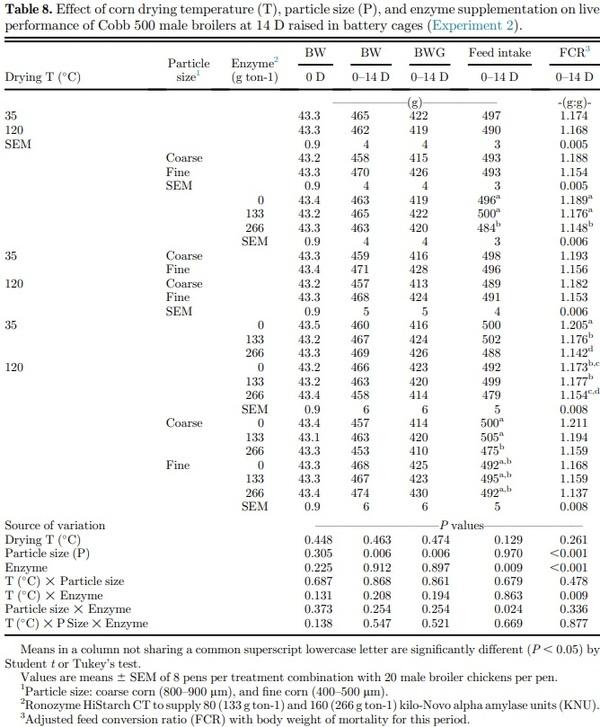
Experiment 2 For chicks raised in cages (Table 11), interaction effects were detected between drying temperature with amylase supplementation on CP fecal digestibility (P = 0.065) and starch ileal digestibility (P = 0.026). The interaction revealed that 266 g ton-1 of exogenous amylase increased the fecal digestibility of CP by 4.69% points in broilers fed corn dried at 120°C, compared to chickens that consumed nonsupplemented feed. In addition, differences for starch ileal digestibility were mainly driven by drying temperature. Drying the corn at 35°C showed greater starch digestibility than feeding diets with corn dried at 120°C, regardless of the level of dietary amylase supplementation. Moreover, the supplementation with amylase improved (P < 0.01) the AMEn by 5 and 4%, when including this enzyme in the diets at 133 and 266 g ton-1, respectively.
DISCUSSION
Corn dried at 35°C had higher moisture (14.45%) than the one dried at 120°C (11.20%), but after storage and before grinding and mixing, the moisture of both corn types only differed in 0.94% (Table 1) and nutrient content of final diets containing both corn kernels practically the same (Table 2). Maize grains release or absorb moisture to and from the environment during storage, and the storage stability is close to 12% (Angelovic et al., 2018).
In the present study, the interaction effects between drying temperature with particle size and enzyme supplementation observed at 14 D of age were not detected at 40 D, and this was consistent with nutrient digestibility for the study conducted in floor pens. Similarly, a recent experiment (Huart et al., 2018) found no effects on growth performance while feeding broilers with diets composed of corn dried at 54°C, 90°C, and 130°C up to 28 D. However, drying the corn at 130°C resulted in worst AID and fecal digestibility of starch. In contrast, Bhuiyan et al. (2010) concluded that offering feeds containing sun-dried corn to broilers increased the starch digestibility, whereas feeding with corn dried at 100°C resulted in poor FCR (> 1.6 g:g) at 21 D. Moreover, Barrier-Guillot et al. (1993) found that drying grains at different temperatures did not affect the digestibility of starch in broilers. In contrast, Kaczmarek et al. (2014) reported a 4% significant decrease in the AID of starch and CP in 35 day-old broilers because of consumption of corn dried at a high temperature (130°C–140°C). These results are in agreement with our findings at 16 D of age (Exp. 2) where starch digestibility was reduced by 2.6% because of drying corn at 120°C. It is possible that drying temperature is more relevant in younger chickens than in later growth phases, as their GIT is not mature (Krogdahl and Sell, 1989; Nir et al., 1993). In addition, the lower values of starch digestion observed when drying the corn at a high temperature could be partially explained by the higher amylose content (26.73 vs. 21.28%) and lower starch gelatinization (1.34 vs. 3.04%) compared to the corn dried at 35°C (Table 12). Previous studies reported the negative effects of these parameters on starch digestibility and further energy utilization (Malumba et al., 2008; Pirgozliev et al., 2010; Selle et al., 2010; Zhou et al., 2010).
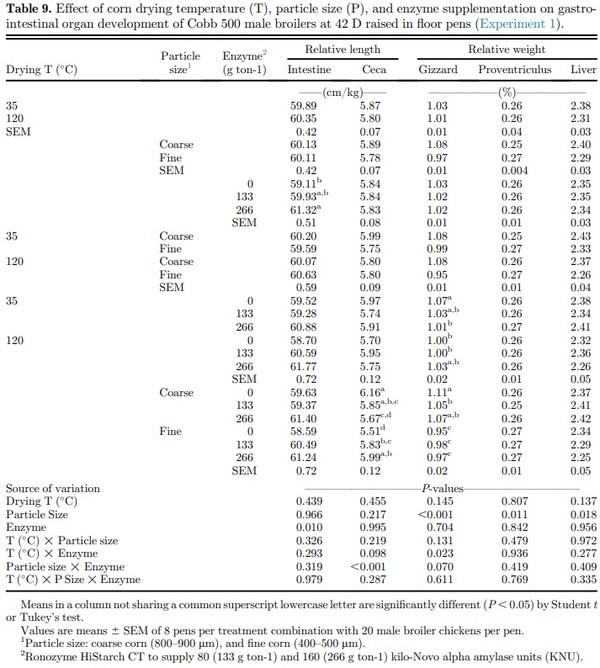
The improvements observed on live performance while feeding FC-based diets at 14 D were consistent in both studies presented here. Therefore, young broilers may not be able to efficiently consume or use larger corn particles (898 mm dgw) because of an underdeveloped grinding capacity of the gizzard (Lott et al., 1992; Kilburn and Edwards, 2001). Other studies have found similar results on live performance when feeding diets containing finely ground corn ground (Engberg et al., 2002; Taylor and Jones, 2004a). In contrast, other researchers (Hamilton and Proudfoot, 1995; Nir and Hillel, 1995; Svihus et al., 2004) reported that live performance is improved when feeding coarse corn, but a little finer than the one used in our studies, and the kernel hardness was not reported. In the present study, a hard corn kernel variety was used. The differences in feed form, pellet or crumble quality, average particle size and distribution, and the different ability of young broilers to use coarse particles could be confounding factors that may explain the inconsistent results in growth performance among studies. Moreover, the improvements on live performance due to offering FC-based diets may be attributed to a possible better pellet quality and durability as reported in previous studies (Muramatsu et al., 2013; Vukmirovic et al., 2017). As expected, in the present studies, the pellet durability index was higher (P < 0.01) in starter diets based on FC than in starter diets comprised of CC. However, this effect was not observed (P > 0.05) in the grower diets. The particle size geometric mean was bigger (P < 0.05) for corn dried at 120°C than for corn dried at 35°C, which worsened (P < 0.01) the pellet durability index in the starter feeds. These results may confirm previous hypothesis that corn may fragment different during grinding as influenced by drying temperature and the changes in starch and protein matrix in corn endosperm (Brown et al., 1979; Malumba et al., 2008; Kaczmarek et al., 2014).
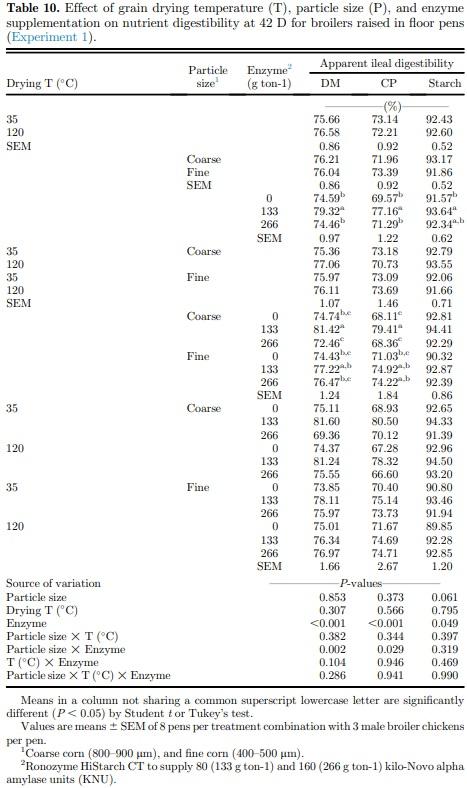
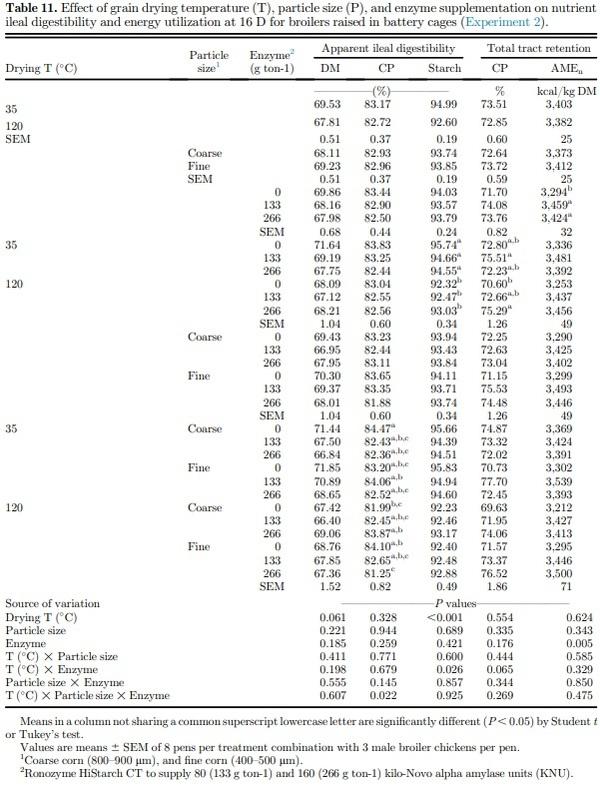
Organ development results showed that feeding FC resulted in enlarged proventriculus and lighter gizzard and liver. In agreement with our findings, previous studies observed an enlargement of the proventriculus and poor gizzard development (Amerah et al., 2008; Zaefarian et al., 2016) being attributed to feeding fine particles (< 600 μm, dgw). In contrast, a linear increase in gizzard weight has been detected with larger corn particles (Olver and Jonker, 1997; Dahlke et al. 2003; Zang et al., 2009; Pacheco et al., 2013, 2014). The gizzard has been reported to be the key gastric organ required to reduce coarse particles to smaller sizes for improved digestive efficiency, and a heavy, well-developed gizzard was involved in the regulation of gut motility (Duke et al., 1977; Ferket and Gernat, 2006; Xu et al., 2015). A well-developed gizzard also has been associated with a reduced digesta pH and passage rate (Nir et al., 1994), enhanced enzymatic digestion efficiency, and improved energy utilization and nutrient digestibility (Duke, 1992; Amerah et al., 2007). Indeed, in the present study, a heavier gizzard observed while feeding CC-based diets was followed by an improvement on starch digestibility at 40 D of age. A better gizzard development may have promoted retro-peristalsis function, which led to a greater starch digestibility. Although feeding FC improved live performance in the present studies, it could negatively impact overall gut health and nutrient utilization (Taylor and Jones; 2004b; Amerah et al., 2007; Yegani and Korver, 2008; Chewning et al., 2012) under stressful conditions. Despite the positive effect of feeding CC on gizzard development and starch digestibility, better live performance responses were observed by feeding FC throughout all the experimental phases and in both experiments in floor pens and cages.
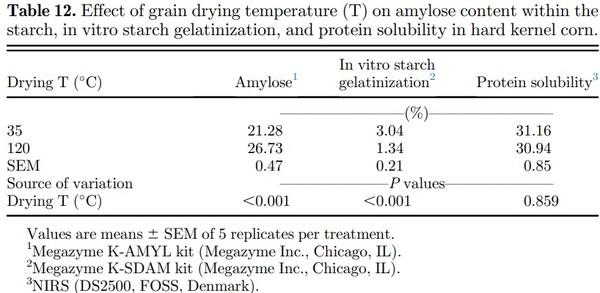
Amylase supplementation increased energy utilization and nutrient digestibility, which improved live performance throughout the experiment. Likewise, Gracia et al. (2003) observed significant increase in starch digestibility when α-amylase was supplemented to corn-soybean meal diets of broilers, indicating that endogenous α-amylase can be limiting for optimal starch digestion. Moreover, Kaczmarek et al. (2014) observed no beneficial effects of amylase supplementation on starch digestibility and growth performance as compared to the control (no enzyme) group. However, amylase improved AMEn by 100 kcal/kg as compared to a control diet without enzyme and comprised of corn that was ground finely (482 μm). There are several factors affecting starch digestion in poultry. Some of those factors are feed processing, particle size, starch content in the feed, presence of fibers, antinutritional factors, and non–feed-related factors such as bird’s genetics and age as explained by Zaefarian et al. (2015). Looking at starch digestion as a simple hydrolysis reaction, another key factor to add to the previous list would be the time required for complete hydrolysis. Feed intake level and particle size of the diet have shown considerable impact on feed retention time in the digestive tract and hence on the starch digestibility. Svihus (2011) clearly illustrated a declining total tract starch digestibility for increasing feed consumption of broiler chickens receiving wheat-based diets. This result was previously supported by Svihus (2006) where he observed decreased AME values (due to lower starch digestibility) for broilers overconsuming pelleted wheat-based diet. High levels of undigested starch in the excreta was detected by Péron et al. (2005) where they provided finely ground wheat diet to broiler chickens immediately after food deprivation, again relating reduced starch digestibility to feed overload and consequently fast transit of feed through the digestive tract. The mechanism by which DM, AMEn, and CP were enhanced by amylase supplementation could be an improved starch digestibility and the subsequent sparing effect on endogenous amino acids and energy associated with a reduction in endogenous amylase (Ritz et al., 1995; Gracia et al., 2003).
The results of these studies confirmed our hypothesis that broiler live performance, GIT organ development, energy utilization, and nutrient digestibility were influenced by interaction effects of grain drying temperature, particle size, and amylase supplementation. In conclusion, the data presented in these studies suggested that a high drying temperature depressed ileal starch digestibility especially in younger chickens. Drying temperature responses were mainly dependent on the interactions with particle size and amylase supplementation, and not by drying temperature itself. Feeding coarse corn did not improve live performance, but it had important contributions to GIT development, stimulating the functionality of the digestive organs (proventriculus, gizzard, intestines, liver), thus allowing for better starch digestibility. Amylase supplementation at 133 g ton-1 increased nutrient digestibility and energy utilization. The FCR was improved at the end of the study (40 D of age) when supplementing at 266 g ton1. Consequently, the usage of an exogenous amylase was effective on improving the live performance of broilers, but a higher dose was required for this corn hybrid with hard endosperm. In this way, a better usage of the starch and energy in this corn was achieved by including amylase in the diet.