DESCRIPTION OF PROBLEM
Phytase has been regularly incorporated into commercial poultry diets due to its relatively low cost and effectiveness at hydrolyzing phytate (IP6; myo-inositol 1, 2, 3, 4, 5, 6-hexakis dihydrogen phosphate; Bedford and Cowieson, 2009). Dephosphorylation through phytase supplementation is needed for birds to access this source of P and also to prevent the chelation of IP6 with other nutrients such as amino acids and minerals (Nelson, 1967; Ravindran et al., 1995; Cowieson et al., 2004). Phytase inclusion draws other advantages from the degradation of IP6 such as reduced feed costs (Kleyn, 2013; Ravindran, 2013), enhanced bone mineralization (Persia and Saylor, 2006), decreased nutrient excretion and subsequent environmental pollution (Correll, 1998; Cowieson et al., 2004), as well as improved bird performance (Sohail and Roland, 1999; Liu et al., 2008).
These benefits have promoted phytase as the most commonly utilized feed enzyme in the poultry industry (Ravindran, 2013). As a result, there are many commercially available phytases for poultry use. Although all share a common substrate under the classification of phytase, these enzymes vary in biochemical properties because of their differences in production and classification (Simon and Igbasan, 2002). Such variations include thermostability, proteolytic resistance, kinetics, dephosphorylation pathway, and optimal pH (Singh et al., 2011). Regardless of these differences, the use of a singular exogenous phytase product is typical among commercial formulations.
While IP5 through IP1 are all end products of phytase activity, the ultimate goal of exogenous phytase is to degrade IP6 into inositol (with the last phosphorus being taken off by endogenous phosphatases as phytases produce IP1 as the end product). Although the supplementation of phytase has been shown to reduce the presence of IP6 and generate substantial quantities of inositol, total conversion of IP6 to inositol has not yet been achieved (Zeller et al., 2015b; Li et al., 2017; Ingelmann et al., 2019). Therefore, the potential for new discoveries of strategies for enhanced phytase efficacy exist and should be evaluated through continued research. Literature examining the supplementation of more than one phytase is very limited, but previous research suggests the need for additional investigation into potential synergistic effects (Elkhalil et al., 2007; Zyla et al., 2012). The following paragraphs will describe in more detail the biochemical properties of pH and dephosphorylation that are likely most important for consideration when choosing PIS that may be synergistic.
Changes in pH occur throughout the digestion process and due to the effects of pH on IP6 susceptibility to hydrolysis, phytase enzymes differ in both their optimal pH and range in pH that they are active (Menezes-Blackburn et al., 2015; Zeller et al., 2015a). Therefore, the use of multiple phytase enzymes that differ in optimal pH may extend the exposure of phytate to phytase activity, leading to further phytate breakdown or complete degradation (Elkhalil et al., 2007). For the present study, phytase A and B, both classified as 6-phytases, were chosen due to their differences in optimal pH. Previous research has reported their optimal pH range to be between 3.5–5.0 and 3.0, respectively (Menezes-Blackburn et al., 2015).
If not hydrolyzed before entering the intestinal tract, some of the lower isomer intermediates of hydrolysis may continue to interfere with nutrient availability (i.e., chelation with certain nutrients), much like IP6 (Yu et al., 2014; Bedford et al., 2016). Enhanced disappearance of IP6 and these residual esters are associated with the occurrence of extraphosphoric benefits for broiler production (Gehring et al., 2013; Zeller et al., 2015a; Bedford et al., 2016); indicating that a more coordinated and efficacious approach toward phytate destruction would be desirable. Dephosphorylation pathway differences among phytase en-zymes can lead to variations in the presence of lower IP isomers (Greiner and Carlsson, 2006; Lim et al., 2007). Therefore, phytase C, classified as a 3-phytase, was included in the present study to primarily evaluate any synergy when combined with the two 6-phytases (A and B) for more effective IP6 degradation; in addition, this enzyme also had a slightly different optimal pH range of 4.5–5.5 reported by Menezes-Blackburn et al., 2015.
This study’s intent was to evaluate the synergistic effects between phytase enzymes vary-ing biochemical properties and verify a potential mode of action (if detected). The resulting effect would possibly offer increased nutrient availability and absorption beyond feeding the individual phytases alone, which would in turn potentially reduce excretion, lower diet costs, and promote broiler growth performance. Two different phytase activity levels were utilized, 120 and 240 FTU/kg; these low levels were chosen to hopefully prevent clouding mechanistic action with any extra phosphoric effects. Ultimately, the objective of this research was to evaluate the effects of feeding various phytase enzymes alone or in combination on male broiler growth performance, tibia characteristics, nutrient digestibility, and ileal IP esters.
MATERIALS AND METHODS
Enzymes
The three commercial phytase enzymes utilized in the present study were chosen based on their unique biochemical properties and their hydrolysis of IP6. All three phytase products were sent to a laboratory for phytase activity analysis before creating ground corn and enzyme premixes for a more precise dosing at the intended low activity levels for each treatment. A universal wet chemistry assay method (ESC SAM035-01 ISO FEFANA) was utilized for phytase activity determination for the enzyme premix blends and manufactured experimental diets; treatments including phytase A were confirmed with a modified assay for the specific enzyme protein (ABV, Elisa method. SAM099-07). Phytase A, an Escherichia coli derived 6-phytase expressed in Trichoderma reesei analyzed to contain an activity level of 7,030 FTU/kg (Quantum Blue 5G, AB Vista, Plantation, FL). Phytase B, a Buttiauxella spp. derived 6-phytase expressed through T. reesei, had an analyzed activity of 22,100 FTU/kg (AxtraPHY 20,000 TPT, DuPont Animal Nutrition. St. Louis, MO). Phytase C, a 3-phytase produced by Aspergillus niger had an analyzed activity of 6,830 FTU/kg (Natuphos 5000 G, BASF Corporation. Florham Park, NJ).
The three phytase enzymes were fed in granular form and one phytase unit (FTU) was defined as the amount of enzyme needed to release 1 μmol of inorganic P during 1 min at 37°C and a pH of 5.5. Enzymes were fed alone (A, B, or C) or in combinations (AB, AC, BC, or ABC) to meet total phytase activities of either 120 or 240 FTU/kg. It is important to note that when feeding the combinations, each enzyme provided half (AB, AC, or BC) or one-third (ABC) of the intended total activity. For example, to achieve the NC + AC at 120 FTU/kg treatment, inclusions of A and C were made to supply 60 FTU/kg of each to achieve a total of 120 FTU/kg.
Ground corn was chosen as a carrier for the enzyme and ground corn blends created to aid in the distribution of the small amount of each enzyme needed to achieve the proper activity levels of 120 and 240 FTU/kg when mixed in the basal diet. Using the batch size of 68 kg of feed and the analyzed phytase activity obtained from each of the 3 products, calculations were performed to determine the amount of each phytase needed to acquire the desired activity for each treatment. This calculated amount differed among treatments according to the differences in pure phytase product activities, the intended activity for finished treatment, as well as for the singular or combined inclusion of these enzymes. The weighed amount of phytase enzymes were then added to 0.907 kg of ground corn and mixed using a commercial stand mixer (Hobart, Legacy Floor Mixer) for 5 min. These blends were kept in appropriately labeled bags until they were introduced to the allocated amount of the NC basal diet for treatment creation. To account for the addition of the enzyme and corn blends to the basal diet, an equal amount of ground corn (0.907 kg for each of the 15 treatments requiring the NC basal and 0.907 kg for PC diet) was removed from the basal batches.
Diet Formulation
The PC and NC diets were corn and soybean meal–based and formulated to be 95% of breeder recommendations for AME, crude protein, and digestible amino acids (Aviagen, 2019; Table 1). Near-infrared spectroscopy (FOSS, Hillerød, Denmark) was utilized at Mississippi State University to scan the corn and soybean meal before formulation and batching to obtain available nutrient values. The only difference between the 2 basal diets was that the PC diet was formulated to contain 0.80% Ca and 0.40% nonphytate P (nPP), whereas the NC diet was formulated to supply 0.40% Ca and 0.20% nPP. These differences were achieved primarily through manipulation of defluorinated P and calcium carbonate. For nutrient digestibility determination, titanium dioxide (TiO2) was included as an indigestible marker in both basal diets at 0.3%.
Batching and Experimental Feed Manufacture
All basal diets (without enzyme inclusion) were batched at the Mississippi State University Poultry Research Unit feed mill and fed as unconditioned mash. Batching of basal diets began with the NC batches and ended with the PC to prevent cross contamination. Homogenous mixing was achieved using a 907 kg capacity vertical single screw mixer (Jacobson Machine Works, Minneapolis, MN) for 5 min with dry ingredients and an additional 10 min after the addition of soybean oil. After mixing, diets were bagged and weighed in aliquots of 22.68 kg per labeled bag. These bags were then sequentially allocated among pallets labeled for each treatment. This was carried out to ensure that treatments requiring the NC basal had equal proportions of each batch to minimize any variation among treatments.
After basal diet treatment allocation, corresponding corn and enzyme blends were introduced to the 68 kg treatment batch and mixed within a double-ribbon horizontal mixer (Wenger Manufacturing, Sabetha, KS; 136 kg capacity) for 5 min. Treatment mixing was performed in order of NC, NC + PIS (starting with singular enzyme inclusions for each com-mon enzyme blend, increasing from 120–240 FTU/kg and finishing with NC + ABC), and finally the PC diet. Between mixing treatments with different PIS, the mixer was cleaned out using paintbrushes and an air hose to remove residual feed-treatment dust. Representative mash samples were taken on emptying the mixer and were analyzed for nutrients, as well as phytase activity (Table 2). Results shown are the average of duplicate samples and the CV between the analyses was <15%. Mixed treatments were weighed in allotments of 6 kg and added to 10 small plastic buckets (10 buckets/ treatment). Each bucket was labeled with appropriate treatment and specific raised wire cage.
Broiler Management
This research was conducted using procedures compliant with the Mississippi State University Institutional Animal Care and Use Committee. Ross x Ross 708 chicks were obtained from a commercial hatchery (Peco Foods. Gordo, AL) on day of hatch and were feather sexed on arrival to the Mississippi State Poultry Research Unit (Starkville, MS). Males were weighed and distributed equally among 80 raised wire cages (12 chicks/cage; 61 cm x 46 cm) located inside one of 2 identical environmentally controlled rooms. In total, 160 raised wire cages of identical size were utilized for this research. These rooms were only separated by an entrance hallway and were enclosed within a common building; each room contained 5 complete blocks/replications (i.e., 10 total blocks/replications). Two nipple drinkers and one gravity-fed cage feeder supplied water and feed ad libitum. For the first 72 h, feed was placed inside a small plastic tray within each cage to ensure feed availability to chicks. Mortality and facility conditions were monitored daily.
Environmental control of the facility was achieved using 2 central heat and air units, as well as 2 small stir fans (36 cm) for ventilation. From placement to day 3 target room temperature was 32.2°C, with a reduction to 31.1°C on day 4. Target temperature decreased gradually per day, beginning on day 7 to end of study, reaching 27.8°C on day 14. Light bulbs (LED, 26.9 lux) provided illumination from the ceiling, as well as on top of the bottom rows of cages to ensure similar lighting throughout the room. All light bulbs followed the same intensity and dimming schedule. Full intensity was used for 24 h from day 0 to 7, then a 4-h dark period was introduced for the remaining experimental period. Lights were steadily dimmed starting on day 10 until reaching 2.69 lux on day 14 (trial ended at day 14).
Measured Variables
Live Performance. Birds were individually weighed on day 7 and 14. Unconsumed mash from cage feeders was emptied into respective cage/treatment buckets before weighing feed on day 7 and 14. Average BW, BW gain (BWG), feed intake per bird (FI), and percentage mortality were calculated from day 0 to 7, 7 to 14, and 0 to 14. Owing to percentage mortality not exceeding 5% for the entire study and no significant differences (P > 0.05) or trends (P > 0.10) during the trial period, mortality was not included in this article and will not be discussed further.
Tibia Ash and Mineralization Analysis. On day 14, three randomly selected birds per cage were euthanized via carbon dioxide asphyxiation for tibia extraction. The right tibia from each bird was carefully collected using scalpels, placed into appropriately labeled Whirl-Pak bags and then stored in a ˜4°C refrigerator for 12 h. Removal of meat, as well as the fibula bone from all tibiae, was performed on the following day. Cleaned bones were stored in a −20°C freezer until drying. A drying oven (105°C) was used to dry tibiae and crucibles for 12 h before crucible and dried tibia weights were recorded. Dried tibiae were wrapped in a labeled filter paper then subjected to Soxhlet fat extraction using ether for 12 h (Watson et al., 2006). Fat extracted tibias were then placed into a muffle furnace (600°C) for 24 h for ash-ing. Tibia ash was weighed and recorded after reaching room temperature (AOAC 932.16). After weighing, ash was sent to a laboratory for mineral content analysis (AOAC 968.08 MOD; Central Analytical Laboratory; Fayetteville, AR).
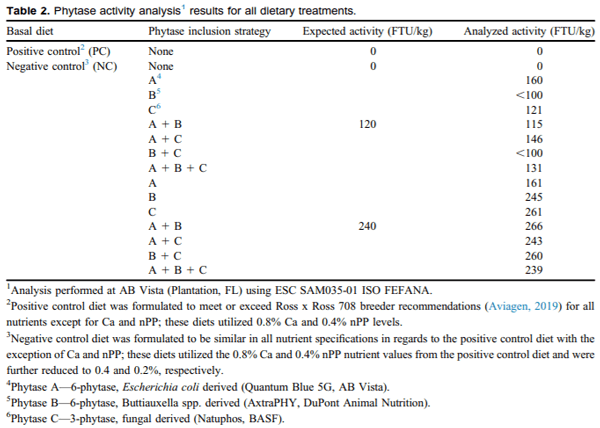
Apparent Ileal Digestibility and IP Ester Analysis. On day 14, ileal digesta was collected from all remaining birds/cage. Birds were euthanized using carbon dioxide asphyxiation before excising the ileum (Meckel’s diverticulum to ileocecal junction). This section was cut in half and the terminal portion was gently cleared of digesta using 5 mL syringes with reverse osmosis water. Digesta from the distal ileum was collected into labeled plastic specimen cups with lids and stored inside a −80°C freezer until freeze drying (Labconco, Kansas City, MO). Dried digesta samples remained inside storage cups with lids until ground using a coffee grinder. To ensure an adequate sample quantity for analyses, 2 dried digesta samples from adjacent blocks of the same treatment were pooled to obtain 5 replications/treatment. Feed samples and a portion of ground ileal digesta were sent to a commercial laboratory (ATC Scientific, North Little Rock, AR; ANAB accredited with AAFCO Proficiency Testing Program) for Ca, P, Lys, Met, Thr, Ile, Val, and TiO2 analysis (AOAC procedures). The resulting analyzed values were utilized to determine ratios of TiO2 and each nutrient within each dietary treatment plus collected digesta to estimate apparent ileal digestibility coefficients (AIDC) through the equation as described by Ravindran et al. (2005): AIDC = 1- [(TiO2diet/TiO2digesta) 3 (Nutrientdiet/ Nutrientdigesta)].
The remaining ground ileal digesta samples were sent to a laboratory (University of East Anglia. Norwich, England) to determine the presence of inositol, inositol triphosphate (IP3), inositol tetraphosphate (IP4), inositol pentaphosphate (IP5), and inositol hexaphosphate (IP6) using high-performance ion chromatography methodology (Madsen and Brinch-Pedersen, 2019; Pirgozliev et al., 2019).
Statistical Analysis
The 16 treatments were arranged within the experimental climate-controlled housing as a randomized complete block design; there were 10 complete replicates/blocks (designated by location; 5 complete blocks per identical experimental room). The experimental unit was one raised wire cage (61 cm x 46 cm) of 12 Ross x Ross 708 male chicks and the experimental period was from 0 to 14 d. The experimental unit for tibia ash mineralization data consisted of the mean obtained from 3 randomly selected tibias from each cage, with 10 replications/treatment. For digestibility and IP ester analysis, the experimental unit consisted of one pooled sample (2 cages of the same treatment from blocks within closest proximity to one another) of freeze dried digesta which was later ground, mixed, split, and sent to separate laboratories, creating 5 replications for each treatment. Samples were pooled to obtain an appropriate quantity needed for analyses. All data were analyzed using the GLM procedure in SAS 2016 (Statistical Analysis Software, Version 9.4, SAS Institute Inc., Cary, NC). The P value was set at ≤ 0.05 and Fisher’s least significant difference was used to investigate significant differences between treatment means. Resulting P values > 0.05 and ≤ 0.10 were identified as trends and will be discussed.
RESULTS AND DISCUSSION
Broiler Performance
In the interest of space and clarity for this 16 treatment study, the authors have chosen to highlight the results demonstrating the possibility of synergism among PIS treatments; general statements will be made in regard to the observations among birds fed PC and NC diets vs. NC + PIS (NC + PIS will be used to identify all enzyme treatments: A, B, C or their combinations fed at either 120 or 240 FTU/kg). In addition, the practice of increasing PIS from 120 to 240 FTU/kg for each similar enzyme treatment will be discussed (i.e., NC + A at 120 FTU/kg and NC + A at 240 FTU/kg).
There were significant differences for day 7 BW (P = 0.049; Table 3), but the PC and NC fed birds had similar BW, despite the differences in Ca and nPP. The reduction in Ca and nPP of the NC diet may have led to better utilization of scarce availability of these minerals; this could have contributed to a lesser difference in nPP between the PC and NC diets, causing a lack of performance differences (Letourneau-Montminy et al., 2008). However, it is also possible that the use of NIR nutrient values for corn and soybean meal when formulating contributed to this discrepancy, as the use of as wet chemistry (and digestible P, rather than nPP) would have been better to ensure intended formulation differences between PC and NC diets (David et al., 2019). In addition, the analyzed Ca were higher than intended and the consistent 2:1 Ca:nPP ratio between the diets may have affected the current data. It should be noted that previous research has observed that feeding low Ca diets enhanced phytase activity, which subsequently muted the effects of providing a diet with low P availability (Akter et al., 2016). As explored in more detail later, lack of PC and NC performance differences does not discount the present study, as phytase research has also established that tibia ash data are often more sensitive to Ca:nPP reductions, rather than live performance (Shastak et al., 2012).
For both 0–7 d BWG and FCR, feeding NC + ABC at 120 FTU/kg tended to reduce BWG and increase FCR in comparison with those fed the NC and PC, resulting in the worst performance for those metrics (Table 3; P = 0.053 and 0.064, respectively); increasing the same PIS to 240 FTU/kg brought bird performance to intermediate. For 0–7 d BWG, birds fed NC + AB at 120 FTU/kg tended to have the highest gain, with all other treatments falling in between, including the NC and PC birds. For 0–7 d FCR, birds fed PC diets tended to have the best FCR, which was a 14-point improvement from that observed with feeding ABC at 120 FTU/kg. In addition, the use of NC + BC, NC + AC, and NC + AB at 240 FTU/kg resulted in FCR numerically improved from the intermediate NC to a similar level of PC.
Significant differences were observed for day 7 BW (Table 3; P = 0.049), in which birds fed NC + AB at 120 FTU/kg had the highest BW, which was also similar to the PC; birds fed NC + ABC at 120 FTU/kg had the lowest BW, which was similar to the NC. However, for this metric, birds fed PC and NC diets resulted in similar day 7 BW, which was intermediate compared with the high BW of birds fed NC + AB at 120 FTU/kg and the low BW of birds fed NC + ABC at 120 FTU/kg. Falling in between the NC and PC fed birds, similar intermediate BW was demonstrated with feeding B and AC at 120 FTU/kg as well as BC, AB, AC, and ABC at 240 FTU/kg. In addition, it is important to note that when examining the performance of birds fed the same PIS, but different phytase activities, there were no differences (i.e., NC + A at 120 FTU/kg vs. 240 FTU/kg; Table 3). Ultimately, birds fed NC + AC, C, B at 240 FTU/kg, as well as NC + B and BC at 120 FTU/kg had similar day 7 BW to birds fed PC and NC + AB at 120 FTU/kg. This was in contrast to our anticipation of improved bird performance with increased activity levels, as previously demonstrated due to better IP6 degradation (Bedford et al., 2016). However, early aged broilers have also been reported to elicit more BW and BWG differences in response to phytase, which may be an explanation for day 7 BW being affected with these low activity levels (Li et al., 2018). Because feeding NC only resulted in small differences for day 7 BW between those fed 120 and 240 FTU/kg of PIS, it is speculated that the addition of phytase was not necessary to restore the BW of NC fed birds up to that of PC fed birds (our formulation parameters for the NC diet likely were not low enough). In addition, it was not unexpected for a lack of performance differences to occur between the 2 PIS activities used in the present study because neither would be considered high enough to achieve the benefits of extraphosphoric effects.
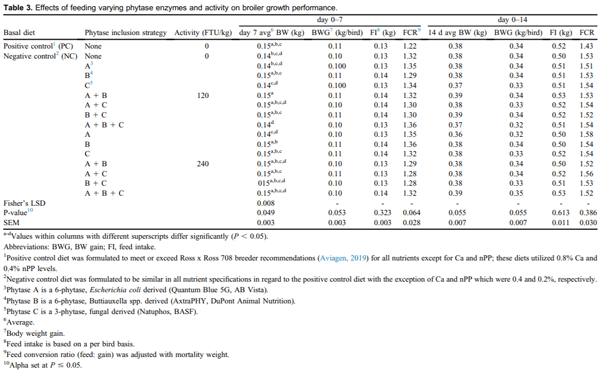
For day 0–14 performance, there were no significant differences for FI/bird, nor FCR (P = 0.613 and 0.386, respectively; Table 3); however, strong trends were found for day 14 BW and 0–14 d BWG (both P = 0.055). For these metrics, birds fed NC + ABC at 240 FTU/kg had the highest BW and BWG and birds fed NC + A at 240 FTU/kg had the lowest; all other treatments fell in between, including the NC and PC fed birds which were similar to either end of the spectrum. Although not as dramatic, benefits have also been found with the inclusion of phytase at low activity levels (100–250 FTU/kg) within reduced P diets for monogastric species (Shirley and Edwards, 2003; Kies et al., 2006; Pirgozliev and Bedford, 2013; Walters et al., 2019). The results of the present study indicate that feeding an increased activity (i.e., 240 FTU/kg) of NC + ABC tended to result in heavier birds on day 14. To create this PIS, each of the 3 phytases in combination were included at 80 FTU/kg, yet this combination numerically improved bird performance.
We had anticipated that feeding AB, AC, BC, or ABC at 120 FTU/kg would improve performance above that demonstrated when feeding 120 FTU/kg of A, B, or C. However, for day 7 BW, the only combination which increased performance compared with singular addition was NC + AB at 120 FTU/kg compared with birds fed NC + A at 120 FTU/kg and 240 FTU/kg. The plausible reasons for the lack of improved performance for the combination enzyme treatments include 1) the enzymes are not synergistic and 2) the activity level for each individual phytase in the 3 enzyme combination (40 FTU/ kg each) was too low to effectively hydrolyze phytate for a noticeable growth response. As previously mentioned, very low phytase activities were utilized for the present study to more clearly investigate potential synergism between multiple phytases on the destruction of phytate; the thought was that low activities would not cloud results through extraphosphoric effects associated with higher phytase activity levels. However, these activities are much lower than typically included in broiler diets and bird response through performance metrics may not have been distinguishable.
All treatments, with the exception of NC + B at 120 FTU/kg, NC + BC at 120 FTU/kg, and NC + A at 240 FTU/kg indicate intended analyzed activity ranges were reasonably achieved for the present study (Table 2). It should be noted that discrepancies in the assay may be contributing to the effects with combined phytase inclusion. For instance, use of a universal phytase activity method and potential for poor distribution of the miniscule enzyme amount in the sample may have influenced in vivo bird response (Loop et al., 2012). More accurate phytase activities may have been achieved through the use of product specific assays; however, this was not feasible given resources, as well as the present study’s intentions of providing multiple phytases to equal the total activity and the minimum dose needed for assay determination.
Apparent Ileal Digestibility Coefficients
In general, AIDC was consistently high with feeding PC, NC + B, and NC + BC at 240 FTU/kg. In addition, birds fed PC had the highest AIDC for both Met and Thr, followed by NC + C at 120 FTU/kg and NC + B at 240 FTU/kg, respectively (P < 0.05; Table 4). Compared with PC, feeding NC resulted in reduced Met AIDC, whereas similar AIDC was found for Thr.
Consistently low AIDCs were observed across all AAs for birds fed NC + AC and NC + C at 240 FTU/kg and NC + ABC at 120 FTU/kg, even compared with the NC (P < 0.0001). Interestingly, NC + C at 120 FTU/kg had consistently high AIDC, similar or close to AIDC demonstrated by birds fed PC (except for Thr). Results pertaining to the specific effects of PIS on AIDC will be provided in the following paragraphs of this section. At times only PIS will be discussed, but some treatment comparisons vs. NC will be made to explore any PIS benefit beyond the AIDC of birds fed NC diets.
Feeding NC + BC at 240 FTU/kg resulted in the highest AIDC for P, Ile, and Val, followed immediately (with similar AIDC) by birds fed NC + B at 240 FTU/kg (P < 0.0001; Table 4). In addition, Ca and Lys AIDC were highest with feeding NC + B at 240 FTU/kg, followed similarly by birds fed NC + BC at 240 FTU/kg (both P < 0.0001; Table 4). For Ca, P, Lys, and Val, birds fed NC + AC at 240 FTU/kg had the lowest AIDC (all P < 0.0001; Table 4). In comparison, birds fed NC had improved AIDC for the same nutrients. For Met, AIDC was the lowest for birds fed NC + C at 240 FTU/kg (P 0.0001; Table 4). Birds fed NC + ABC at 120 FTU/kg had the lowest AIDC for Thr and Ile, followed immediately (with similar AIDC) by birds fed NC + AC and NC + C at 240 FTU/kg. In comparison with feeding NC + ABC at 120 FTU/kg, feeding NC resulted in improved, although intermediate AIDC for Met, Thr, and Ile. The authors speculate that the addition of phytase C to these PIS resulted in interference with the A and B phytase enzymes when utilized in combination.
When specifically comparing the same PIS at 120 vs. 240 FTU/kg, differences were observed and varied in accordance with PIS and nutrient measured. In general, enhanced AIDC was observed with feeding NC + C, NC + AB, and NC + AC at 120 FTU/kg compared with 240 FTU/kg for the same PIS (P < 0.0001; Table 4). Improvements in AIDC were noted depending on the nutrient measured for each of the following PIS at an activity of 120 FTU/kg vs. the same PIS at an activity of 240 FTU/kg: NC + A AIDC for Ca; NC + AB AIDC for Ca, Met, Ile, and Val; NC + AC AIDC for Ca, P, Lys, Met, Thr, and Val; and NC + C AIDC for Ca, P, Lys, Met, Thr, Ile, and Val (P < 0.0001; Table 4).
Alternatively, other PIS resulted in improved AIDC when supplemented at an increased activity level (i.e., 240 FTU/kg vs. 120 FTU/kg). In general, AIDC was improved when feeding 240 FTU/kg of NC + B, NC + BC, and NC + ABC compared with the same PIS at 120 FTU/kg (P < 0.0001; Table 4). Specific improvements in AIDC were noted for each of the following PIS included at 240 FTU/kg vs. the same PIS included at 120 FTU/kg; NC + B for Ca, P, Lys, Met, Thr, and Val; NC + BC for P, Lys, Thr, Ile, and Val; and NC + ABC for Lys, Thr, Ile, and Val (P < 0.0001; Table 4).
The lower AIDC found within the PIS of NC + C and NC + AC at 240 FTU/kg, as well as NC + ABC at 120 FTU/kg vs. their activity counterparts may indicate activity was not effective enough to cleave the amino acids from phytate complexes (Rutherfurd et al., 2004). Alternatively, including C with other phytases combinations (NC + AC at 240 FTU/kg or NC + ABC at 120 FTU/kg) may have lowered the efficacy of amino acid cleavage by the other phytases. This may be due to the differences in phytases, as phytase C is a 3-phytase and may perhaps be the cause for the mineral AIDC data as well. However, Zyla et al (2012) demonstrated the positive effects for Ca retention from including a 6-phytase with a nonspecific acid phosphomonoesterase, which is supposed to remove phosphate groups with less preference.
Ileal IP Esters
No significant differences were observed for the variable IP3 (P = 0.3239; Table 4), which is supported by previous research (Beeson et al., 2017). However, significant differences were observed for IP6, IP5, IP4, and inositol concentrations (P < 0.0001; Table 4). For both IP6 and IP5, feeding PC resulted in the highest concentration. This finding was expected due to the lack of exogenous phytase and the formulation goal of increased dietary Ca and nPP; which likely interfered with endogenous phytase hydrolysis (Zeller et al., 2015a).
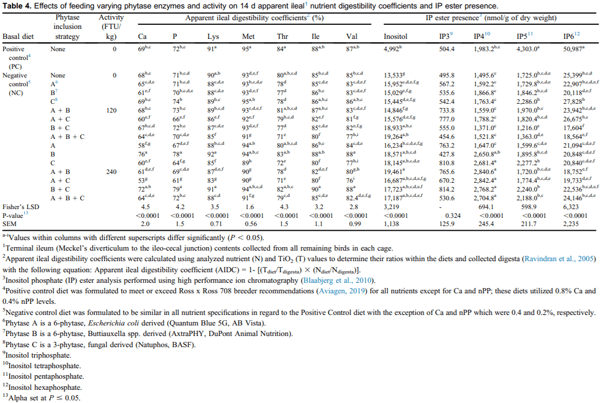
Results pertaining to the specific effects of PIS on ileal IP ester concentrations will be described in the following. At times only PIS will be discussed, but some comparisons with NC will be made to demonstrate if there was any benefit of PIS beyond ileal IP ester proportions of birds fed NC diets. Feeding NC + BC at 120 FTU/kg resulted in the lowest concentration for both IP6 and IP5. When compared with feeding NC, birds fed NC + BC at 120 FTU/kg had reduced IP6 and numerically reduced IP5 concentrations. For IP4, birds fed NC + AC at 240 FTU/kg had the highest concentration, followed by similar, but numerically increased amounts of IP4 by NC + AB, NC + BC, NC + ABC, and NC + C all at 240 FTU/kg (P < 0.0001; Table 4). Birds fed NC + BC at 120 FTU/kg had the lowest ileal IP4 concentration, immediately followed with a similar, but numerically increased concentration by NC and the following PIS: NC + ABC at 120 FTU/kg; NC + AB at 120 FTU/kg, NC + A at 120 FTU/kg, NC + A at 240 FTU/ kg, NC + C at 120 FTU/kg, NC + AC at 120 FTU/kg, NC + B at 120 FTU/kg. Feeding PC resulted in an intermediate concentration of IP4. In general, treatments were polarized in their response regarding IP4 concentrations, with the only intermediate concentrations demonstrated with PC and NC + B at 240 FTU/kg; whereas IP4 concentrations generally increased with increasing phytase dose for all treatments (exception for NC + A at 240 FTU/kg). This effect was expected because the production of IP4 is greater than its hydrolysis when lower doses of phytase are used; IP4 reduction is often only seen when phytase is superdosed (Walk et al., 2018). These data confirm that feeding broilers the higher dose of 240 FTU/kg pro-vided more phytase to the intestinal tract.
When specifically comparing the same PIS at 120 vs. 240 FTU/kg, increased IP6 concentrations were observed with feeding 120 FTU/kg of NC + AC and NC + C. As for IP5, increased concentrations were observed with feeding 240 FTU/kg of NC + ABC and BC vs. the same PIS at 120 FTU/kg. For IP4, increased concentrations were observed with feeding 240 FTU/kg (vs. 120 FTU/kg) of the following PIS: NC + AB, NC + ABC, NC + AC, NC + B, NC + BC, and NC + C. These data suggest that higher phytase dose for many of the combined PIS results in a coordinative action (rather than antagonistic) among specific phytases and their resulting dephosphorylation process of IP6 and IP5 to IP4.
It is likely that unique mechanisms of dephosphorylation associated with each phytase carry more significance toward effective cleavage of lower esters when used at lower activity levels. Bello et al. (2019) found reduction in ileal digesta IP4 with a Citrobacter braakii phytase vs. a Buttiauxella sp. phytase (similar to phytase B), both supplemented at 500 FTU/kg; however, both performed similarly, when included at higher activity of 1,000 FTU/kg. Previous research by Walk and Olukosi (2019) reported that any hindrance in the stepwise cleavage of lower esters due to the biochemical properties associated with individual phytase enzymes may be overcome with the use of higher inclusions, such as 2,000 or 4,000 FTU/ kg. Therefore, it is possible the extremely low amounts of phytase used in the present study contributed to the lack of results observed for IP3 and IP4 concentration.
For the metric inositol, feeding NC + AB at 240 FTU/kg resulted in the highest ileal concentrations followed by birds fed NC + ABC at 120 FTU/kg (P < 0.0001; Table 4). Feeding PC resulted in the lowest inositol concentration and although increased in comparison, birds fed NC had the next lowest inositol concentration (P < 0.0001; Table 4); all remaining PIS were numerically, if not statistically, higher. It was anticipated that the NC and PC would have low concentrations of ileal inositol as a result of exogenous phytase absence. Although PC and NC diets did not include exogenous phytase, it is not unusual to detect inositol within the ileum due to endogenous sources of phytase or intrinsic plant phytases (Shastak et al., 2014; Rodehutscord and Rosenfelder, 2016). This intestinal phytase is especially activated when low calcium and phosphorus diets are fed, which is reflected in the reduced IP6 and elevated inositol levels noted on the NC diet compared with the PC in this study. When specifically comparing the use of 120 vs. 240 FTU/kg, increased inositol concentrations were observed with feeding NC + B and NC + AB at 240 FTU/kg vs. their 120 FTU/kg counterparts. It was not unexpected for the lower activity levels of 120 FTU/kg PIS to be less effective at decreasing IP6 into its lower esters as previous research has shown increased efficacy with higher dosages (Li et al., 2017).
In general, less effective IP6 and lower ester degradation was obtained when feeding the following PIS: NC + A, NC + B, NC + C, NC + AB at 120 FTU/kg and NC + ABC at 240 FTU/kg; this was verified by the resulting high concentrations of IP6 and IP5 paired with lower concentrations of IP4 and inositol from the aforementioned treatments. However, improved degradation effects (less IP6, IP5, and more IP4, IP3, inositol) were demonstrated consistently by birds fed NC + BC and NC + ABC at 120 FTU/kg, with a similar response observed in birds fed NC + AC at 240 FTU/kg. Based on previous research findings of Bello et al. (2019), this would suggest that the use of phytase combinations when included at a low dosage can perform similarly to that of higher dosages. Because phytase A and C were of different classifications, it is presumed that the characteristics for stepwise dephosphorylation contributed to more efficacious approach to IP6 cleavage.
Tibia Ash and Mineral Concentration of Ash
Increased tibia ash percentage was observed with feeding birds the PC diet in comparison with all other treatments (P < 0.0001; Table 5). Although not similar to the PC, the next highest tibia ash percentage was demonstrated when feeding NC + ABC at 240 FTU/kg. For the same metric, feeding NC + ABC at 120 FTU/ kg resulted in the lowest tibia ash percentage, although it was similar to birds fed NC. Improvements in tibia ash percentage between feeding PIS at 120 or 240 FTU/kg were only found with birds fed the aforementioned treatments of NC + ABC at 240 vs. 120 FTU/kg. As eluded to earlier, tibia ash percentage differences were observed between the PC and NC, with increased tibia ash percentage when feeding PC vs. NC. Feeding NC + AC, NC + BC, NC + A, NC + B, and NC + AB at 120 FTU/kg and NC + ABC, NC + AB, NC + BC, NC + B, and NC + C at 240 FTU/ kg resulted in a tibia ash percentage significantly reduced from PC; and all were similar to NC + A + B + C at 120 FTU/kg, which had the lowest tibia ash percentage, except for birds fed NC + AC and NC + BC at 120 FTU/kg and NC + AB, NC + BC, and NC + ABC at 240 FTU/kg.
When comparing the specific minerals found within tibia ash samples, differences were observed for Mn concentration (P = 0.0477; Table 5). For tibia Mn, feeding NC + B at 240 FTU/kg resulted in the highest concentration followed by birds fed NC + BC at 240 FTU/kg and NC + AC, NC + C, NC + AB at 120 FTU/ kg; whereas birds fed NC + A at 240 FTU/kg had the lowest Mn concentration, which was similar to the PC and NC. For this metric, feeding the PC and NC diets resulted in similar Mn concentrations. In addition, when comparing the same PIS at 120 vs. 240 FTU/kg, tibia Mn concentration was enhanced with the activity of 240 FTU/kg for NC + B and NC + BC. This trace mineral has been reported to chelate readily with phytic acid (Mohanna and Nys, 1999). Increasing the proportion of Mn in tibias is an indication that phytase was able to release Mn from its insoluble complex with phytate. This result would be a desirable effect due to the involvement of this particular trace mineral with broiler leg health and immune response (Lu et al., 2007; Ghosh et al., 2016).
There were no other significant differences for tibia mineral concentration (Ca, P, S, K, Na, Zn, Al, and Fe; data not shown, P-values ranging from 0.3540 to 0.8946); however, strong trends were found for Cu and Mg and will be discussed in the following paragraph (P = 0.0514 and 0.0671, respectively; Table 5). First, the authors would like to comment on the lack of significance for Ca and P given that these 2 particular minerals influence bone health and can also interfere with phytase efficacy. Given the importance of these 2 minerals, the lack of differences between birds fed NC and PIS treatments in the current study was unexpected. However, this may be explained by the low phytase activities utilized, as previous phytase research has also reported increased tibia ash without an effect on Ca and P tibia content with addition of phytase at 500 FTU/kg (Viveros et al., 2002; Pintar et al., 2005)
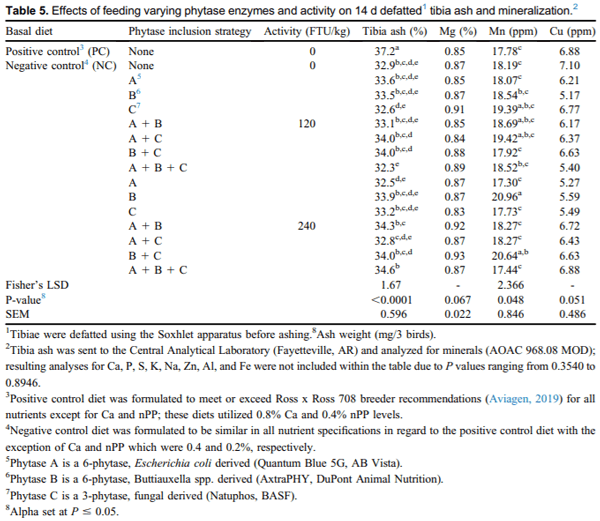
As previously mentioned, some noteworthy trends were demonstrated for tibia Cu and Mg content and will be further discussed within this paragraph. For Cu, birds fed NC tended to have the highest Cu concentration, whereas birds fed NC + B at 120 FTU/kg tended to have the lowest; and all other PIS, as well as the PC, fell in between. By contrast, Singh et al (2013) found decreased tibia Cu when feeding broilers a diet low in Ca (0.75% in starter). For Mg, birds fed NC + BC at 240 FTU/kg tended to have the highest concentration, whereas birds fed NC + C at 240 FTU/kg tended to have the lowest; and all other PIS, as well as the PC and NC tended to have intermediate Mg concentrations.
Phytic acid readily chelates with cationic minerals such as Zn, Mn, and Fe, which can be found organically within feedstuffs or included into the diet (Akter et al., 2015); this provides an explanation for the increased presence of Mn and Mg within tibias of birds fed all PIS. Increasing the availability of organic trace minerals, such as Mn, Cu, Mg, and Fe can enhance leg health (Sirri et al., 2016), decrease mineral excretion (Nollet et al., 2008), and contribute to antioxidant properties (Midorikawa et al., 2001). Once again, although these diets did include a vitamin mineral pre-mix, there were no additional sources of these minerals except for those present in the feed-stuffs; therefore, it is unexpected that the NC fed birds, without PIS, tended to have the highest Cu mineral concentration. It is speculated by the authors that this effect resulted from the low presence of IP4 ileal concentrations observed with feeding NC. This lower ester has been reported to impact the digestibility of minerals from its chelating ability (Beeson et al., 2014; Bedford et al., 2016). Our results for tibia ash metrics demonstrated more sensitivity toward PIS than growth performance, which is in agreement with previous research (Farhadi et al., 2017). As such, determination of bone ash is regarded to be a key indicator in the evaluation of phytase’s ability to enhance the bioavailability of minerals via destruction of phytate–mineral complexes (Hall et al., 2003).
Overall
Consistency for specific PIS treatments across growth performance, AIDC, ileal IP ester concentrations, and tibia ash and mineral concentration of ash was not clearly achieved; however, some treatments did demonstrate synergistic potential for several metrics. These data demonstrate that tibia ash and mineralization, AIDC, and IP ester presence were more sensitive to PIS programs, with performance being minimally affected. At this time, specific recommendations cannot be made toward a particular PIS, but these data indicate that there may be potential benefits with multiple phytase inclusion at low dietary activity levels as carried out in this trial. Some of the PIS demonstrating synergy were found with feeding: NC + BC at 120 FTU/kg and NC + AB at 240 FTU/kg in that these treatments demonstrated increased IP6 degradation, high AIDC for Ca, P, Lys, Met, Thr, Val, and Ile, as well as similar day 7 and day 14 BW/BWG to that of birds fed PC. It is important to consider the multitude of factors that affect bird response to phytase including dose, dietary Ca and P content, bird age, and the use of cages vs. floor (Bedford et al., 2016). The present study was designed as an initial investigation into the use of multiple phytase enzymes on a small scale. Results from this experiment indicate a need for further investigation on a larger scale. Therefore, future research into combined phytase strategies is warranted and should be conducted using higher phytase doses, alternative Ca:P ratios, longer experimental period, as well as the use of floor pens to evaluate if these PIS may be applicable to the commercial poultry industry.
CONCLUSIONS AND APPLICATIONS
- Supplementation of phytase(s) into the NC diet which had reduced Ca and nPP (0.4 and 0.2%, respectively) resulted in decreased IP6 and IP5 ileal concentrations compared with feeding birds PC diets (0.8% Ca and 0.4% nPP; no exogenous phytase). It is likely that the higher proportion of nutrients for PC, specifically Ca, interfered with endogenous intestinal phytase efficacy to dephosphorylate IP6 effectively.
- The present study suggests some benefit toward multiple phytase inclusion and synergistic potential; thus, warranting future research. With the future in mind, research should evaluate higher phytase activities, different dietary Ca and nPP levels, and a longer grow out within a floor-pen facility.
- Feeding NC + BC at 120 FTU/kg and NC + AB at 240 FTU/kg resulted in similar day 7 BW to PC, which was improved beyond birds given NC + C at 120 FTU/kg and NC + A at 240 FTU/kg. In addition, feeding NC + BC at 120 FTU/kg and NC + AB at 240 FTU/kg tended to result in similar day 14 BW and day 0–14 BWG to that of birds fed PC. These particular enzymes had reduced ileal concentrations of IP6 and IP5, as well as increased IP. In addition, these treatments had consistently high AIDC for Ca, P, Lys, Met, Thr, Val, and Ile. Collectively, these data suggest that the addition of B may improve efficacy of A or C, even at a lower dose than when included singularly (i.e., AB at 240 FTU/kg contained 120 FTU/kg of A and of B).
ACKNOWLEDGMENTS
The authors would like to express gratitude toward the U.S Poultry and Egg Association for the financial support of this project (#F082). In addition, the authors would like to recognize AB Vista for sourcing the enzymes and providing their assistance with funding for the phytase activity, IP ester, and tibia ash mineral content analyses. This project was also successful due to the tremendous assistance of the Mississippi State Poultry Research Unit agricultural technicians, as well as staff, and fellow graduate students within the Mississippi State University Poultry Science Department; specifically Donna Morgan, Andrew Brown, Carley Rhoads, Jonathan Moon, and Farley Fondren. Use of trade names in this publication is not an indication of endorsement by Mississippi State University. This project was funded by U.S Poultry and Egg Association (project #F082). In addition, the authors would like to recognize AB Vista for sourcing the enzymes and providing their assistance with funding for the phytase activity, IP ester, and tibia ash mineral content analyses.
DISCLOSURES
Some of the coauthors employed by AB Vista helped to source enzymes and provided funding assistance for phytase activity, IP ester analysis, and tibia ash mineral content analysis.
This article was originally published in 2020 Journal of Applied Poultry Research 29:977–994. https://doi.org/10.1016/j.japr.2020.09.006. This is an Open Access article under the CC BY-NC-ND license (http://creativecommons.org/licenses/by-nc-nd/4.0/).