INTRODUCTION
The importance of nutritional management for improving production and minimizing environmental impacts from beef and dairy cattle industry is well-established (McAllister et al., 1996; Havlík et al., 2014; Bach et al., 2020). Corn grain is the most commonly used cereal grain for dairy and beef production in the United States and its high starch content yields energy to support greater levels of milk and meat production (Giuberti et al., 2014). However, improving productivity in this manner may hinder cow health as high-starch diets can lead to disorders like rumen acidosis (Owens et al., 1998). Furthermore, high corn prices directly affect feed costs and can reduce farm profitability (Ferraretto et al., 2011). Thus, using well-formulated high-energy corn-based diets can guarantee rumen health while optimizing feed cost-effectiveness.
Chase (2007) reported that the dietary starch concentration for lactating dairy cows in the U.S. ranges from 20% to 30%, while in feedlots, finishing diets may contain up to 62% of starch (Zinn et al., 2007). Total tract starch digestibility in ruminant diets ranges from 70% to 100% (Firkins et al., 2001) while rumen digestibility as a percentage of starch intake ranges from 47% to 90% (Huntington, 1997; Firkins et al., 2001). Greater ruminal starch digestibility is associated with greater microbial protein synthesis (Firkins et al., 1998) and fast fermenting starchy substrates can increase microbial protein in the rumen when compared to slowly fermenting ones (Cone and Becker, 2012). Therefore, improving rate and extent of starch digestion in the rumen may provide nutritional advantages through increased volatile fatty acid (VFA) and microbial protein synthesis on this site.
Various feed (processing method, grain particle size, maturity at harvest, corn silage chop length) and animal-related factors (intake levels, physiological state of animals, production levels) can influence corn starch digestibility by ruminants (Firkins et al., 2001). In addition, extensive crosslinking of zein proteins yielding vitreous endosperm lower corn starch in vitro and in situ digestibility (Philippeau and Michalet-Doreau, 1997; Correa et al., 2002; Ngonyamo-Majee et al., 2008). Studies have shown that corn grain varieties with greater vitreousness lowered in vivo starch digestibility in lactating dairy cows (Allen et al., 2008; Lopes et al., 2009).
Supplementing exogenous enzymes have potential to improve starch digestibility by removing structural barriers and increasing accessibility of microbial α-amylases to starch granules in corn grain. Exogenous enzymes have been explored as alternatives associated with physical processing of corn in dairy and beef diets. Several studies have reported positive effects of α-amylase supplementation on dry matter intake (DMI), ruminal and total tract starch digestibility, feed efficiency, milk and meat production (Tricarico et al., 2005; Harrison and Tricarico, 2007; Klingerman et al., 2009; Meschiatti et al., 2019; Toseti et al., 2020), although inconsistencies across trials have been observed (Ferraretto et al., 2011; Weiss et al. 2011; DiLorenzo et al., 2011). Similarly, exogenous proteases or blends of enzymes containing proteases have been evaluated as feed additives in total mixed ration (TMR) of both dairy and beef cows; however, studies have reported greater (Eun and Beauchemin, 2005; Gado et al., 2009) or no effect (Vera et al., 2012; Meschiatti et al., 2019) on animal performance when compared with control. Exogenous proteases have also been used as additives in high-moisture and whole-plant corn silage and results showed greater corn starch digestibility when compared to control due to the breakdown of the protein matrix surrounding the starch granules (Young et al., 2012; Kung Jr et al., 2014; Ferraretto et al., 2015a; Der Bedrosian and Kung Jr, 2019). Despite these studies, there is limited literature available on the effects of the direct addition of endo-acting α-amylases and proteases on mature corn grain on in vitro dry matter and starch digestibility. Furthermore, to the best of our knowledge, we are lacking studies with exo-acting glucoamylases, which are important considering that the rumen microbiome have 5,000 putative endo-acting amylases while only about 20 putative exo-acting amylases (Stewart et al., 2018). Thus, in the current experiment our focus was to screen the endo-acting α-amylases, exo-acting glucoamylases, and proteases under ruminal conditions (temperature, pH) using corn grain as substrate, in search of enzymatic additives that are substrate-specific, effective, and efficient in improving rumen digestibility of feedstuffs (Meale et al., 2014). The objective of this study was to evaluate the effects of applying incremental doses of several exogenous α-amylases, glucoamylases, and proteases on ruminal in vitro dry matter digestibility (IVDMD), in vitro starch digestibility (IVSD), gas production, and VFA production from mature dent corn grain in a batch culture system. We hypothesized dose-dependent improvement in IVDMD and IVSD with the inclusion of exogenous enzymes to the dent corn grain.
MATERIALS AND METHODS
All animal handling and rumen fluid sampling procedures were performed according to a protocol approved by the University of Florida Animal Care Research Committee; protocol number 202009849.
Substrate
Mature dent corn grain was used as a substrate for these experiments. Intact corn kernels were dried at 60 °C for 48 h in a forced-air oven and ground to pass through a 4-mm screen in a Wiley mill (A.H. Thomas Scientific, Philadelphia, PA). Sample for nutrient characterization was collected, ground to pass through a 1-mm screen and analyzed for dry matter (DM), starch, CP, neutral detergent fiber (aNDF), and acid detergent fiber (ADF) at a commercial laboratory (Dairy One Laboratory, Ithaca, New York).
Enzymes and Enzyme Activity
Ten exogenous enzymes with amylolytic activity; α-amylases, glucoamylases, an α-glucosidase, and three exogenous proteases (DuPont Nutrition and Biosciences, Wilmington, DE) were evaluated in this study. Exogenous enzymes were identified as both endo-acting α-amylases or exo-acting glucoamylases; 1LAT (bacterial α-amylase), 2AK, 3AC, 4Cs4, 5Trga, 6Afuga, 7Fvga, and 10Tg (fungal α-amylases, glucoamylases, and α-glucosidase), 8Star and 9Syn (fungal amylase-mixtures; experiment 1), and exogenous proteases; 11P14L, 12P7L, and 13P30L (bacterial proteases; experiment 2).
Enzyme type, source, and protein concentration are presented in Table 1.
Endo-acting α-amylase and exo-acting glucoamylase activities (Table 2) were determined for all enzymes screened in experiment 1 in triplicate using potato starch as substrate under simulated ruminal conditions (pH 6.0 and 40 °C) as recommended by Colombatto and Beauchemin (2003). Enzymes were serially diluted in 0.1 M citrate– phosphate buffer solution at pH 6.0 and final activity was reported as µmol of glucose released per mL of enzyme per min of incubation (µmol/mL/ min). α-Amylase activity was measured using the 3,5-dinitrosalicylic acid (DNS) method (Wood and Bhat, 1988). Briefly, potato starch and enzymes were incubated for 5 min at 40 °C, stained with DNS reagent, and assay samples were read at 540 nm using a spectrophotometer (Jasco V-530, Jasco, Tokyo, Japan). Absorbance was corrected for blanks, substrate and enzyme, and release of reducing sugars were expressed as µmol/mL/min. Glucoamylase activity was measured using a glucose oxidase kit (Teco Diagnostics, CA, USA; Catalog# G520). Briefly, potato starch and enzymes were incubated in an oven at 40 °C for 90 min and the reaction was stopped by adding glucose oxidase reagent. Samples were read at 500 nm using a temperature-controlled microplate reader (Spectra Max 340PC, Molecular Devices Corporation, Sunnyvale, CA, USA).
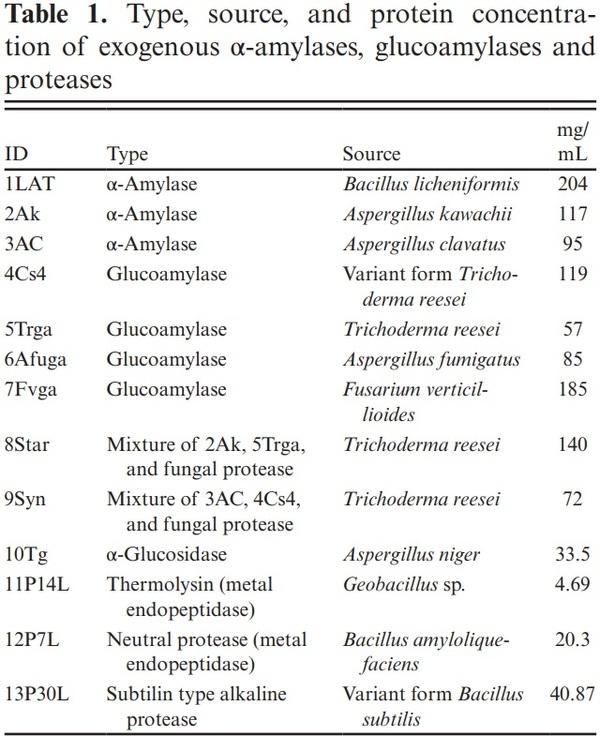
Absorbance was corrected for blanks, substrate and enzyme, and release of glucose was converted to µmol/mL/min.
Protease activity (Table 2) was defined by units/ mg of enzyme protein for all enzymes in experiment 2. For protease 11P14L, 1 unit (U) was defined as the quantity of enzyme that produces 200 µg of tyrosine-equivalent trichloroacetic acid-soluble peptides per min when using casein substrate under pH 6.5 and 35 °C. Protease 12P7L activity was determined by the hydrolysis of azo-casein at pH 7.5 and 30 °C. Protease 13P30L activity was determined based on the hydrolysis of N-succinyl-AlaAla-Ala-p-nitroanilide at pH 8.6 and 30 °C and release of p-nitroanilide.
Pilot Study
A pilot study was conducted to select the range of optimal doses for enzymes to be used for experiments 1 and 2. Three α-amylases were randomly selected (1LAT, 2AK, and 3AC) and based on protein concentration, appropriate dilutions were prepared in 0.1 M citrate–phosphate buffer solution (pH 6.0) to achieve 0.25, 0.50, 0.75, 1.00, 1.25, and 1.50 mg of enzyme/g of the substrate on a DM basis. The enzyme dilutions were prepared no earlier than a day before incubation and kept refrigerated at 4 °C until application. Dried ground dent corn (4-mm, 0.50 g) was weighed into Ankom F57 filter bags (Ankom Technology, Macedon, NY). Before sample weighing, empty F57 filter bags were prerinsed in acetone for 5 min and then air-dried per manufacturer instructions. Enzymes were applied directly to the substrate. Bags were sealed with a Uline Tabletop Poly Bag Sealer (Impulse type AIE200, American International Electronics, Kenneth City, FL) and immediately placed into 160 mL serum vials. No enzymes were applied to the control bags. Blank bags with no substrate were incubated to account for bag weight variation and gas production. IVDMD and gas production were examined using six replicates for each dose in a 12 h in vitro batch culture incubation in each of three runs.
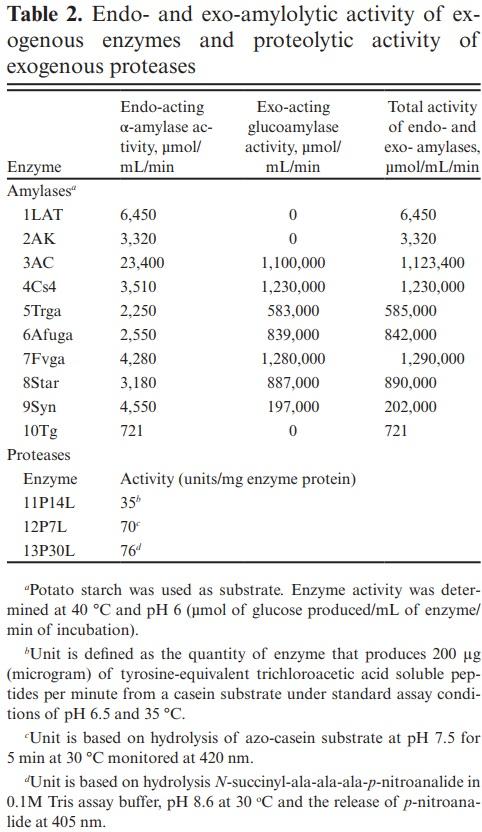
In vitro batch culture. Rumen fluid was collected from three lactating, ruminally cannulated Holstein dairy cows approximately 2–3 h after the morning feeding. Donor cows were fed a TMR containing (DM basis) corn silage (38.2%), alfalfa hay (4%), ground corn (27.3%), soybean meal 44% (14.5%), citrus pulp (9.2%), mineral mix and clay (5%), and rumen-inert fat (1.8%). Diets were offered twice every day (06:00 a.m. and 14:00 p.m.).
Rumen fluid was manually collected, filtered through two-layer cheesecloth, and pooled into prewarmed thermos flasks kept at 39 °C. Thermos flasks containing pooled rumen fluid were kept airtight until transported to the laboratory for final filtration with two more layers of cheesecloth (total of four layers). Rumen fluid inoculum was then added to a buffered pre-warmed (39 °C) media (Goering and Van Soest, 1970) in a 1:2 ratio (rumen fluid:artificial saliva). The media was continuously infused with CO2 to maintain the anaerobic environment for the rumen fluid inoculum.
Buffered rumen fluid (52 mL) was added to the 160-mL serum vials containing Ankom bags, and a continuous stream of CO2 was flushed into the vials during the whole inoculation process. Vials were closed with rubber stoppers and crimped with aluminum seals. Vials were immediately placed in an air-forced incubator at 39 °C without a shaking system for 12 h for the pilot study.
Gas pressure. Gas pressure was measured at 3, 6, 9, and 12 h after incubation using a pressure transducer and subsequently converted to gas volumes after correcting for gas volumes from blank bottles. Based on the conditions in the laboratory, the following equation was used to convert pressure to volume:
Gas vol. (mL)=[Gas pressure ( psi) ∗ 4.8843] + 3.1296
In vitro dry matter digestibility. Dry matter digestibility was calculated after 12 h of incubation. The incubations were terminated by placing bottles on ice. Bags were taken out of serum bottles, washed with tap water until effluent was clear, dried in a forced-air oven set at 60 °C for 48 h. Dried residues were weighed, and their DM concentrations were used to estimate IVDMD.
Effects of exogenous α-amylases, glucoamylases and α-glucosidase (experiment 1) and proteases (experiment 2) on in vitro rumen fermentation parameters. The effects of 10 exogenous enzymes with amylolytic activity; α-amylases, glucoamylases, α-glucosidase, and some combinations (1LAT, 2AK, 3AC, 4Cs4, 5Trga, 6Afuga, 7Fvga, 8Star, 9Syn, and 10Tg; experiment 1) and three exogenous proteases (11P14L, 12P7L and 13P30L; experiment 2) added at 0, 0.25, 0.50, 0.75, and 1.0 mg/g substrate DM were examined on IVDMD, IVSD, gas production, and rumen fermentation parameters. Enzyme dilutions were prepared in 0.1 M citrate– phosphate buffer solution (pH 6.0) to achieve 0.25, 0.50, 0.75, and 1.0 mg/g of substrate on a DM basis. Substrate (0.5 g ground dent corn) was weighed into Ankom F57 filter bags (Ankom Technology, Macedon, NY) and enzymes were directly applied to the substrate and immediately incubated for 7 h (Richards et al., 1995) at 39 °C with buffered-rumen fluid following procedure as described for the pilot study. Six replicates per treatment (experiment 1) and four replicates per treatment (experiment 2) were used for each run and three runs were conducted for both studies.
Gas pressure. Gas pressure was measured at 7 h after incubation, for both experiments 1 and 2, as described in the pilot study.
In vitro dry matter digestibility. Dry matter digestibility was calculated after 7 h of incubation for experiments 1 and 2 as previously described.
In vitro starch digestibility. Starch digestibility was estimated in experiments 1 and 2. After 7 h of incubation, two replicate bags were opened, and the residues were pooled to provide enough sample for starch analysis. Samples were shipped to a commercial laboratory (Dairy One Laboratory, Ithaca, NY). Starch analysis was conducted on samples after pre-extracting for sugar by incubating in 40 ºC water bath followed by filtration on Whatman filter paper no. 41. Residues were solubilized using an autoclave followed by incubation with glucoamylase enzyme to hydrolyze starch to produce dextrose (glucose). Prepared samples were injected into a sample chamber of YSI Analyzer (YSI 2700 SELECT Biochemistry Analyzer, Yellow Springs, OH; www.ysilifescience.com) where dextrose diffuses into a membrane containing glucose oxidase. The dextrose was immediately oxidized to hydrogen peroxide and d-glucono-4-lactone. The hydrogen peroxide was detected amperometrically at the platinum electrode surface. The current flow at the electrode was directly proportional to the hydrogen peroxide concentration, and hence to the dextrose concentration. Starch was determined by multiplying dextrose by 0.9 (method adapted from Dairy One laboratory, Ithaca, NY).
pH and volatile fatty acids. The pH of the rumen inoculum postincubation was measured using a pH meter (Accumet XL25 pH meter, Fisher Scientific Inc., Waltham, MA). Filtrate from two replicate vials (20 mL each) was pooled into 50 mL centrifuge tubes. The 40 mL pooled sample was acidified with 0.4 mL of 50% H2SO4, centrifuged at 7,000 × g for 15 min at 4 °C. The supernatant was filtered with a 0.22 μm filter and frozen (−20 °C) until analyzed for VFA concentration (Muck and Dickerson, 1988) using a high-performance liquid chromatograph (HPLC) system (Merck Hitachi Elite LaChrome, Hitachi, L2400, Tokyo, Japan) and a Bio-Rad Aminex HPX-87H ion exclusion column (300 × 7.8 mm i.d.; Bio-Rad Laboratories, Hercules, CA). Briefly, the column was used in an isocratic elution system containing 0.015 M sulfuric acid in the mobile phase of HPLC with a UV detector (wavelength 210 nm; L-2400, Hitachi) and a flow rate of 0.7 mL/min at 46 °C.
Statistical Analysis
For the pilot study, data for each enzyme (1LAT, 2AK, and 3AC) were analyzed separately using the GLIMMIX procedure of SAS (version 9.1; SAS Institute, Cary, NC) as a completely randomized block design using run as a blocking factor. Dose of each enzyme was used as a fixed effect and run as a random effect in the model and IVDMD was the response variable. Linear, quadratic, and cubic orthogonal contrasts were tested using the CONTRAST statement in SAS. For gas production data, main effect and interaction of dose (0, 0.25, 0.50, 0.75, 1.0, 1.25, and 1.50 mg/g substrate DM) and sampling hours (3, 6, 9, and 12 h) were tested in the model as fixed effects. Sampling hours were considered a repeated factor in GLIMMIX procedure of SAS. All means for IVDMD and gas production are presented as LSMEANS. Significance was declared at P ≤0.05 while tendency was declared at 0.05 < P ≤ 0.10.
For experiments 1 and 2, data for each enzyme were analyzed separately using GLIMIIMX procedure of SAS (version 9.1; SAS Institute, Cary, NC). Both experiments were designed as randomized complete block design using run as a blocking factor. Dose was used as fixed effect while run was considered a random effect for all variables, which included IVDMD, IVSD, gas production, filtrate pH, and VFA profile. Linear, quadratic, and cubic orthogonal contrasts were tested using the CONTRAST statement in SAS. The UNIVARIATE procedure of SAS was used to test the residuals for outliers and normality before the final analyses were performed. Treatment effects were declared significant at P ≤ 0.05 while any trends were defined at 0.05 ≤ P ≤ 0.10.
RESULTS AND DISCUSSION
Exogenous enzymes have been studied in both dairy and beef cattle diets for improving nutrient digestibility. While fibrolytic enzymes have received considerable attention in the past decades for improving ruminal fiber degradation and improving efficiency of livestock production (Beauchemin et al., 1995), exogenous amylases and proteases have not been extensively studied in ruminants (Beauchemin and Holtshausen, 2010). Regardless of the type of exogenous enzymes, the effects on improving nutrient digestibility and production performance have been inconsistent due to a variety of factors such as enzyme activity, inclusion level, method of supplementation, diet, and animal production level (Meale et al., 2014). While screening enzymes in vivo is an ideal approach, this method is time-consuming and expensive. Consequently, in vitro batch culture evaluation systems are preferred for screening the most effective enzymes in order to be considered for inclusion in the dairy and beef cattle diets. In the present study, we screened 10 α-amylases, glucoamylases, α-glucosidase, and three proteases based on their effects on IVDMD, IVSD, and ruminal fermentation parameters. All amylolytic enzymes evaluated in this study showed endo-acting amylase activity; however, no exo-acting glucoamylase activity was observed after 90 min of incubation for enzymes 1LAT, 2AK, and 10Tg (Table 2).
Ground dent corn was used as substrate in this study with following chemical composition: DM, 88.8%; starch, 67.7%; total digestible nutrients (TDN) 89.0%; nonfibrous carbohydrates, 79.3%; CP, 8.40%; aNDF, 6.70%, and ADF, 3%. In the pilot study (Table 3), enzyme 1LAT increased IVDMD by 22.7% compared to control when applied at the lowest dose (0.25 mg/g), but no greater improvements were observed with higher dose applications. Enzyme 2AK increased DMD by 21.6% compared to control when applied at 1.0 mg/g substrate DM; however, no effects were observed with an application rate of 0.25, 0.50, and 0.75 mg/g substrate DM, compared to the control. Enzyme 3AC increased IVDMD by 6, 23, 31, 39, 32, and 31% at 0.25, 0.50, 0.75, 1, 1.25, and 1.50 mg/g, respectively. Based on the results from this pilot study, we observed that the optimal range of enzyme application to improve IVDMD was between 0.25 and 1.0 mg/g substrate DM. Gas volume was increased (Figure S1) with enzyme 1LAT application for all the time-points evaluated in the pilot study with greater accumulation observed at 12 h for doses greater than 0.50 mg/g of substrate. Gas production was increased with 2AK (Figure S2) after 3 h postincubation and greater effects were observed at dose 0.25 mg/g. Enzyme 3AC increased gas volume after 6 h postincubation and greater effects were observed with dose 1.0 mg/g (Figure S3). Based on the results from the pilot study, we selected four doses; 0.25, 0.50, 0.75, and 1.0 mg/g substrate DM for experiments 1 and 2.
Exogenous enzymes, α-amylases, glucoamylases, and an α-glucosidase, were evaluated in experiment 1 and except for 2AK, 3AC, and 10Tg, all enzymes increased IVDMD, compared with control (Table 4). Enzymes 1LAT, 5Trga, and 8Star increased IVDMD at the lowest dose (0.25 mg/g substrate DM) by 25.3%, 48.8%, and 62.3%, respectively, compared with control. Enzymes 4Cs4, 6Afuga, 7Fvga, and 9Syn increased IVDMD in a dose-dependent manner, with greatest IVDMD observed when 1 mg of enzyme was applied. The improvement in IVDMD with exogenous glucoamylase is probably attributed to improvement in IVSD, mainly because the endosperm comprises the majority of the kernel DM and approximately 86% of the endosperm is composed of starch (Eckhoff and Paulsen, 1996; Ferraretto et al., 2018). Enzymes 1LAT, 5Trga, and 8Star increased IVSD by 35%, 40.9%, and 58.3% at the lowest dose of enzyme application (0.25 mg/g substrate DM); however, no further improvements were observed with higher doses of application. Enzymes 4Cs4, 6Afuga, 7Fvga, and 9Syn increased IVSD in a dose-dependent manner. Enzyme 4Cs4 increased IVSD by 51.8% compared to control when applied at 0.50 mg/g but no further improvements were observed at higher doses of application. For enzymes 6Afuga, 7Fvga, and 9Syn greatest improvements were observed with 1.0 mg/g substrate DM dose as IVSD was increased by 85.9%, 37.8%, and 65%, respectively, compared to the control. Enzymes 2AK, 3AC, and 10Tg did not induce any improvements in IVSD. The lack of effects observed with 2AK and 10Tg can be attributed to lower exo- and endo-glucanase activity (Table 2). The results observed on IVDMD and IVSD with other enzymes are in agreement with Rojo-Rubio et al. (2001) and Mora-Jaimes et al. (2002) who tested the effects of an exogenous α-amylase on ruminal starch digestibility of sorghum using in vitro and in vivo systems, respectively. Ruminal IVSD was increased by 55.7% when compared with control after 12 h of incubation in vitro (Rojo-Rubio et al., 2001) while in vivo ruminal starch digestibility was 16% greater (Mora-Jaimes et al., 2002), compared with control. While an increase in starch digestibility was observed with both in vitro and in vivo experimental models, the magnitude of improvement was lower in vivo possibly because of the rapid degradation of exogenous enzymes by rumen proteases (Chesson, 1994; Hristov et al., 1998). The efficacy of 5Trga and 6Afuga was further validated using in vivo study in beef bulls as both DM and starch digestibility was improved with enzyme supplementation (Devant et al., 2020). Nevertheless, we should be cautious interpreting this data since results were not confirmed using multiple corn hybrids and future research is warranted to validate these findings using multiple corn varieties.
While rumen stability of the exogenous amylases was not measured in this study, we speculate that most of the enzymes tested in this study were relatively stable under ruminal conditions (pH, temperature, microbiota, and intrinsic proteases) because of their efficacy at increasing IVDMD and IVSD. Previous studies have shown efficacy of exogenous α-amylases at improving nutrient digestibility using in vitro, in situ, and in vivo experimental conditions. An α-amylase from Aspergillus niger increased IVDMD of corn grain when added at 6 mg/g of the substrate (Mora-Jaimes et al., 2002). Similarly, in situ DM digestibility of corn and sorghum grain was increased with an α-amylase from Bacillus licheniformis and glucoamylase from A. niger (Gutiérrez et al., 2005). Velasco (2004) reported that exogenous α-amylases added at 2, 4, and 6 g/kg of the substrate (sorghum grain) were effective in improving IVDMD after 6 and 12 h; however, no effects were observed after 24 and 48 h. Similarly, Mora-Jaimes et al. (2002) reported greater IVSD from sorghum grain treated with α-amylase from B. licheniformis compared with control. Several in vivo studies have shown potential of exogenous α-amylase in improving productivity of dairy and beef cattle (Tricarico et al., 2005; Harrison and Tricarico, 2007; Klingerman et al., 2009; Gencoglu et al., 2010; Meschiatti et al., 2019; Toseti et al., 2020). In addition, α-amylase may improve animal productivity by increasing NDF digestibility regardless of its effects on starch degradability (Bowman et al., 2002; Gencoglu et al., 2010) suggesting that enzymes may promote the growth of fiber degrading bacteria (VargasRodriguez et al., 2014) or that enzyme preparations may include fiber- and protein-degrading along with starch-degrading activities (Adesogan et al., 2014).
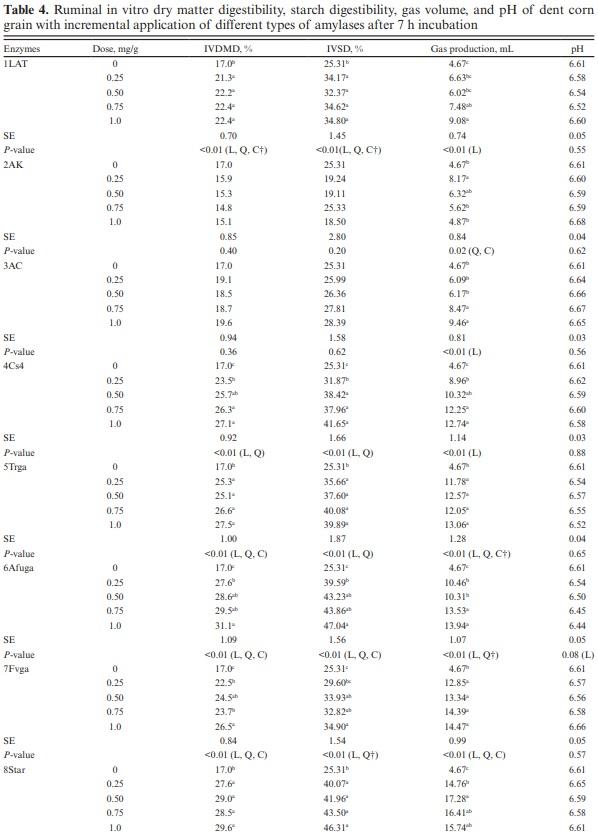
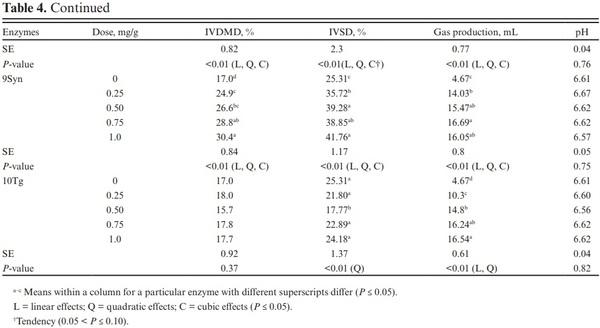
The endosperm cells of corn grain are characterized by the presence of starch granules embedded in a protein matrix. The starch–protein matrix in corn is considered a physicochemical barrier to starch digestion in ruminants (Owens et al., 1986). Previous studies have observed lower in vitro and in situ starch digestibility with extensive crosslinking of zein proteins yielding vitreous endosperm (Philippeau and Michalet-Doreau, 1997; Correa et al., 2002; Ngonyamo-Majee et al., 2008). Therefore, using exogenous proteases may increase starch digestibility by breaking down structural barriers and increasing microbial α-amylase access to starch granules within the endosperm. Exogenous proteases, 11P14L and 12P7L increased IVDMD by 97.6% and 86.7% when compared to control at the lowest dose of application, while enzyme 13P30L linearly increased IVDMD, and an increase of 44.8% was observed at the highest dose of application, compared with the control (Table 5). IVSD was also increased by exogenous proteases with 57% and 64% improvement observed with enzymes 11P14L and 12P7L at the lowest dose application, respectively. Enzymes 13P30L increased IVSD by 30% when applied at the highest dose. In agreement, Colombatto and Beauchemin (2009), when testing an exogenous protease have reported greater IVDMD of several substrates including a TMR after 22 h of incubation. Similarly, studies evaluating the effects of proteases as silage additives have reported greater IVSD when compared with control (Ferraretto et al., 2015a; Ferraretto et al., 2015b; Der Bedrosian and Kung Jr, 2019).
Gas production provides an indirect measurement of digestion of soluble and insoluble carbohydrates (Menke, 1988; Getachew et al., 2005) and originates from microbial degradation of feed and buffering of acids produced during ruminal fermentation (Getachew et al., 2005). The amount of gas produced also reflects production of VFA as when feed is fermented to acetate and butyrate, fermentative gas is produced while propionate synthesis yields gas by buffering of the acids (Getachew et al., 2005). Hence, feed that produces more propionate will produce lower gas compared to feeds producing acetate and butyrate (Beuvink and Spoelstra, 1992). In agreement with IVDMD results, total gas volume was increased with all enzyme applications when compared with control. For most of the enzymes, with the exception of 2AK, gas production was linearly increased with enzyme dose (Table 4). Quadratic response on gas production was observed for all enzymes, except for 1LAT, 3AC, and 4Cs4. Similarly, gas production was linearly increased with exogenous proteases and greater effects observed at higher doses of application (Table 5). The effects observed on gas production with protease application are in agreement with improvements in IVDMD and IVSD. These results are in agreement with several other published studies where gas production was increased with enzyme applications when compared to control (Beauchemin et al., 2003; Eun et al., 2006; Elghandour et al., 2013).
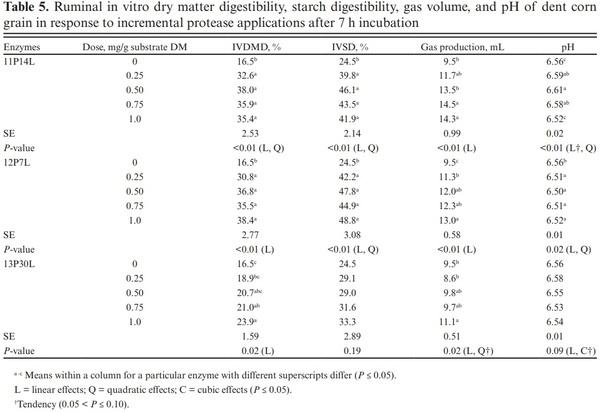
The effects of enzymes 2AK, and 3AC on VFA profile were not analyzed as both enzymes did not improve IVDMD, and IVSD. For other enzymes, total VFA was not affected by enzymes 1LAT, 4Cs4, and 10Tg (P > 0.10; Table 6). However, total VFA was decreased by enzymes 5Trga, 6Afuga, 7Fvga, 8Star, and 9Syn (P < 0.05). Linear, quadratic, and cubic effects were observed for 6Afuga, 7Fvga, and 9Syn. Enzyme 5Trga decreased total VFA in a linear and quadratic manner while only linear effects were observed for enzyme 8Star. Molar proportion of acetate was not affected by exogenous α-amylase and most glucoamylases, except for the linear decrease observed with 6Afuga, while quadratic effects were observed with 7Fvga. No treatment effects were observed on propionate (P > 0.10), acetate-to-propionate, and butyrate, except for a linear decrease in butyrate proportion with enzyme 10Tg (P = 0.01). Enzymes 1LAT, 5Trga, 6Afuga, 7Fvga, 8Star, and 9Syn decreased iso-butyrate (P < 0.05) while 4Cs4, 6Afuga, 7Fvga, 8Star, and 9Syn increased iso-valerate proportions (P < 0.05).
No effects were observed with exogenous proteases on total VFA profile along with the molar proportions of acetate, propionate, and iso-butyrate (Table 7). Due to the lack of effect on VFA profile, only the two lowest doses (0.25 and 0.50 mg/g) of proteases were analyzed. The acetate-to-propionate ratio was also similar for all treatments (P > 0.10).
Butyrate increased at a higher dose of 11P14L (P < 0.01) while no effects were observed with 12P7L. The lack of effects observed on VFA profile despite increases in IVDMD, IVSD, and gas production is atypical. We expected the IVDMD, IVSD, and gas production increases with enzyme application to be accompanied by an increased VFA production. Getachew et al. (2004) observed a significant correlation between in vitro true digestibility (IVTD) and total VFA (r = 0.33; P < 0.05); however, except for propionate, individual VFA concentration was not correlated with IVTD. Substrates digested in rumen are partitioned into VFA, gas production, and microbial biomass. The VFA production does not account for substrate utilized for microbial growth. We speculate that greater nutrient digestibility with α-amylase and glucoamylases may have partitioned digested substrate towards increased microbial biomass (Getachew et al., 2005). We can also speculate lag phase between substrate degradation and VFA production and with a shorter incubation period in this study, we may have missed treatment effects on VFA profile. The conflicting VFA response can also be related to a shift in the fermentation pathway from propionate to lactate as suggested earlier (Beuvink and Spoelstra, 1992); however, lactate concentrations were not measured in this study and we cannot validate this speculation for lower VFA concentration. The effects of enzyme addition on microbial abundance and activity can also reduce total VFA concentration; however, lack of enzyme effect on IVDMD and IVSD does not support this argument. We did not analyze total and individual VFA profile before sample incubation and the confounding effects of VFA profile from donor cows may have altered treatment effects on VFA profile after 7 h of incubation. With similar rumen inoculum used for control and enzyme samples and with three replications over time, we should be able to rule out this possibility.
When highly fermentable sugars are abundant under low ruminal pH conditions, some rumen bacteria such as Selenomonas ruminantium and Streptococcus bovis produce lactate from pyruvate via lactate dehydrogenase (Beuvink and Spoelstra, 1992). However, when fermentable carbohydrates are limiting, the fermentation pattern reverts back to VFA production (Russell and Strobel, 1988). Lactic acid accumulates during initial hours of fermentation and it can be metabolized by lactate-utilizing bacteria, such as Megasphaera elsdenii, S. ruminantium, and Veillonella parvula into propionate (Dawson et al., 1997; Russell and Wallace, 1997). While accumulation of lactic acid is a possibility during initial phase of fermentation with only 7 h incubation; it may not be applicable in this study as donor cows were fed normal lactation diet and rumen microflora may have been adapted to utilize lactic acid preventing any incidences of lactic acid accumulation.
Cotta (1988) reported that six strains of rumen microbes with extracellular α-amylase activity produced malto-oligosaccharides and little free glucose suggesting they were not effective at hydrolyzing starch into glucose. We can speculate that though exogenous amylase addition increased IVSD in the current study, the starch may have been broken down into malto-oligosaccharides instead of free glucose, which may have limited its fermentation into VFA. Rather microbes may have had to rely on their own enzymes to further breakdown these oligosaccharides into glucose, pyruvate and subsequently VFA.
CONCLUSION
Addition of seven out of 10 exogenous α-amylases, glucoamylases, and α-glucosidase and all exogenous proteases tested increased IVDMD and IVSD of mature dent corn grain. These effects induced greater gas production but total VFA concentration was not consistently increased. Based on the results observed in these experiments, four exo-acting glucoamylases; 4Cs4, 5Trga, 6Afuga, and 7Fvga and two neutral exogenous proteases 11P14L and 12P7L were the most promising enzymes. In order to validate these findings, future in vivo studies using multiple corn hybrids, along with best addition strategies are required before these enzyme additives can be recommended for improving the digestibility of mature dent corn grain.
This article was originally published in Translational Animal Science 2021.5:1-16. doi: 10.1093/tas/txaa222. This is an Open Access article distributed under the terms of the Creative Commons Attribution Non-Commercial License (http://creativecommons.org/licenses/by-nc/4.0/).