Introduction
Precision nutrition aims to design nutritional recommendations in order to increase metabolic efficiency, reduce costs, improve product quality, minimize environmental impact while improving the health and well-being of dairy cattle at the individual level. According to Gonzales et al. (2018):
“Precision animal nutrition requires the application of principles, techniques and technologies that automatically integrate biological and physical processes related to animal nutrition using remote monitoring, modelling and control tools that allow making precise, accurate and timely decisions”.
The level of precision achieved is, however, dependent of the understanding of the biological processes involved. Nutrient supply and demand are the main targets to manage but limiting the modelling tools to control energy and major nutrient balance is likely to leave large portions of the variability unacknowledged for. Minor nutrients, such as trace metals and vitamins, are involved in major metabolic pathways, their supply affecting metabolic utilization of energy and major nutrients. Consequently, an inadequate supply of minor nutrients could explain some of the discrepancies between the outcomes of prediction models and observed animal performance under conditions otherwise similar.
Among the minor nutrients required for the physiological functions essential to life are B vitamins. Unlike other nutrients, the vitamins do not serve structural functions, nor does their catabolism provide significant energy. B vitamins play different key roles in metabolism but they all share a common role as coenzymes, metabolites essential for the catalytic activity of enzymes (Combs and McClung, 2017).
In dairy cows, with the exception of vitamin B12, B vitamins are provided by the diet and synthesis in the rumen. Vitamin B12 supply is entirely dependent of its synthesis by the rumen microbiota, if the cobalt supply is sufficient. In mature ruminant, the amount of B vitamins provided by the diet and synthesized by the ruminal microflora is generally sufficient to prevent apparition of deficiency symptoms. Nevertheless, deficiency symptoms are the last stage of the deficiency; deficiency appears as soon as the supply is inferior to the need leading to a loss of metabolic efficiency (Combs and McClung, 2017). Indeed, lack of a coenzyme would progressively slow down the metabolic reaction and then, drive to a shift towards an alternative pathway not requiring this coenzyme but with a greater energy cost. Beneficial effects of B-vitamin supplementation suggest that, even in mature cows, the need for B vitamins can sometimes exceeds the supply from the diet and the synthesis by rumen microbiota, reducing metabolic efficiency and leading to suboptimal milk production.
The present paper is not an exhaustive description of the importance of each B vitamin in dairy cow nutrition. Its objective is to illustrate by an example, in the present case, folic acid and vitamin B12, how an inadequate supply of minor nutrients could impact metabolism and lactational performance of dairy cows.
What is the Interest of Folates and Vitamin B12 for Dairy Cow Nutrition?
Metabolic Roles of Folates and Vitamin B12
Folates are enzyme co-substrates for the transfer of one-carbon units obtained from formate or catabolism of amino acids, such as serine, glycine, histidine. The one-carbon units provide through folate metabolism are essential for DNA synthesis and replication, and thus, cell division. Folate metabolism also provides one-carbon units for de novo synthesis of methyl groups for formation of the primary methyl donor for biological methylations, S-adenosylmethionine.
S-adenosylmethionine transfers its methyl group in many reactions, among them DNA methylation, which controls gene transcription and genetic stability, and synthesis of phosphatidylcholine, creatine and neurotransmitters (Choi and Mason, 2000).
Folate metabolism plays a major regulatory role in milk protein synthesis in mammary epithelial cells (Menzies et al., 2009a). In many mammal species including dairy cows, it was observed that, during periods of increased milk protein secretion, the greatest change in gene expression in mammary epithelial cells is for the folate receptor alpha (FOLR1) which allows the entry of folates into the cells (Ramanathan et al., 2007; Menzies et al., 2009b; Menzies et al., 2009a). These observations were supported by the fact that hormonal stimulation of milk protein synthesis in mammary explants from dairy cows increased expression of FOLR1 and ALDH1LI. The later enzyme allows the entry of one-carbon units into the folate cycle (Menzies et al., 2009b).
Vitamin B12 acts as coenzyme in only two metabolic reactions. The vitamin is the coenzyme for the cytosolic enzyme, methionine synthase, the critical interface between folates and vitamin B12 which allows the transfer of the methyl group from the 5-methyl-tetrahydrofolate to homocysteine for regeneration of methionine. Besides this role, the vitamin is the coenzyme for the mitochondrial enzyme, methylmalonyl-coenzyme A mutase, essential for the entry of propionate into the Krebs cycle and gluconeogenesis (Combs and McClung, 2017).
Metabolism of these two vitamins is tightly linked. In most mammals, a vitamin B12 deficiency diverts all available one-carbon units towards the synthesis of 5-methyl-tetrahydrofolate, a form of folates which can be demethylated only by the vitamin B12-dependent enzyme, methionine synthase. Consequently, a lack of vitamin B12 leads to a secondary folate deficiency due to the accumulation of folates under a form, 5-methyl-tetrahydrofolate, which can no longer be used by the cells (Scott, 1999). This link was confirmed in dairy cows during a study looking at the effects of increasing doses of folic acid in the diet, 0, 2 and 4 mg of folic acid per kilogram of body weight given daily to 32 primiparous and 31 multiparous cows from one month before the expected date of calving until 305 d of lactation (Girard and Matte, 1998). Overall, for the complete lactation, supplementary folic acid had no effect on milk production and composition of primiparous cows although milk production decreased linearly with the increase in folic acid supplementation during the first 100 d of lactation, 28.8, 27.0 and 26.6 kg/d for 0, 2 and 4 mg/kg body weight, respectively. In multiparous cows, however, supplementary folic acid was associated with a linear increase in milk production (8284, 8548 and 8953 kg for cows fed diets supplemented with 0, 2, and 4 mg of folic acid/kg of body weight per day, respectively) and milk casein yield during the complete lactation (3 to 305 d after calving). The effect was greater during the first 200 days in milk. In these cows, folic acid supplementation also decreased milk non-protein nitrogen concentration during the first 100 d of lactation supporting the link between folic acid supply and the efficiency of nitrogen utilization for protein synthesis in the mammary gland. A likely explanation for this difference between the response of primiparous and multiparous cows to the folic acid supplementation is the vitamin B12 status of both groups. Overall during the lactation, plasma concentration of vitamin B12 was lower for primiparous cows and this difference was especially marked during the first 8 weeks of lactation when average plasma concentration of the vitamin was around 170 pg/mL in primiparous cows (Girard and Matte, 1999; Figure 1).
Figure 1. Serum concentration of vitamin B12 during lactation of primiparous and multiparous cows (adapted from Girard and Matte, 1999).
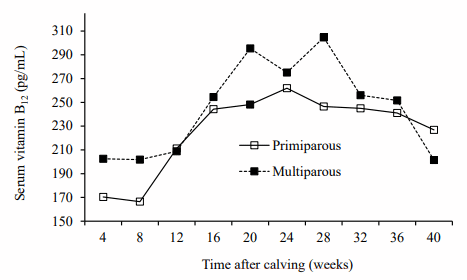
The presence of a secondary folic acid deficiency due to a lack of vitamin B12 was further confirmed by two experiments conducted simultaneously. In the first one, from one month before calving until 305 d of lactation, 54 multiparous Holstein cows were either fed a diet calculated to supply methionine as 1.75% metabolizable protein, equivalent to 70% of methionine requirement or the same diet supplemented with a rumen-protected methionine supplement to bring the estimated methionine supply to 2.2% of metabolizable protein. Within each diet, the cows received daily 0, 3, or 6 mg/d of folic acid per kg of body weight (Girard et al., 2005). In the second experiment, from the 4th to the 18th weeks of lactation, 14 primiparous Holstein cows were fed the same basal diet than in the first experiment supplemented daily with rumen-protected methionine and 4 mg of folic acid per kg of body weight per day and then, received either a weekly intramuscular injection of saline or 10 mg of vitamin B12 (Girard and Matte, 2005). In the first experiment, there was no effect of supplementary folic acid, methionine or their interactions on milk and milk component yields. It is noteworthy that serum concentration of vitamin B12 was low throughout the lactation but especially so, in early lactation, 181, 232, 257 and 265 at 8, 18, 28 and 38 weeks of lactation, respectively. In the second experiment, in control cows, serum concentration of vitamin B12 was stable throughout the experimental period, under 200 pg/mL. In this experiment, supplementary vitamin B12 increased energy-corrected milk from 25.8 to 29.0 kg/d as compared to cows fed only the dietary supplement of folic acid. Interestingly, packed cell volume and blood hemoglobin were greater when cows received the vitamin B12 supplementation. The increases in packed cell volume and blood hemoglobin concentrations in cows supplemented with folic acid and vitamin B12 suggest that, despite an abundant supply of folates, its utilization for DNA biosynthesis was not optimal under the basal conditions. Moreover, serum methylmalonic acid concentration was lower when cows received the vitamin B12 supplementation. In absence of sufficient vitamin B12 supply, methylmalonyl-CoA from catabolism of propionate accumulates and is degraded in methylmalonic acid (Scott, 1999). Serum methylmalonic acid provides a very sensitive index of vitamin B12 deficiency (Selhub et al., 2009). The decrease in serum concentrations of methylmalonic acid in cows injected with supplementary vitamin B12 further supports the hypothesis that a suboptimal supply in vitamin B12 during early lactation could affect folate utilization and efficiency of energy metabolism as supported by the increased energy-corrected milk yield without changes in dry matter intake.
The opposite situation, a secondary vitamin B12 deficiency due to a lack of folates, has been observed in humans (Selhub et al., 2007) and dairy cows (Graulet et al., 2007; Preynat et al., 2010; Duplessis et al., 2017a). A supplement of folic acid and vitamin B12 increased the affinity of the enzyme, methylmalonylCoA mutase, for vitamin B12 when compared to a supplement of vitamin B12 alone (Graulet et al., 2007). Supplementary vitamin B12 also increased MUT expression, the gene responsible for synthesis of methylmalonylCoA mutase, when cows received the folic acid supplement whereas it had no effect when cows did not receive the folic acid supplement (Duplessis et al., 2017a). Similarly, in the study of Preynat et al. (2010), the highest MUT expression in liver was obtained with the combined supplement of folic acid and vitamin B12. However, MUT expression also tended to be greater in cows receiving only the supplement of folic acid than in control cows. Although folates is not involved in the methylmalonyl-CoA mutase pathway, an adequate supply in folates is essential to the transfer of vitamin B12 from the cytosol to the mitochondria (Riedel et al., 1999).
Transition Period and Early Lactation, Critical Periods for the Folate and Vitamin B12 Balance
As illustrated in Figure 1, the lowest serum concentration of vitamin B12 was observed in early lactation. Colostrogenesis leads to a rapid secretion of large amounts of folates and vitamin B12. The amounts of folates and vitamin B12 secreted in the first colostrum are 1.5 and 2.6 times the amounts secreted in milk collected at the morning milking during the first weeks of lactation (Table 1; Duplessis et al., 2015).
Table 1. Amounts of folates and vitamin B12 secreted in colostrum and milk of dairy cows fed a dry cow diet providing 1.14 Mcal NEm/kg DMa
Dietary changes and low dry matter intake during the transition period could reduce folate and vitamin B12 supply during this period of high demand for secretion in colostrum. In addition, due to the metabolic roles of these vitamins, it is to be expected that the consequences of improving metabolic efficiency would be more critical during this period of negative energy and nutrient balance. Consequently, many experiments looking at the effects of supplementing these two vitamins were conducted during the transition period and in early lactation.
Production and Metabolic Responses of Cows to a Combined Supplement of Folic Acid and Vitamin B12
In 7 experiments conducted in cows during the transition period and in early lactation, a combined supplement of folic acid and vitamin B12 had no effect on dry matter intake. The supplement increased milk production in 5 of them (Table 2). Milk total solid, protein, lactose and fat yields were increased by the combined supplement of folic acid and vitamin B12 in 5, 4, 4 and 1 out of these 7 experiments, respectively. Although nutrient composition of the diets fed during these experiments were calculated to meet the NRC (2001) recommendations; the ingredients used, especially forages, differed among experiments.
Table 2. Milk response to a folic acid and vitamin B12 supplement given from 3 weeks before the expected date of calving until 8 weeks of lactation, except for experiment 7 which lasted until 3 weeks of lactation
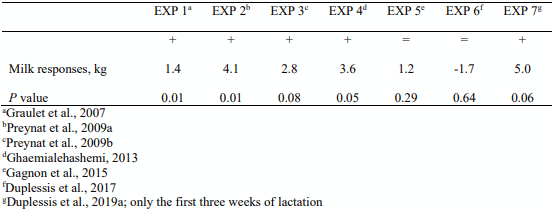
An experiment was also conducted in 15 farms, involving 805 cows. In this experiment, all cows received either weekly intramuscular injections of saline (control) or 320 mg of folic acid and 10 mg of vitamin B12 from 21 days before the expected date of calving until 60 days in milk. Overall, the combined supplement of vitamins increased milk protein content from 30.9 to 31.5 g/kg and decreased milk fat content from 42.1 to 40.3 g/kg, then, decreasing the fat-to-protein ratio. Body condition score and body weight losses were also reduced after calving by the vitamin supplement; during the first 60 d of lactation, body weight losses were 30 and 23 kg for controls and cows injected with vitamins, respectively. The reduction of the fat-to-protein ratio and the body weight losses without effect on milk yield suggest that supplementary folic acid and vitamin B12 improved energy balance in early lactation (Duplessis et al., 2014a). Moreover, in cows at their 2nd lactation or more, the vitamin supplement shortened the interval between calving and 1st insemination by 3.8 days and reduced by 50% calving problems (Duplessis et al., 2014b). Indeed, Gagnon et al. (2015) showed that a combined supplement of folic acid and vitamin B12, at the doses used in the farm trial during the same physiological period, upregulated genes related to follicle differentiation and ovulation suggesting that the supplement promoted a more rapid growth of the dominant follicle. Using an identical experimental design, Ghaemialehashemi (2013) observed that the vitamin supplement increased the number of large follicles and the size of the dominant follicle. He also observed a numerical decrease of the number of days in milk at the first insemination. In both experiments, body reserve mobilization seems to have been reduced by the vitamin supplement without change in dry matter intake (Gagnon et al., 2015; Ghaemialehashemi, 2013).
Variability of Production and Metabolic Responses of Cows to a Combined Supplement of Folic Acid and Vitamin B12
Another interesting observation from the farm trial is the variability of the response to the combined supplement of folic acid and vitamin B12. Variability could have been expected based on results from controlled experiments described previously. However, the farm trial gave us the opportunity to study the responses in cows receiving the same treatments during the same physiological stages but under different management conditions. Figure 2 illustrated the fat-toprotein ratio and body weight loss in early lactation observed in the 15 farms. A lower fat-toprotein ratio following use of the vitamin supplement was observed in 13 farms. Not surprisingly, BW loss differed among farms in control cows, varying from 9 to 40 kg. Nevertheless, in 12 farms out of 15, body weight loss was smaller in cows receiving the vitamin supplement, varying from 0 to 37 kg.
Figure 2. Milk fat-to-protein ratio (a) and body weight loss (b) from calving until 60 days in milk in cows receiving weekly intramuscular injection of saline (control) or 320 mg folic acid and 10 mg of vitamin B12 from 3 weeks before the expected date of calving until 60 days in milk (Adapted from Duplessis et al. 2014a).
Dietary Factors Affecting Folate and Vitamin B12 Status of Dairy Cows
No blood samples were collected to estimate the folate and vitamin B12 status of the cows during this on-farm experiment. However, in control cows, milk concentrations of vitamin B12 varied among farms from 2.25 to 3.84 ng/mL suggesting that the vitamin status differed among farms. Based on diets fed in the 15 farms involved in the experiment, Duplessis et al. (2016) detected a positive relationship between milk vitamin B12 concentration and dietary acid detergent fiber content and a negative relationship between milk concentration of the vitamin and dietary crude protein content. The former relationship was confirmed by a survey on 100 dairy farms in Québec showing that vitamin B12 concentration in milk was positively related to percentage of fiber (acid detergent and neutral detergent fibers) and negatively related to non-fiber carbohydrate and starch as well as energy of the diet (Duplessis et al., 2019b). Relationships between folate status and dietary factors were not explored in these two studies (Duplessis et al., 2016; 2019b)
A cross-sectional study conducted on Holstein cows at similar stages of lactation across United States and Canada looking at the effect of diet composition on plasma concentrations of folates and vitamin B12 showed that plasma folate concentrations decreased with dietary fiber concentrations (i.e. neutral and acid detergent fibers and lignin) but increased with dietary nonfiber carbohydrate concentrations. The opposite relationships were observed for plasma vitamin B12 concentrations. For example, in this study, the lowest plasma concentration of folates was observed in Californian farms feeding diets with high fiber concentrations whereas the lowest plasma vitamin B12 concentration was reported in Quebec and New York state farms feeding diets with high non-fiber carbohydrate concentrations (Table 3). It is noteworthy, however, that plasma concentrations of folates and vitamin B12 are not highly correlated. Consequently, in spite of this apparent contradiction, a high status in both vitamins can be achieved by feeding diets providing average fiber and non-fiber carbohydrate concentrations as compared to these extremes (Table 3; Duplessis et al., 2020).
Table 3. Average dietary content of fibers and non-fiber carbohydrates (NFC) in areas with different plasma concentrations of folates and vitamin B12a
A meta-analysis on data from 340 lactating cows involved in 16 published studies showed that the amount of vitamin B12 reaching the duodenum, i.e. the amount available for absorption by the cow, is positively related to dry matter intake but inversely related the dietary starch content (Brisson et al., University of Guelph, personal communication). On the other hand, duodenal flow of folates is best predicted by positive relationships with dry matter intake and dietary concentrations of folates and starch (Brisson et al., personal communication). It is noteworthy that in all the studies cited in the present manuscript, cobalt supply was not limiting, it was always at or over the NRC (2001) recommendations.
Differences in diet composition likely entail changes in composition and activity of the rumen microbiota which could alter B-vitamin synthesis and use in the rumen. Magnusdottir et al. ( 2015) demonstrated that not all bacterial species present in gut are able of de novo synthesis of B vitamins; these species are consequently dependent of dietary B-vitamin supply and/or provision by commensal bacteria. Therefore, B-vitamin supply could be modified by alterations of the gut microbiota composition. In dairy cows, results from an observational study support this conclusion. This study showed that abundance of vitamin B12 in the rumen of dairy cow is better linked to the absence of high vitamin B12 consumers than to the presence of efficient vitamin B12 producers. Therefore, to some extent, bacterial consumption of vitamin BB12 2 in the rumen may have a greater influence on the amount of vitamin B12 available for the cow than bacterial production of the vitamin (Franco-Lopez et al., 2020). Knowledge on the relationships between rumen microbiota and B-vitamin supply is extremely limited. This exploratory study highlights the importance of increasing knowledge on rumen microbiota and factors affecting its composition to enable prediction of B-vitamin supply from synthesis in the rumen. Nevertheless, these different approaches help to progressively define dietary conditions to maximize vitamin B12 supply. A similar approach still needs to be conducted for the other B vitamins.
Conclusion
This example supports the concept that, in dairy cows, B-vitamin intake could be insufficient to meet the need. Similar data exist for some other B vitamins (Schwab et al., 2005; Lean et al., 2011). Precision of the nutritional modelling tools is dependent on the acquisition of knowledge on the effects of minor nutrients, such B vitamins, on the efficiency of major metabolic pathways. Given the improvements in production and metabolic efficiency observed when B-vitamin needs are fulfilled, it is clear that controlling energy and major nutrient balance is no longer sufficient to optimise cow nutrition. Precision nutrition is dependent on the ability of the models to evolve taking into account new knowledge. Meanwhile, it is obvious that research is needed to better identify the physiological periods and management conditions leading to a negative balance between B-vitamin supply and metabolic need of the cow and to support the decision of either to use supplements or to change diet composition to avoid deficiency.
Presented at the 2021 Animal Nutrition Conference of Canada. For information on the next edition, click here.