Selenium (Se) is an essential trace element and has been documented to be important for the health of both animals and humans (Rayman, 2000; McKenzie et al., 1998). However, selenium intake is generally low in many areas of the world, particularly the Nordic countries, where content of selenium in soil is low. Thus, feed for livestock is supplemented with selenium, mostly as inorganic sodium selenite, although bioavailability of inorganic selenium is lower than that of organic forms (Ortman and Pehrson, 1999). Selenium is indicated, together with vitamin E, as necessary in controlling mastitis (Hemingway, 1999; Smith et al., 1997), and an increased intake and incorporation of organic selenium may therefore improve animal health, and is expected to lower somatic cell counts in milk and result in higher milk quality.
Oxidation of milk is a serious problem for the dairy industry; thus, enhancing the natural, endogenous antioxidant content of milk becomes critical for product stability and robustness. Selenium is specifically required for important antioxidant enzymes, such as glutathione peroxidases and thioredoxin reductases; and oxidative stability of dairy products may therefore be improved if higher levels and activities of these enzymes can be attained in milk. This may be accomplished with either organic or inorganic forms of Se, the latter typically being sodium selenite. However, if organic Se in the form of the naturally-occurring, but low-abundant amino acid selenomethionine is enriched in the diet, Se will become a significant part of a much wider spectrum of proteins and may thereby provide novel antioxidant properties. In the experiments described herein, we supplemented dairy cows with high levels of selenium in the form of Sel-Plex® in order to maximize transfer of selenium to milk.
Selenium may have beneficial health effects beyond those documented, and it is becoming increasingly apparent that levels of selenium intake based solely on preventing deficiency symptoms may be less than
optimal (Jackson et al., 2003). Selenium has been implicated as a booster of immune function, an antidepressive agent, and in protection against cancer (Finley et al., 2001; Klein et al., 2003). Health benefits in humans from increased intake of Se-enriched, high quality dairy products will be determined in future studies.
Oxidation of milk proteins: does this initiate lipid oxidation?
Oxidation of milk lipids is known to result in a number of degradation products that cause inferior taste and odor and thus product deterioration. However, initiation of lipid oxidation is presumably caused by transition metal ions, like iron and copper, which are normally associated with amino acids and proteins. Therefore, amino acid residues in proteins are likely initial targets of oxidation, and the redox properties of the amino acids surrounding initial free radical sites will be critical for subsequent oxidation. The sulfur-containing amino acids, methionine and cysteine, are particularly vulnerable to oxidation, in fact the exposed free thiols of cysteine disappear rapidly from foods such as milk.
Methionine may be oxidized to methionine sulfoxide by molecular oxygen and other reactive oxygen species and the surface location of methionine residues in various proteins indicates that this may be an important antioxidant defence mechanism for protection of other more vulnerable sites of oxidation, i.e. catalytic residues (Levine et al., 1996). The evolution of and requirement for a specific mechanism for subsequent regeneration of native methionine by the action of methionine sulfoxide reductases further demonstrates the relevance of this defence system (Moskovitz et al., 1997; Ruan et al., 2002).
Thus, both methionine and cysteine may be important endogenous antioxidants in milk. Selenium is, however, a better nucleophile than sulfur and thus more prone to react with electrophiles. Selenium is an essential trace element and absolutely required for several proteins involved in various antioxidant defence systems. Selenium is specifically incorporated into these proteins as selenocysteine and directly functions as the catalytic site for reduction of various substrates, e.g. organic hydroperoxides. A family of proteins in this group is the glutathione peroxidases (GSH-Px) with six members, each with specialized functions or cellular/tissue-specific locations. These enzymes may use glutathione or thioredoxin as electron donors, and oxidation of either glutathione or thioredoxin requires the subsequent action of either glutathione reductase or thioredoxin reductase, another Se-cysteine protein, where both enzymes use NADPH as an electron donor. Thus, ultimately the reducing capability depends on energy status, since NADPH is generated either by the pentose shunt (anaerobic glycolysis) or the mitochondrial citrate cycle (aerobic glycolysis).
Whereas only selenocysteine is specifically incorporated during translation into particular proteins in response to a special codon (Lacourciere et al., 2002), selenomethionine will be randomly incorporated in place of methionine in all proteins made. Thus, proteins with structural or non-redox functions will then contain novel catalytic sites in the form of selenoamino acids instead of the normal sulfur amino acids and thereby may provide additional antioxidant properties. Particularly selenomethionine is important, since protein methionine residues are believed to provide antioxidant protection (Stadtman et al., 2002). Why is selenomethionine catalytic? Because non-enzymatic, direct reduction of methionine selenoxide occurs with thiols and other lowmolecular weight reductants, e.g. ascorbic acid (Assmann et al., 1998) in contrast to the reduction of methionine sulfoxide, which requires the action of specific reductases (Kryukov et al., 2002; Grimaud et al., 2001). Selenomethionine residues in proteins will therefore function as catalytic antioxidants until lowmolecular reductants are exhausted. This may be exploited to produce dairy consumables of superior quality and stability.
Antioxidant properties of high-Se milk
INCORPORATION OF SEL-PLEX® SELENIUM IN MILK
Two groups of cows, control and Se-supplemented, with three cows per group were used in a cross-over study. Both treatment groups received a basal diet containing 0.1 ppm Se, which supplied ~2 mg Se daily. The Se supplemented group received an additional 25 mg Se daily in the form of Sel-Plex® (Alltech Inc.).
Selenium appears rapidly in milk after a delay of only one day (Figure 1). Sel-Plex® supplementation at ~25 mg Se per day results in a level of ~140 mg/L, or approximately 10-fold that of the controls.
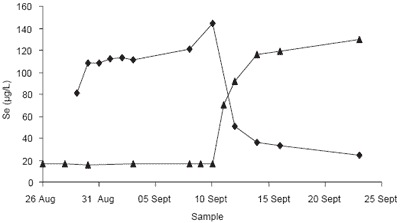 |
Figure 1. Milk from three cows per treatment ( at start are controls until cross-over) was sampled daily and pooled for selenium analysis using atomic absorption spectroscopy. Amount of selenium in milk is shown as a function of days after experimental supplementation: start 26th of August, cross-over 9th of September. |
MEASUREMENT OF TOTAL ANTIOXIDANT CAPACITY IN MILK
Milk levels of 150 μg/L Se correspond to ~2 μM. If all selenium occurred as selenomethionine, the expected direct antioxidant effect would be small, at least as determined by the ABTS assay (Figure 2). Thus, at least 100 times more selenomethionine would be required for a direct antioxidant effect.
Using the ABTS assay shown in Figure 2, we compared the hydrogen peroxide/lactoperoxidase-induced formation of ABTS·+ radicals in the control milk and selenium-enriched milk and the direct effect observed after addition of methionine or selenomethionine to the same two milks (Figure 3).
This experiment shows that formation of ABTS·+ radicals is reduced by ~20% in Se-enriched milk compared to control milk, and that 2 mM selenomethionine would be necessary for a direct antioxidant effect, i.e. 1000 times more than represented by the amount of selenium determined in the milk. Thus, the data show that there is an increased antioxidant capacity in Se-enriched milk, but this increased capacity is not due to a direct stoichiometric effect of selenomethionine.
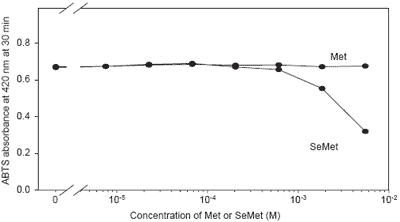 |
Figure 2.Methionine (Met) or selenomethionine (SeMet) were added to milk from a 0.1 M stock solution in 10 mM HCl (20-fold dilution), and ABTS·+ radical formation measured after 30 min following addition of ABTS and hydrogen peroxide (0.1 mM). |
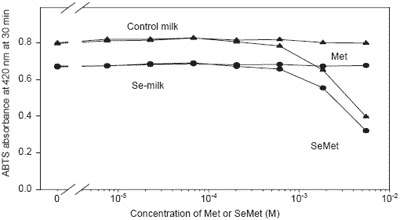 |
Figure 3.Methionine (Met) or selenomethionine (SeMet) were added to control or Se-enriched milk from a 0.1 M stock solution in 10 mM HCl (20-fold dilution), and ABTS·+ radical formation measured after 30 min following addition of ABTS and hydrogen peroxide (0.1 mM). |
STORAGE AND INDUCED OXIDATION EXPERIMENTS
A number of experiments have been conducted to determine the oxidative stability of Se-enriched milk compared to control milk. Measures of oxidation have been primarily hexanal (lipid oxidation) and formation of dityrosine (protein oxidation).
Analysis of volatiles after storage for 8 days at 4ºC
Pasteurized selenium-enriched milk and control milk were stored at 4oC for 8 days. Aliquots of 2 ml were analysed by solid phase microextraction gas chromatography (SPME-GC) using a carbon-coated fiber equilibrated for 15 min at 43oC in a sealed vial containing the milk. GC analysis was performed on a 50 m column using a temperature gradient of 25 oC/ min from 50-250 oC. Typical chromatograms from selenium-enriched milk and control milk are superimposed in Figure 4; and the peak areas are plotted in Figure 5. Note that the dynamic range for detection is more than three orders of magnitude (smallest peak area ~100 AU, largest ~300000 AU). The plot in Figure 5 allows visualization of which peaks are more (above diagonal) or less (below diagonal) abundant in seleniumenriched milk compared to control milk. Due to the logarithmic scale, peaks outside the diagonal appear more similar than they actually are. Table 1 shows the most relevant peaks, areas, ratios, and identifications.
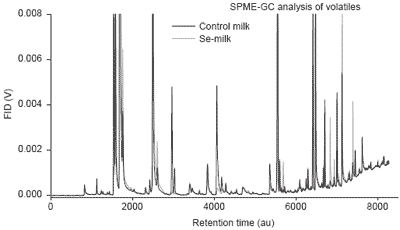 |
Figure 4.Chromatogram examples of volatiles from control milk and milk from cows given 25 mg Sel-Plex® Se daily. |
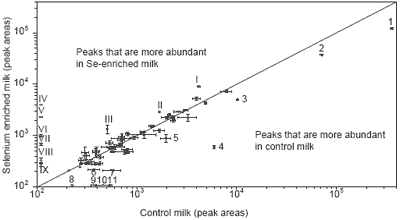 |
Figure 5.Plot of peak areas from Figure 4 (mean and SEM of 12 replicates). |
Table 1.Identification, areas and ratios of peaks noted in Figures 4 and 5. |
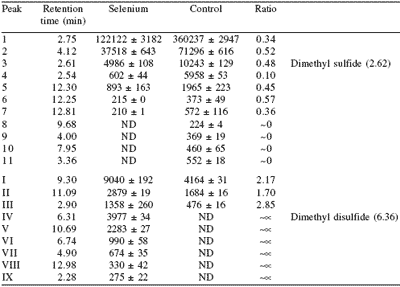 |
Most volatiles (total number of 58 peaks were detected and quantified) are similar for Se-enriched milk and control milk, i.e. appear at, or close to, the diagonal in Figure 5. Peaks 1-5 are reduced in Se-enriched milk, particularly peak 4, which is a rather dominant peak in control milk. Peaks 6-11 are low abundant peaks and are less certain. Peaks I-IX are increased in Se-enriched milk, particularly peaks IV-V which were not detected in control milk.
Lipid oxidation, as assessed by formation of hexanal (retention time 7.78 min, not included in Table 1), was reduced by ~15% in Se-enriched milk compared to control milk in this 8-day storage experiment, i.e. comparable to light-accelerated formation at 10 hrs (see below). Hexanal peak areas were 1988 ± 124 vs. 2324 ± 131 for Se-enriched milk and control milk, respectively.
If Se-enrichment of milk had a general, antioxidant effect, we would expect similar reduction in all oxidation products. Clearly, there are major differences as discussed above, and experiments are in progress to determine which peaks are generated during storage and to identify the molecular species for these particular peaks.
LIGHT-INDUCED OXIDATION
Formation of lipid oxidation products, primarily hexanal, was induced by exposure to light (2000 Lux, 4-6 oC) as a function of time. Volatiles, including hexanal, were analysed by solid phase microextraction gas chromatography (SPME-GC), and amount of hexanal quantified by integration of peak areas. Dityrosine formation was determined by HPLC separation on C18 with fluorescent detection of amino acids after complete acid hydrolysis of delipidated samples and normalized to tyrosine content. This dityrosine:tyrosine ratio had considerably lower variation between replicates than measurement of dityrosine alone.
Results are shown in Figure 6. Both hexanal and dityrosine formation are affected by Se-enrichment, but whereas lipid oxidation (hexanal) is decreased by ~15%, protein oxidation (dityrosine) increases. A simple explanation is therefore that incorporation of selenomethionine into milk proteins renders them more susceptible to oxidation and correspondingly reduces lipid oxidation, but it remains to be determined whether there is a causal relationship between the reduced lipid and increased protein oxidation.
Oxidation was also induced by addition of hydrogen peroxide, which triggers the endogenous lactoperoxidase, and copper ions (Cu2+) (see Figure 7). Whereas Cu2+ did not induce formation of dityrosine compared to control, addition of hydrogen peroxide caused a rapid formation of dityrosine in control milk, but much less in Se-enriched milk. Thus, protein oxidation, measured as formation of dityrosine, was reduced to 20-25% of that observed in control milk.
The magnitude of this effect was unexpected and scientifically very intriguing. A series of experiments were then conducted to address the antioxidant mechanism(s) causing this effect.
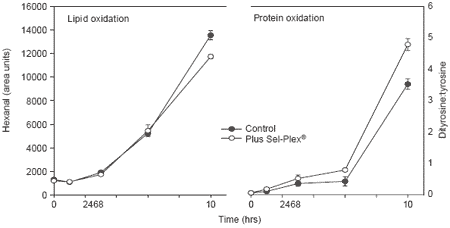 |
Figure 6.Oxidation in control milk and milk from cows given Sel-Plex® as a function of time of exposure to light. |
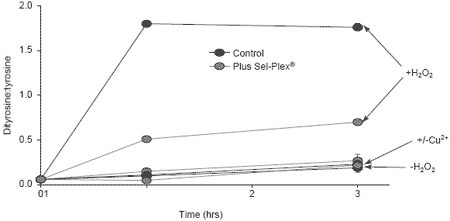 |
Figure 7.Protein oxidation as a function of time after addition of hydrogen peroxide or Cu2+. |
We focused on lactoperoxidase, a prominent enzyme in milk that hydrolyses hydrogen peroxide. Our working hypothesis was that intrinsic differences in lactoperoxidase, due to a partial but significant replacement of its twelve methionine residues with selenomethionine, would lead to a substantial difference in catalytic activity.
Figure 8 shows that lactoperoxidase in Se-milk appears to have a substantially lower activity, whereas radical scavenging activity measured as quenching of chemically pre-formed ABTS·+ radicals is not affected (Figure 9). Next, we purified lactoperoxidase from control and Se-enriched milk on a strong cation-exchange column, the elution of which is shown in Figure 10. For comparison, purified lactoperoxidase and lactoferrin were also run. When activity of lactoperoxidase was measured in the individual fractions (Figure 11), lactoperoxidase from Se-milk was substantially less active than that obtained from control milk.
Analysis of the purified lactoperoxidase fractions showed that Se-milk contained a novel protein band compared to control milk (see Figure 12). The identity of this band has not been elucidated.
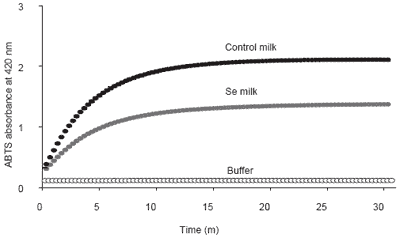 |
Figure 8.Activity of lactoperoxidase measured as formation of ABTS·+ radicals in milk as a function of time (m=min). |
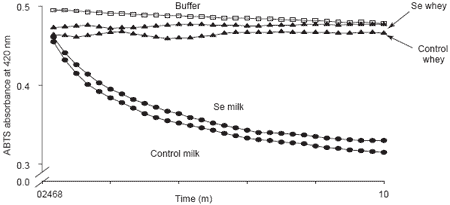 |
Figure 9. ABTS·+ radical quenching as a function of time after addition of milk or whey from Se-enriched milk or control milk. |
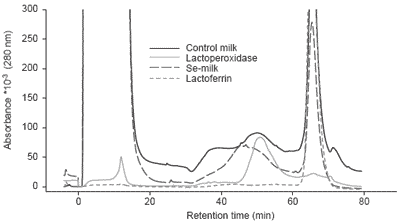 |
Figure 10. Chromatograms on SP-agarose. Flow-through and wash from zero to 30 min, then a gradient of 0.15-0.5 M NaCl from 30-80 min was applied. |
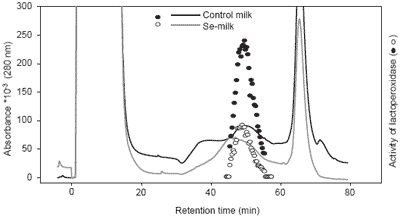 |
Figure 11.Chromatograms and measurements of lactoperoxidase activity. Activity measurements are superimposed onto chromatograms. |
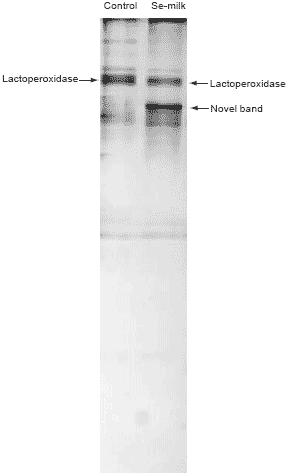 |
Figure 12.Silver-stained SDS-PAGE of peak lactoperoxidase fractions from control milk (left lane) or Se-milk (right lane). Authentic lactoperoxidase and the novel band seen only in Se-milk are indicated by arrows. |
Conclusions - Sel-Plex® selenium supplementation results in rapid appearance of selenium in milk.
- Milk selenium levels ten times higher than controls were achieved.
- Lipid oxidation induced by light is lower in Semilk compared to control milk, whereas protein oxidation is higher.
- Protein oxidation induced by hydrogen peroxide is much lower in Se-milk compared to control milk. This is apparently not due to a simple antioxidant effect of selenomethionine, but seems to reside with intrinsic properties of lactoperoxidase itself or associated proteins.
|
Acknowledgments
This work was supported by ØFORSK (Danish Science Ministry), the Danish Dairy Research Foundation (Danish Dairy Board) and Alltech Inc.
References
Assmann, A., K. Briviba and H. Sies. 1998. Reduction of methionine selenoxide to selenomethionine by glutathione. Arch. Biochem. Biphy. 349:201-203.
Finley, J.W., C. Ip, D.J. Lisk, C.D. Davis, K.J. Hintze and P.D. Whanger. 2001. Cancer-protective properties of high-selenium broccoli. J. Agric. Food Chem. 49:2679-2683.
Grimaud, R., B. Ezraty, J.K. Mitchell, D. Lafitte, C. Briand, P.J. Derrick and F. Barras. 2001. Repair of oxidized proteins. Identification of a new methionine sulfoxide reductase. J. Biol. Chem. 276:48915-48920.
Hemingway, R.G. 1999. The influences of dietary selenium and vitamin E intakes on milk somatic cell counts and mastitis in cows. Vet. Res. Commun. 23:481-499.
Jackson, M.J., C.S. Broome and F. McArdle. 2003. Marginal dietary selenium intakes in the UK: Are there functional consequences? J. Nutr. 133:1557S.
Klein, E.A., I.M. Thompson, S.M. Lippman, P.J. Goodman, D. Albanes, P.R. Taylor and C. Coltman. 2003. SELECT: the selenium and vitamin E cancer prevention trial. Urolog. Oncol. Semin. Orig. Invest. 21:59-65.
Kryukov, G.V., R.A. Kumar, A. Koc, Z. Sun and V.N. Gladyshev. 2002. Selenoprotein R is a zinc-containing stereo-specific methionine sulfoxide reductase. PNAS 99:4245.
Lacourciere, G.M., R.L. Levine and T.C. Stadtman. 2002. Direct detection of potential selenium delivery proteins by using an Escherichia coli strain unable to incorporate selenium from selenite into proteins. PNAS 99:9150.
Levine, R.L., L. Mosoni, B.S. Berlett and E.R. Stadtman. 1996. Methionine residues as endogenous antioxidants in proteins. Proc. Natl. Acad. Sci. USA 93:15036-15040.
McKenzie. R.C., S. Rafferty and G.J. Beckett. 1998. Selenium: an essential element for immune function. Immunol. Today 9:342-345.
Moskovitz, J., B.S. Berlett, J.M. Poston and E.R. Stadtman. 1997. The yeast peptide-methionine sulfoxide reductase functions as an antioxidant in vivo. Proc. Natl. Acad. Sci. USA 94:9585-9589.
Ortman K. and B. Pehrson. 1999. Effect of selenate as a feed supplement to dairy cows in comparison to selenite and selenium yeast. J. Anim Sci. 77:3365.
Rayman, M.P. 2000. The importance of selenium to human health. The Lancet 356:233-241.
Ruan, H., X.D. Tang, M.L. Chen, M.L. Joiner, G. Sun, N. Brot, H. Weissbach, S.H. Heinemann, L. Iverson, C.F. Wu and T. Hoshi. 2002. High-quality life extension by the enzyme peptide methionine sulfoxide reductase. PNAS 99:2748.
Smith, K.L., J.S. Hogan and W.P. Weiss. 1997. Dietary vitamin E and selenium affect mastitis and milk quality. J. Anim Sci. 75:1659.
Stadtman. E.R., J. Moskovitz, B.S. Berlett and R.L. Levine. 2002. Cyclic oxidation and reduction of protein methionine residues is an important antioxidant mechanism. Mol. Cell Biochem. 234-235:3-9.
Authors:JAN STAGSTED1, TIEN HOAC2, BJÖRN ÅKESSON2 and JACOB H. NIELSEN1
1 Department of Food Science, Research Center Foulum, Danish Institute of Agricultural Sciences, Tjele, Denmark
2 Biomedical Nutrition, Lund Institute of Technology, Lund University, Lund, Sweden