Sequencing of the bovine genome offers new opportunities to understand the biology of dairy cattle and provides the framework to identify the genetic basis for the improvements and animal differences in productive efficiency. The new areas of biomics era includes genomics (covering DNA), transcriptomics (RNA), proteomics (protein), metabolomics (metabolites) and systems biology (integrating all of these), with bioinformatics. Omics technologies help to design better dietary regimensmatching to genotype of the animal. Gene expression profiling using microarray technology is rapidly becoming an important tool for nutritional studies and for evaluating nutritional strategies.
Applications & Advances of Nutrigenomics in Animal nutrition
Nutrigenomics applies genomic technologies to study how nutrients affect expression of genes. The study of how genes and gene products interact with dietary chemicals to alter phenotype and, conversely, how genes and their products metabolize nutrients is called Nutritional genomics or Nutrigenomics (Kaput et al., 2005). Nutrigenetics focuses on how the genetic composition (i.e., genetic variation) of an animal influences their response to a given diet. Nutrigenomics studies will aid in formulating nutritionally appropriate diets that may be optimized for animals based on their genomic underpinnings. Individual gene markers may have variable response in individual animals receiving similar diets, hierarchical cluster analysis and pattern recognition methods are becoming tools for comparing nutritional responses with different diets. There are two areas where the developments in genomics are already affecting our understanding related to nutrition, feed efficiency and the biology of milk production.
Nutrigenomics applications in feed efficiency and nutrient utilization.
The use of residual feed intake as a selection tool identify cows with superior genetics for feed efficiency. Koch et al. (1963) first proposed the use of RFI as an attempt to partition feed intake into growth and maintenance components. Residual feed intake is defined as the difference between the actual feed intake of an animal and its expected feed requirements for maintenance and growth (Basarab et al., 2003). A significant improvement in profitability could be achieved through a reduction of production costs via implementation of selection strategies to improve feed efficiency with superior genetics , independent of growth rate. Genetic variation in maintenance energy requirements of cattle is moderately to highly heritable and, therefore, an opportunity to select for more efficient cattle may exist (Carstens et al., 1989). Genetic selection based on RFI can result in progeny that eat less without compromising growth performance (Richardson et al. 1998).
Nutrigenomics to understand energy balance in the lactating dairy cow.
Nutrigenomics describes how the amount and/or type of feed an animal receives, relative to its genetic and physiological requirements, affects its molecular phenotype, thus changing production, energy balance, reproduction, and health. The uncoupling of the somatotropic axis and the level and duration of this uncoupling can be influenced by diet. Factors that influence energy balance, such as nutrition and milking frequency, have different effects on the metabolism of the mammary gland, liver, and adipose tissue. For example, although once-daily milking and greater feeding levels improve energy balance, once-daily milking lowers milk production through reduced secretary cell activity and number, whereas, greater feeding levels increase milk production through the provision of more nutrients. These effects are independent and at least partially additive; if both strategies are used, the outcome is a combination of the two. Experimental results indicate that the molecular changes underpinning these effects can persist beyond the period of treatment. Less frequent milking results in an earlier recoupling of the somatotropic axis post-partum and adipose accretion, physiological changes that are also influenced by energy balance, but are dependent on feed type as well as availability. A greater understanding of the effects of nutrition on the molecular phenotype is required to optimize cow management for productivity and welfare.
Nutrigenomics to understand the effect of diet on metabolism
Nutrition affects metabolism homeostasis and metabolic pathways of different cell (Kardia 2000). Nutrients can directly act on gene expression as well as regulate gene expression by metabolites or hormones. Nutrient intake also entrains the daily rhythm of metabolism through regulation of a transcriptionally regulated biological clock. Although the exact nutrient is not clear, nutritional entrainment of the mammary gland clock has been demonstrated. In studies of steers under nutritional restriction due to intake of poor quality feeds, expression of specific genes associated with protein turnover, cytoskeletal remodeling, and metabolic homeostasis was clearly influenced by diet. These studies provide application of nutrigenomics to resolve the molecular markers important in nutrition research (Byrne et al., 2005). Understanding the mechanisms of bioactive nutrients has allowed development of targeted nutritional strategies and has great future potential as an experimental approach.
Nutrigenomics to understand milk fat depression
Milk fat depression is caused by specific intermediates of ruminal biohydrogenation of polyunsaturated fatty acids and is a clear example of nutritional regulation of metabolism. Advances in lipid analysis and chemistry allowed identification of the bioactive conjugated linoleic acid isomers, but nutrigenomic approaches were key to identification of mechanism. Thus, under certain dietary conditions rumen biohydrogenation is altered so that unique FA intermediates are produced and some of these are potent inhibitors of milk fat synthesis. To date three conjugated linoleic acid (CLA) isomers have been identify as bioactive FAs that inhibit milk fat synthesis – trans-10, cis-12 CLA, cis-10, trans-12 CLA and trans-9, cis-11 CLA. This inhibition involves a coordinated down regulation of gene expression for key enzymes in the synthesis of milk fat. This has been best studied for trans-10, cis-12 CLA and the cellular mechanism involves the SREBP1 transcription factor family. The genes for key enzymes in FA synthesis have a base sequence in their DNA code that is referred to as a SRE element. The SRE element binds the active fragment of SREBP1 thereby reducing gene expression (Bauman et al., 2011). Improving our understanding of the role that specific nutrients play in the regulation of gene expression and metabolism represents a developing area that should offer exciting new opportunities to improve productive efficiency of dairy cows.
Nutrigenomics to understand the animal performance (production/disease/aging process)
Healthy adult and senior animals given the same foods can be studied to identify the gene expression and biochemical differences characteristic of the aging process. Foods for senior animals can then be rationally designed and evaluated for their ability to modify gene expression profiles in animals to more closely reflect those found in healthy adult animals, which has the potential to improve health and quality of life. In some of the more classical studies, expression studies have allowed for an improved understanding of the physiological basis on the beneficial global effects of caloric restriction on aging (Lee et al., 2002) and the effects of minerals such as selenium on intestinal function (Rao et al., 2001). In poultry, gene expression studies have lead to a better understanding of disease resistance (Liu et al., 2003), and of growth and tissue differentiation. These tools have also been used to differentiate effects of specific nutrient forms.
Omics Technology in Nutrition Research
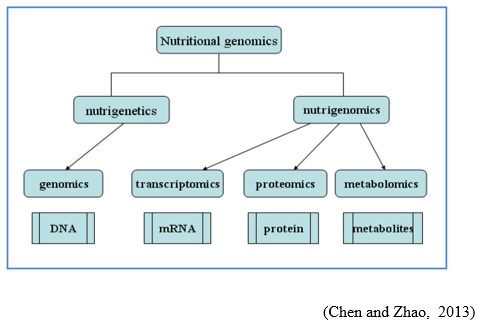
- DNA Chip Technology: Gene chip technology is mainly used to identify the sequence (gene or gene mutation) and gene expression level, such as nutrigenomics applications and impacts of dietary protein on gene expression. Gene chip technology related to the molecular mechanisms of some dietary bioactive components which supplies molecular basis for the accuracy and reasonableness of Dietary Reference Intakes (DRIs) through evaluating molecular biomarkers of nutritional status (Song 2009)
- mRNA Differential Display Technology: The basic principle of mRNA differential display (DD) method is to reversely transcript expressed mRNAs into the corresponding cDNAs in comparable cells under suitable condition. Macronutrients, vitamins, minerals, and various phytochemicals can modify gene transcription and translation, which can alter biological responses such as metabolism, cell growth, and differentiation. Gene expression (mRNA) is analysed in a biological sample using microarray technology. In dairy industry, an effective utilization of microarray technology was beneficial to study mammary gland tissues (milk production and udder health), muscle growth and development and myogenesis process (beef production) and the role of gut microflora on nutritional diet intake in ruminants (health and food safety).
- Proteomics Technology: Proteomics is the study of all the proteins in a particular cell, tissue or compartment. Proteomic analysis was quite effective and useful to evaluate the effect of dietary methionine on breast-meat accretion and protein expression in skeletal muscle of broiler chickens. The major tools of proteomics are two dimensional gel electrophoresis and mass spectrometry.
- Metabolomics Technology: Metabolomics provides an advanced technology for nutrition research which is now widely used in the field of multiple nutritional research (He et al., 2011). For example, biomarkers could be used to measure the metabolic effects to the external environment in the body or early disease detection (Walsh et al., 2008). Related application of Metabolomics in nutrigenomics displays the characteristic on understanding their health benefits through the intake of functional complexes. He et al. (2009) studied arginine requirement of pigs using NMR-based metabolomics technology and found that the increasing of dietary arginine could significantly improve the growth performance of pigs and cause metabolism variation. As in the case of transcriptomics and proteomics, the scope of metabolomic analysis is mainly restricted to the assessment of the influence of dietary components on the metabolome of selected organs or tissue in animal nutrition studies.
Future prospects in nutritional research through nutrigenomics
In the next decade these “omics” technologies will redefine the criteria for evaluating nutrient requirements and can focus on the ability of dietary components to meet physiological needs rather than on specific requirements for key nutrients. Nutrigeonmics will be a path breaking tool through identification of pathways and candidate genes responsible for dietary induced diseases and ultimately reduction in production losses due to these diseases in animals. The discoveries of gene markers related to economically important traits like milk, meat, wool production etc whose expression can be improved by dietary regimens is a need of today’s nutrigenomic research which will help for sustainable livestock production. It will provide methods and specific markers for rapidly evaluating the nutritional status of individual and groups of animals. It will help us define the hidden effects of specific nutrients and nutrient interactions. Nutrigenomics also aims to demonstrate the effect of bioactive food compounds on health, which should lead to the development of functional foods that will develop and maintain health according to the needs of the individual.
References:
Basarab, J. A., Price, M. A., Aalhus, J.L., Okine, E.K., Snelling, W.M. and Lyle, K.L. 2003. Residual feed intake and body composition in young growing cattle. Can. J. Anim. Sci. 83:189-204.
Bauman, D. E., Harvatine, K. J and Lock, A. L. 2011. Nutrigenomics, rumen-derived bioactive fatty acids and the regulation of milk fat synthesis. Annu. Rev. Nutr. 31:299-319.
Byrne, K. A., Wang, Y. H., Lehnert, S. A. 2005. Gene expression profiling of muscle tissue in Brahman steers during nutritional restriction. J Anim Sci. 83:1-12.
Carstens, G.E., Johnson, D.E., Johnson, K.A., Hotovy, S.K. and Szymanski, T.J. 1989. Genetic variation in energy expenditures of monzygous twin beef cattle at 9 and 20 months of age. Energy Metabolism of Farm Animals. EAPP Publ. Pudoc, Wageningen, The Netherlands. Page 312.
Chen, L and Zhao. 2013. Advances of nutrigenomics: concept, content, technology and development. Experimental Biology and Agricultural Sciences. Volume–1(3) http://www.jebas.org
Ghormade, V., Khare A., Baghel, R. P. 2011. Nutrigenomics and its Applications in Animal Science . Veterinary Research Forum. Volume 2 No: 3: 47 - 155
He, Q., Ren, P., Wang, Y. 2011. Applications of Metabolomics in Nutrition Research: A Review. Food Science :32.
Kaput, J., Ordovas, J. M., Ferguson, L. 2005. The case for strategic international alliances to harness nutritional genomics for public and personal health. Brit J Nutr . 94:623-32.
Kardia, S. L. 2000. Context-dependent genetic effects in hypertension. Current Hypertension Reports 2: 32-38.
Koch, R. M., L. A. Swinger, D. Chambers and K. E. Gregory. 1963. Efficiency of feed use in beef cattle. J. Anim. Sci. 22:486-494.
Lee, C. K.., Allison, D, B., Brand, J., Weindruch, R and Prolla, T. A. 2002. Transcriptional profiles associated with aging and middle age-onset caloric restriction in the mouse heart. PNAS. 99:14988-14993 (www.pnas.org/cgi/doi/10.1073/pnas.232308999)
Liu, H.C., H.H. Cheng, V. Tirunagaru, L. Sofer, and J. Burnside. 2001. A strategy to identify positional candidate genes conferring Marek’s disease resistance by integrating DNS microarrays and genetic mapping. Anim. Genet. 32:351-359.
Rao, L., B. Puschner and Prolla, T.A. 2001. Gene expression profiling of low selenium status in the mouse intestine: Transcriptional activation of genes linked to DNA damage, cell cycle control and oxidative stress. J. Nutr. 131: 3175-3181.
Richardson, E.C., Herd, R.M., Archer, J.A., Woodgate, R.T. and Arthur, P.F. 1998. Steers bred for improved net feed efficiency eat less for the same feedlot performance. Aust. Soc. Anim. Prod. 22:213-216.
Song , Y. 2009. Advanceds in Nutrigenomics. Chinese Journal of Animal Science: 21: 020.
Walsh, M., Nugent, A., Brennan, L and Gibney, M. 2008. Understanding the metabolome–challenges for metabolomics. Nutrition Bulletin 33: 316-323.