1. Introduction
The intestinal epithelium is a major interface with the outside world. This interface is separated from the body’s internal milieu by a single layer of epithelial cells consisting of absorptive enterocytes, goblet, enteroendocrine and Paneth cells. These cells are exposed, at the apical domain, to an external environment that is continuously changing by types and amounts of microorganisms, microbial products, gastrointestinal secretions and potentially toxic chemicals. The intestinal epithelium constantly monitors (senses) the composition of its luminal contents in order to defend threats to its integrity.
Enteroendocrine cells (EECs), dispersed amongst the cells lining the intestinal epithelium are pivotal to the chemosensing pathways of the intestinal tract. They are flask-shaped, with the majority having long slender apical projections that are in direct contact with the gut lumen, where they can sense the luminal contents. They respond to changes in luminal contents by releasing hormones. There are at least twelve discrete cell types that make up the enteroendocrine family and collectively they produce over twenty different hormones that act locally, centrally or in the periphery [1e3].
The intestine contains more than 1000 different species of bacteria [4] and must discriminate between pathogenic and commensal bacteria in order to maintain a balance between immune protection and inflammatory over-reaction. Toll-like receptors (TLRs), a class of proteins known as pattern recognition receptors, play a key role in the recognition of microbes via detection of specific and conserved microbial molecular features [5,6]. They are present on an array of immune cells including antigen presenting cells, macrophages and dendritic cells as well as the basolateral membrane of some columnar epithelial cells [6]. To date 10 TLRs have been identified in humans and 13 in mice, detecting certain molecular structures that are conserved in bacteria [7]. TLRs that respond to bacterial products include TLR2, TLR4, TLR5 and TLR9, with their respective ligands being bacterial peptidoglycan, lipopolysaccharide, flagellin and DNA containing unmethylated CpG dinucleotide motifs [8], the latter relatively common in microbial genomes, but highly methylated and uncommon in vertebrate genomes [9]. The immune system recognizes the presence of any of these molecular structures activating appropriate defense pathways to resist infection. In terms of TLR9, the most stimulatory of these CpG motifs are hexamers with the sequence RR/CG/YY (R = G or A; Y = C or T) [10]. Since intestinal pathogens often differ from commensals by just the presence (or absence) of a few sets of genes i.e. pathogenicity islands [11], or “black holes” [12], it is sensible that TLRs recognize products common to both pathogens and intestinal commensal microbes [13].
Little is known about sensing of bacteria by enteroendocrine cells of the intestine. The objective of this study was to identify potential mechanism(s) by which enteroendocrine cells of the intestine may recognize bacteria.
2. Materials and methods
2.1. Tissues and species’ source
Pigs: Male and female Gloucestershire Old Spot piglets aged 40 d were housed in standard pens in a room with continuous heating (26.7 °C/80 °F) and 12 h light and dark cycle. All animals had free access to water and feed (commercial swine diet, Target Feeds Ltd, Whitchurch, UK). They were sacrificed with an intravenous injection of pentobarbitone (200 mg/ml, Pentoject, AnimalCare Ltd, York, UK) to the cranial vena cava (in line with the UK Home Office schedule 1 regulations). Immediately, sections of the intestine, proximal, mid and distal, were removed, rinsed in icecold saline, fixed in 4% neutral buffered formalin (NBF, Leica, Milton Keynes, Buckinghamshire, UK) for 4 h at 4 °C before storing in 20% sucrose (Fluka, Gillingham, Dorset, UK) in PBS at 4 °C.
Mice: Male and female C57BL/6 mice aged 8 weeks had access to water and food (standard chow, energy 3.60 kcal/g; Special Diet Services, Witham, Essex, UK) and were housed in standard tube cages with automatically controlled temperature and humidity and a 12:12 h light-dark cycle. They were killed by cervical dislocation in accordance with the UK Animals (Scientific Procedures) Act, 1986 and with guidelines set out by the University of Liverpool Ethics Committee and in line with UK Home Office schedule 1 regulations. The small intestine was immediately removed, rinsed in ice-cold saline and proximal (duodenum) section was fixed and treated as above
Human: Human duodenal biopsies (2nd part of duodenum) were obtained, with prior consent of patients and approval of Research Ethics Committee (MREC Ref: 02/11/228/A &2K/128) from male and female lean subjects undergoing endoscopy for routine gastrointestinal investigations, as described before [14]. After removal, histologically normal biopsies were fixed in 4% NBF pH 7.4 at room temperature for 2 h and then immersed in 20% sucrose/PBS at 4 °C.
2.2. Immunohistochemistry
Fixed tissue samples were embedded in gelatin blocks frozen and sectioned at a thickness of 10 μm on a cryostat (Leica, CM 1900UV-1-1), mounted on polysine-coated slides (and air-dried before storing at −80 °C, as described previously [15]. Slides containing human and mouse tissue sections were brought to room temperature and washed for 3 x 5 min each in PBS to remove unbound fixative. Sections of pig intestine were subjected to heat treatment for 10 min at 60 °C, rinsed 3 times in PBS, permeabilized in a gradient of alcohol. Immunohistochemistry was then performed on all tissue sections as previously described [3,15] with primary antibodies to TLR9 (H-100): Sc-25468, CCK (C-20): Sc21617 and chromogranin A (E20): sc-18232 (Santa Cruz Biotechnology, INC., Santa Cruz, CA, USA) diluted 1:100. Secondary antibodies, Cy3-conjugated IgG/IgY and Fluorescein isothiocyanate (FITC)-conjugated IgG/IgY (Stratech, Scientific Limited, Suffolk, UK), diluted 1:500, were then used. Sections mounted with Vectashield Hard Set Mounting Medium with 4′, 6-diaminido-2-phenylindole, DAPI (Vector Laboratories Ltd, Peterborough, UK) were visualized using an epifluorescence microscope (Nikon, Kingston upon Thames, Surrey, UK) and images were captured with a Hamamatsu digital camera (C4742-96-12G04, Hamamatsu Photonics K.K, Hamamatsu City, Japan). Omission of primary antibody was routinely used as a control.
2.3. Cell culture
STC-1 mouse enteroendocrine cells were maintained by serial passage in DMEM containing 10% fetal bovine serum, 2 mM L-glutamine, 100 μg/ml streptomycin and 100 U/ml penicillin as described before [16].
2.4. Measurements of CCK secretion
STC-1 cells were grown to 80%e90% confluence in 24-well plates before incubation at 37 °C in assay media (HBSS containing 20 mM HEPES-1% FBS [pH 7.4]) [16] supplemented with either CpGODN1668 (InvivoGen, Toulouse, France) with/without TLR9 inhibitors, or heat-killed bacterial cells for 4 h. Control cells were maintained simultaneously in assay media alone. For TLR9 inhibition experiments, cells were pre-incubated with inhibitor compounds, Hydroxychloroquine (Fisher Scientific, Loughborough, UK) or E6446 (Merck, Darmstadt, Germany), in DMEM for 1 h before addition of CpG-ODN1668 (±inhibitor) in assay media. For assessment of bacterial-induced CCK secretion, overnight cultures of Lactobacillus amylovorus GRL1112, Escherichia coli O55/H7, Shigella flexneri 2457T and Salmonella typhimurium ST4/74 were adjusted to 108 cells/ml in assay media and heat-killed at 80 °C for 30 min.
After 4 h, assay media were collected, centrifuged to remove cell debris, and stored at 80 °C until use. CCK concentrations were measured using a commercially available enzyme immunoassay (EIA) kit (Phoenix Pharmaceuticals Inc., Burlingame, USA) according to the manufacturer’s instructions. Standard curves were constructed using GraphPad Prism 8 (GraphPad Software Inc., La Jolla, USA).
2.5. Statistical analysis
Data are presented as mean ± SEM. Significance of differences was determined using one-way ANOVA with Tukey’s multiple comparison test (GraphPad Prism 8). Results were considered significant if P < 0.05.
3. Results and discussion
Expression of TLRs in the intestine. Using immunohistochemistry, we assessed expression of TLRs 2, 4, 5 and 9, that directly recognize bacterial components, along the length of porcine intestinal tract and showed that TLRs were mainly expressed in immune cells, lymphocytes, submucosal plexus and in basolateral membrane of enterocytes in the distal intestine (data not shown). Of note, TLR9 was also expressed in EECs of the proximal intestine (duodenum). By immunohistochemistry we showed 8% of chromogranin A-positive cells (a classical marker of EECs) also contained TLR9 (Fig. 1A).
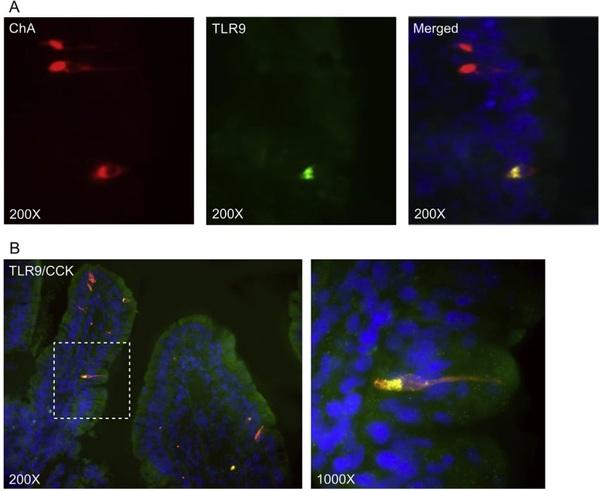
Fig. 1. Immunohistochemical co-localization of TLR9 in porcine enteroendocrine cells with CCK. Sections of porcine duodenal tissue were probed with: (A) antibodies to TLR9 (green) and enteroendocrine marker chromogranin A (ChA, red), and (B) antibodies to TLR9 (green) and CCK (red). (A) The merged images (yellow) indicate expression of TLR9 in ChA-containing cells. (B) Merged images of TLR9 and CCK (yellow), demonstrate co-expression. Images are 200X and 1000X magnified. Nuclei are stained with DAPI (blue).
Having demonstrated that TLR9 resides in EECs of the proximal intestine, we sought to determine which type of EECs express TLR9. Traditionally it has been accepted that discrete cell types that make up the EEC family have a distinct hormonal profile and localization along the length of the gut [1]. However, recent investigations have shown that the ‘one hormone, one cell’ is not necessarily correct, and that there are more complex patterns of gut hormone colocalization in EECs. In general, CCK is released by I-cells, secretin by S cells and glucose-dependent insulinotropic peptide (GIP) by K cells, primarily located in the proximal small intestine. Peptide YY (PYY) and glucagon-like peptide 1 and 2 (GLP-1 and GLP-2) are secreted predominantly by L-cells residing mostly in the distal gut [17]. However, some L-cells have also been identified in the duodenum, with higher numbers observed in jejunum and ileum [18]. Enterochromaffin cells (EC), a subtype of EEC, secrete serotonin.
Using immunohistochemistry, we showed that in porcine duodenum TLR9 was expressed with CCK-containing cells (Fig. 1B).
We further observed that 38% of all EECs in porcine duodenum contained CCK, 8% contained TLR9 and 97% of TLR9-positive cells also possessed CCK. The number of duodenal EECs expressing PYY, GLP-1 and GLP-2 was much lower (8%, 2% and 1% respectively), but it was notable that 38% of TLR9-positive cells also contained the satiety hormone PYY. There was no expression of TLR9 in EECs possessing secretin, GIP, or serotonin (see Appendix A supplementary data).
Fig. 2. Immunohistochemical localization of TLR9 and CCK in human and mouse duodena. Sections of human (top panels) and mouse (bottom panels) duodenal tissues were probed with antibodies to TLR9 (red) and CCK (green). In top and lower panels, the overlay images of TLR9 and CCK (yellow) show co-expression in the same cell. Images are 200X magnified.
Fig. 3. Release of CCK by STC-1 cells in response to 5 μM CpG ODN1668 (a specific ligand for mouse TLR9) in the presence or absence of 3 μM hydroxychloroquine or 0.1 μM E6446 (specific inhibitors of TLR9). Results show 65-70% reduction in CpG ODN1668-induced CCK release in the presence of TLR9 inhibitors. Data are shown as % of control (±SEM). A one-way ANOVA with Tukey’s multiple comparison post-test was used to determine statistical significance where **p < 0.01.
Fig. 4. CCK secretion by STC-1 cells in response to bacterial exposure. STC-1 cells were exposed to the heat-inactivated pathogenic Escherichia coli O55/H7, Shigella flexneri 2457T, Salmonella typhimurium ST4/74, and non-pathogenic Lactobacillus amylovorus GRL1112 (108 cells/ml) for 4 h. Supernatants were collected and amount of CCK secreted was measured using a commercial enzyme immunoassay kit. Results are shown as % of control (±SEM).
To determine if the profile of TLR9 with CCK co-expression also applies to other species, we further demonstrated, using immunohistochemistry, that TLR9 and CCK also co-localize in duodenal EECs of human and mouse intestine (Fig. 2).
CCK plays a variety of roles in digestive processes, such as slowing gastric emptying, mediating intestinal motility, and stimulating pancreatic and gall bladder secretion [19]. It also inhibits food intake in a manner consistent with a role in satiety [20]. However, CCK if secreted in excess, leads to onset of emesis (vomiting) [21].
Having shown that proximal intestine of various species expresses TLR9 and CCK, and that they reside in the same endocrine cells, we next examined the responsiveness of STC-1 cells, a murine model of EEC, to the agonist of TLR9, unmethylated CpG oligonucleotide, in evoking CCK release. STC-1 cells express CCK and secrete the biologically active form of the peptide, CCK-8 [16]. Using PCR and immunohistochemistry, we also demonstrated that STC1 cells express both TLR9 mRNA and protein (see Appendix B supplementary data).
Exposure of STC-1 cells to 5 μM CpG ODN1668 (a specific ligand for mouse TLR9) showed 2.1-fold increase in CCK release compared to untreated cells (Fig. 3). This increase in CCK secretion was reduced by 60-70% in the presence of 3 μM hydroxychloroquine or 0.1 μM E6446, specific inhibitors of TLR9 [22,23] (Fig. 3), supporting that CpG DNA activation of TLR9 induces CCK secretion.
To show direct effects of bacteria on CCK secretion, STC-1 cells were incubated with heat-inactivated pathogenic Escherichia coli O55/H7, Shigella flexneri 2457T, Salmonella typhimurium ST4/74, and non-pathogenic Lactobacillus amylovorus GRL1112. In all cases there was an increase in CCK secretion compared to untreated control. However, levels of CCK release were higher in response to pathogenic bacteria and lowest in response to the non-pathogenic Lactobacillus (Fig. 4).
We have determined that the pathogenic strains we have used to induce CCK secretion by STC-1 cells (E. coli O55/H7; S. flexneri 2457T; S. typhimurium ST4/74), not only have substantially bigger genomes than the non-pathogenic L amylovorus, they also have significantly higher numbers/frequency of RR/CG/YY stimulatory CpG hexamers in their genomic DNA (Table 1). It is tempting to propose that there is an association between numbers/frequency of stimulatory CpG hexamers, activation of TLR9 and amount of CCK released. Physiologically, pathogen-induced excessive secretion of a gut hormone, such as CCK, provoking emesis can serve as a protective mechanism against development of enteric infections.
The sensing receptors that face the lumen of the intestine and are responsive to luminal contents provide unique therapeutic opportunities. Targeting of these receptors with agonists/antagonists has the potential to provide gut-directed therapies.
This article was originally published in Biochemical and Biophysical Research Communications, Volume 525, Issue 4, 14 May 2020, Pages 936-940. https://doi.org/10.1016/j.bbrc.2020.02.163. This is an Open Access article under the CC BY license (http://creativecommons.org/licenses/by/4.0/).