INTRODUCTION
Mycotoxins are substances that occur naturally in the environment (1, 2). In addition to mycoestrogens such as zearalenone (3, 4), selected trichothecenes, including DON and/or its metabolites (but in vitro), can affect steroidogenesis (2) and changes in gene expression, which suggests that these compounds could disrupt hormonal homeostasis (1).
Deoxynivalenol (DON), a polar organic compound produced mainly by Fusarium graminearum and Fusarium culmorum (5), is one of the most economically important mycotoxins in the world (6). The presence of a ketone group at position C8 is characteristic of B trichothecenes. Deoxynivalenol also contains an epoxide group which enables the mycotoxin to bind to a large number of eukaryotic ribosome subunits and disrupt the activity of peptide transferase, thus compromising the elongation or shortening of peptide chains (7). Deoxynivalenol affects the cellular transport rate and enzyme metabolism in the cytoplasm (8), it leads to changes in affinity for active binding sites and disrupts protein synthesis (2).
There is a general scarcity of in vitro and, in particular, in vivo evidence that DON can directly trigger the overexpression of steroid hormone receptors or induce hormonal activity. However, DON affects steroidogenesis (2) and changes in gene expression (9), which suggests that it can potentially disrupt the functioning of the hormonal system. The available background knowledge indicates that estrogen receptors (ER) are essential for various physiological processes, including the regulation of the endocrine system, lipid profile, bone integrity, hemostasis and endothelial function. Estrogen receptors are inflammatory markers, and they promote the growth of various tissues, and contribute to pre-natal and post-natal development (10). Estrogen receptors are divided into two subtypes, ERα and ERβ, which have different identity (11) and interact with different tissues. For example, ERα is more prevalent in the gonads, mammary glands, kidneys and bronchi, and it stimulates proliferative processes in cells. In turn, ERβ is predominant in bones and pulmonary alveoli, and it delivers antiproliferative effects (12, 13). Unlike other steroid receptors, ERs bind to a wide range of exogeneous compounds, and are they are increasingly often classified as promiscuous receptors (14). Ligand recognition and receptor-ligand binding are regarded as highly specific biological processes that activate the appropriate receptors and trigger specific responses to stimuli in vivo. It is believed that protein-ligand recognition relies on a lockand-key model and an improved induced-fit model. Estrogen receptors can bind to various ligands due to their unique ability to associate ligands and detect binding cavities. They appear to be an exception to the core concept of lock and key, and they do not discriminate between the bound ligands. It remains unknown whether the above mechanism also applies to non steroid compounds. Estrogen receptors have evolved to play different tissue-dependent biological roles (15).
The fact that DON is not involved in phase I biotransformation reactions (16), and P450 isoenzymes, in particular CYP1A1, participate in the metabolism of many structurally different endogenous and exogenous compounds, especially the hydroxylation of estrogens (17), in distal segments of the intestines, had to be considered in the present research. Nandekar et al. (18) suggested that the CYP1A1 gene in macroorganisms undergoes transcriptional activation under exposure to environmental contaminants (such as DON), in particular substrates of the CYP1A1 enzyme. Recent in vitro studies have demonstrated that CYP1A1 can also act as a detoxifying enzyme (9).
Glutathione S-transferase (GST), also known as ligandin, is a phase II biotransformation enzyme and a biomarker of plant contamination (19) with undesirable substances such as mycotoxins (9). Glutathione S-transferase isoenzymes are generally overexpressed under exposure to substances which modulate apoptotic processes and induce the expression of biologically active proteins, as well as during detoxification processes (20).
The above problems were considered in the process of formulating the research objective. This study set out to determine whether DON can influence the expression of ER and genes encoding selected large intestine enzymes which participate in the detoxification of mycotoxins. A pioneering in vivo study was conducted to verify the research hypothesis. The presented research is innovative because it aimed to determine whether the presence of a NOAEL dose of DON, which is not a contributing factor to clinical mycotoxicosis, can induce subclinical states such as steroidogenesis dysfunction or physiological detoxification. The aim of this study was to determine in vivo whether a low dose of DON administered per os for 42 days can affect the immunohistochemical expression of ER in different intestinal segments on different dates of exposure or the expression of genes (CYP1A1 and GSTP1) encoding selected enzymes in the colon of pre-pubertal gilts.
MATERIALS AND METHODS
Experimental Animals
The experiment, conducted at the Department of Veterinary Prevention and Feed Hygiene, Faculty of Veterinary Medicine (University of Warmia and Mazury in Olsztyn, Poland), involved 36 pre-pubertal gilts. The animals were clinically healthy, and had initial BW of 25 ± 2 kg. They were kept in group pens, with free access to water. The feed offered to gilts had been tested for the presence of the following mycotoxins: DON, ZEN and α ZEL. Mycotoxin concentrations were determined by separation techniques, using immunoaffinity columns (DON-TestTM DON Testing System, VICAM, Watertown, USA; Zearala-TestTM Zearalenone Testing System, G1012, VICAM, Watertown, USA) and HPLC (Hewlett Packard, type 1,050 and 1,100) (21) with fluorescence and/or UV detection [limit of detection: 1.0 ng/g for DON–(22); 3.0 ng/g for ZEN–(23)].
Experimental Design
The animals were divided into an exposed group (E = DON; n = 18) and a control group (C, n = 18) (24). Group E gilts were administered DON per os at 12 µg/kg BW – this value was consistent with the limits set by EFSA (25) and the NOAEL dose when the experiment was planned. Group C animals received a placebo. Deoxynivalenol was administered daily, before morning feeding, in gastro-soluble gel capsules, with feed as the carrier. The animals in group C received the same gel capsules, but without the mycotoxin.
The analyzed mycotoxin (DON) was biosynthesized, purified and standardized at the Department of Chemistry, Poznan University of Life Sciences (Poland). The experiment lasted for 42 days. The DON dose was adjusted to the BW of pre pubertal gilts. In order to prevent uneven feed intake, DON was administered in capsules Deoxynivalenol samples were diluted in 500 µL of 96% ethyl alcohol (SWW 2442-90, Polskie Odczynniki Chemiczne SA, Poland) to achieve the required dose (based on the BW of animals). Final solutions were stored for 12 h at room temperature to evaporate the solvent. During the adjustment period, the animals were weighed at 7-day intervals. Three gilts from the exposed and control groups (a total of six animals) were euthanized by intravenous administration of pentobarbital sodium (Fatro, Ozzano Emilia BO, Italy) and bleeding on experimental days 7 (week I), 14 (week II), 21 (week III), 28 (week IV), 35 (week V) and 42 (week VI). Immediately after cardiac arrest, sections of intestinal tissues were collected for analyses.
Reagents
Deoxynivalenol
Deoxynivalenol was biosynthesized at the Department of Chemistry, Poznan University of Life Sciences (Poland), in accordance with a previously described procedure (26, 27).
Chemicals and Apparatus
A chromatographic analysis of DON was conducted at the Department of Chemistry, Poznan University of Life Sciences (Poland), in accordance with a previously described procedure (22).
Immunohistochemistry
Tissue Samples
Every week, post-mortem samples of porcine gastrointestinal tissues were rinsed with phosphate buffer. Cross-sectional tissue samples (measuring ∼1 × 1.5 cm, two samples from each examined tissue) were obtained from: the duodenum – the first part (duodenal cap) and the horizontal or third part; jejunum and ileum – middle parts; cecum – 1 cm from the ileocecal valve; and the colon – middle parts of the ascending colon and descending colon.
Location of ERα and ERβ
The variability in ER expression was assessed in intestinal epithelial cells. Tissue specimens were fixed in 4% paraformaldehyde solution and embedded in paraffin. The negative control sections were incubated with normal horse serum instead of the primary antibody and were processed together with the evaluated sections. The ovaries of multiparous pigs were used as a positive tissue control for the applied ERα or ERβ antibodies. The analytical procedure has been described previously (28).
Optical Density (Scanning) of Stained Slides
The expression of ERα and ERβ in the intestinal samples collected from gilts in both groups was analyzed in scanned slides (Pannoramic MIDI Scanner, 3DHISTECH, Budapest, Hungary) with the use of NuclearQuant software (3DHISTECH, Hungary) (see Figures 1, 2). The slides were converted into digital images, and nuclear immunoreactivity was evaluated. The nucleus detection profile was as follows: radius – 1.50-2.10µm, minimum nuclear area – 0.9µm, minimum circularity – 3, smoothness – 1. ERα and ERβ expression was evaluated on a 4- point scale: negative = 0 (0 points), weak and homogeneous = + (1 point), mild or moderate and homogeneous = ++ (2 points), intense or strong and homogeneous = +++ (3 points). Staining intensity was evaluated on the following scale: from 0 – none of the below, + average intensity < 190 (CD BrownInt), ++ average intensity < 170 (CD BrownInt), + + + average intensity < 100 (CD BrownInt) (29). The results were presented in terms of the average percentage of cells expressing ERα and ERβ. The presence of ER proteins was determined based on the value of PER which represents the average number of enterocytes showing nuclear expression of ER proteins in 10 fields of view for at least 1,000 cells. The analysis of optical density and the nuclear detection profile used was performed by the authors, and the results had been previously published in several papers (9, 28).
The results of immunohistochemical examinations of the expression of ERα and ERβ in the gastrointestinal tract of pre pubertal gilts from both groups were analyzed statistically in two analytical approaches. In the first approach, the expression of both ER was analyzed statistically and compared between groups. In the second approach, the influence of sampling date (duration of exposure) on ER expression in each group and in a given intestinal segment was statistically analyzed. In both analytical approaches and in both groups, the effects of the 6- week exposure to DON on the expression of selected ER was evaluated in selected segments of the digestive tract on a 4-point scale (negative = 0 points; weak and homogeneous = 1 point; mild or moderate and homogeneous = 2 points; intense or strong and homogeneous = 3 points).
Expression of CYP1A1 and GSTP1 Genes
Collection and Storage of Samples for RNA
Extraction
Segments of the ascending colon and descending colon were collected immediately after cardiac arrest. The specimens were stored in RNAlater solution (Sigma-Aldrich; Germany), in accordance with the manufacturer’s instructions. The samples were collected on the same days of slaughter.
Total RNA Extraction and cDNA Synthesis
The total RNA was extracted (∼20 mg per sample tissue; n = 3 in each group), and purified using a Total RNA Mini Isolation Kit (A&A Biotechnology; Poland). The final RNA concentration and purity was determined using a UV-Vis spectrophotometer (BioPhotometer, Eppendorf; Germany), obtaining A260/280 ratios between 1.70 and 1.86 in all the samples. Next, the samples were incubated with DNase I (Roche Diagnostics; Germany), and then used to synthesize cDNA using a RevertAidTM First Strand cDNA synthesis kit (Fermentas; Lithuania). Briefly, the reaction contained 1 µg of the DNAse-treated total RNA and 5µM of oligo(dT)18 primer. After incubation at 65°C for 5 min, the samples were chilled on ice, and the following reagents were added in: 4 µL of 5× Reaction Buffer, 20 U of RiboLockTM RNAse Inhibitor, 1 mM of dNTP mix, and 200 U of RevertAid M-MuLV Reverse Transcriptase. The reaction was carried out at 42°C for 60 min, followed by heat-inactivation at 70°C for 5 min. Synthesized cDNA samples were stored at −80°C until used.
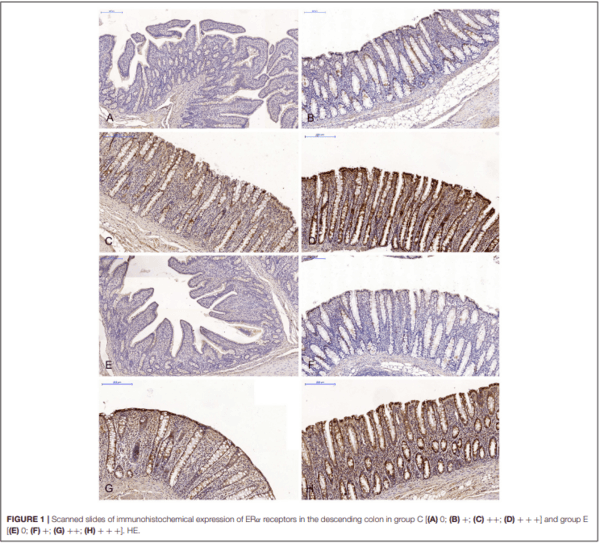
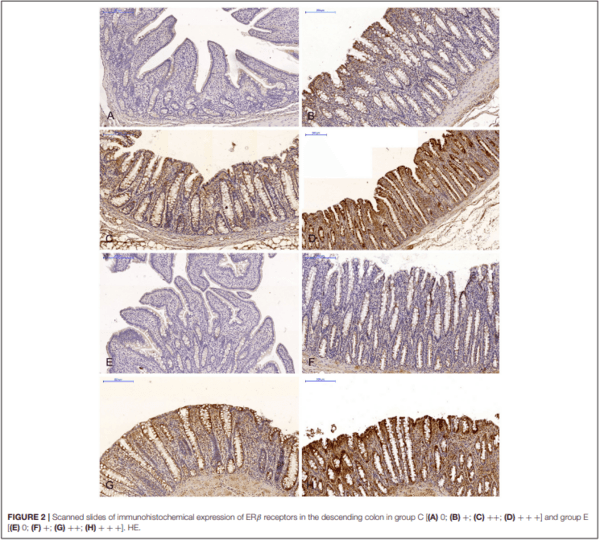
Statistical Analysis
The activity of ERα and ERβ in the digestive tract of gilts, and the expression of CYP1A1 and GSTP1 genes in the ascending colon and the descending colon were presented as geometric mean values (±) SD for each group, relative to control group from the beginning of the experiment (control 0d). The results were analyzed using Statistica software (StatSoft Inc., USA). Mean values in the control and exposed groups were compared by repeated measures one-way ANOVA based on the DON dose administered to pre-pubertal gilts. If differences were noted between groups, Tukey’s post-hoc test was used to determine which pairs of group means were significantly different. In ANOVA, group samples are drawn from normally distributed populations characterized by the same variance. Since the above assumptions were not met in all cases, the equality of group means was tested using the Kruskal-Wallis test of ranks and the multiple comparisons test in ANOVA. Non-parametric tests were used to test for differences in samples.
RESULTS
The experimental diets did not contain mycotoxins or their mycotoxin concentrations were below the sensitivity of the method (VBS, values below the sensitivity of the method). Clinical signs of DON mycotoxicosis were not observed during the experiment. The levels of modified or masked mycotoxins were not determined in the study. All laboratory and statistical analyses were carried out in 2013.
In the first analytical approach, the immunohistochemical expression of ERα and ERβ in each segment of the porcine gastrointestinal tract (where the samples were collected) was compared in all (six) weeks of exposure, between the control group (C) and the experimental group (E). In this analytical approach, ER expression was presented graphically (refer to Figures 3–6). The results were not presented graphically for the segments of the intestinal tract where statistical differences were not found (refer to Supplementary Figures 1–4).
TABLE 1 | Real-time PCR primers used in this study.
In this analytical approach, no statistical differences in the immunohistochemical expression of ERα not shown were observed in weeks I and VI (see Supplementary Figures 1A,B). In week II (see Figure 3A), statistical differences in the immunohistochemical expression of ERα were noted in the duodenal cap and the jejunum, in week III (see Figure 3B), differences were found in the third part of duodenum, in week IV (see Figure 3C), differences were noted in the ascending colon, and descending colon in week V (see Figure 3D), differences were observed in the ascending colon.
The levels of ERα expression in different tissues and in different weeks of exposure were also presented graphically (see Figure 4 and Supplementary Figure 2).
The average percentage of cells expressing ERα was similar in both groups. The highest average percentage of cells expressing ERα was noted in unstained cells (0 points), in particular in group C, whereas the lowest average percentage of cells expressing ERα was observed in the strongly stained cells (3 points) in group C. In group E, the average percentage of unstained cells (0 points) was lower, whereas the average percentage of stained cells (1– 3 points) was generally higher. These results indicate that the average percentage of strongly stained cells (3 points) expressing ERα increased steadily during exposure to DON.
In the same analytical approach (see Figure 5), differences in the immunohistochemical expression of ERβ were noted (at ∗P ≤ 0.05) in week II (see Figure 5A) in the duodenal cap, jejunum and the descending colon, and in the descending colon, and in week III (see Figure 5B), differences were found only in the descending colon. No significant differences in the immunohistochemical expression of ERβ were noted in weeks I, IV, V, or VI (see Supplementary Figures 3A–D).
Similarly, the levels of ERβ expression were presented in Figure 6 and Supplementary Figure 4. In general, no statistical differences in ERβ expression were found between the groups.
The average percentage of cells expressing ERβ was similar in both groups. ERβ expression continued to increase in intensely stained cells (3 points) on successive days of exposure to DON in both groups. In group E, a considerable increase in ERβ expression was observed in the duodenal cap and the third part of the duodenum (see Figure 6B).
In the second analytical approach (evaluating the influence of exposure time on ER expression), ER expression was presented only graphically due to a large number of significant differences (refer to Figures 7, 8). The results were not presented graphically for the segments of the intestinal tract where significant differences were not found (refer to Supplementary Figures 5, 6).
In this analytical approach, no significant differences in the immunohistochemical expression of ERα were found in the descending colon in group C and in the cecum and the descending colon in group E.
An analysis of the results presented in Supplementary Figure 5 indicates that the immunohistochemical expression of ERα continued to decrease in unstained (negative and homogeneous, 0 points) cells and in weakly stained and homogeneous cells (1 point) on successive sampling dates and in successive intestinal segments. An increase in ERα expression in intestinal cells [moderately (2 points) and strongly (3 points) stained cells] appears to be a normal process in pre-pubertal gilts.
In group E (Figure 7), the percentage of unstained (negative and homogeneous, 0 points) cells also decreased, whereas the percentage of cells showing mild and homogeneous (2 points) and strong and homogeneous (3 points) expression of ERα continued to increase. These findings indicate that feed contamination with low doses of DON increases the percentage of intestinal cells expressing ERα (2 points and 3 points) in pre-pubertal gilts.
In this analytical approach, no significant differences in the immunohistochemical expression of ERβ were noted in the cecum and the ascending colon in group C, and in the duodenal cap, the third part of the duodenum, the cecum and the descending colon in group E.
It can be assumed that the observed ERβ expression levels in the intestinal cells of control group animals (Supplementary Figure 6) are normal (physiological) and typical of pre-pubertal gilts. In proximal intestinal segments, the percentage of unstained (negative and homogeneous, 0 points) cells expressing ERβ continued to decrease on successive days of exposure. In turn, the percentage of cells where ERβ expression was strong and homogeneous (3 points) increased during the experiment. These differences in ERβ expression levels disrupt the stability of endocrine processes.
Significant differences were noted only in the duodenum (in the proximal segment) and in the descending colon (Figure 8). The presence of DON in feed increased the percentage of intestinal cells strongly expressing ERβ (3 points) from 45% in the duodenum on sampling date I to 79% in the jejunum on sampling date IV on average. More stable ERβ expression may have positive implications for endocrine processes in prepubertal gilts.
The ER expression index should be calculated for each receptor type to obtain prognostic information. The results can be used to determine the degree/direction of expression of both types of analyzed ER. The ER expression index (P-ER) was calculated in 576 samples. The mean values of P-ER reached 66 ± 10 for ERα and 26 ± 11 for ERβ in group C, and 56 ± 9 for ERα and 23 ± 7 for ERβ in group E. P-ER values were not normally distributed (Table 2).
In group C, the average value of P-ERα was determined at 66, reaching 62 and 73 in the lower and upper quartile, respectively. Based on the median, the upper and lower quartiles, the expression values were divided into four sub-groups: A – very low P-ERα (P-ERα < 62), B–low P-ERα (62 < P-ERα < 66), C– high P-ERα (66 < P-ERα < 73), and D – very high P-ERα (P-ERα > 73) (Table 2). In group C, very low (A), low (B), high (C) and very high (D) values of P-ERα were noted in 7 (29%), 4 (17%), 7 (29%) and 6 (25%) cases, respectively. The statistical analysis, conducted for the mean, median, upper and lower quartile cut-off points, revealed no significant differences.
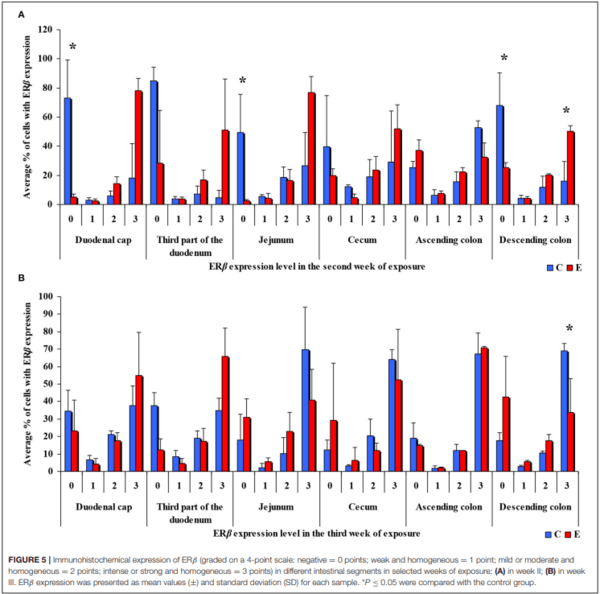
In group E, the average value of P-ERα was determined at 56, reaching 52 and 61 in the lower and upper quartile, respectively. Based on the median, the upper and lower quartiles, the expression values were divided into four sub-groups: A – very low P-ERα (P-ERα < 52), B–low P-ERα (52 ≤ P-ERα < 56), C– high P-ERα (56 < P-ERα < 61), and D – very high P-ERα (P-ERα > 61) (Table 2). In group E, very low (A), low (B), high (C) and very high (D) values of P-ERα were noted in 8 (33%), 5 (21%), 5 (21%), and 6 (25%) cases, respectively. The statistical analysis, conducted for the mean, median, upper and lower quartile cut-off points, revealed no significant differences.
In group C, the average value of P-ERβ was determined at 26, reaching 22 and 33 in the lower and upper quartile, respectively. Based on the median, the upper and lower quartiles, the expression values were divided into four sub-groups: A – very low P-ERβ (P-ERβ < 22), B–low P-ERβ (22 < P-ERβ < 26), C–high P-ERβ (26 < P-ERβ < 33), and D–very high PERβ (P-ERβ > 33) (Table 2). In group C, very low (A), low (B), high (C) and very high (D) values of P-ERβ were noted in 7 (29%), 5 (21%), 4 (17%) and 8 (33%) cases, respectively. The statistical analysis, conducted for the mean, median, upper and lower quartile cut-off points, revealed no significant differences.
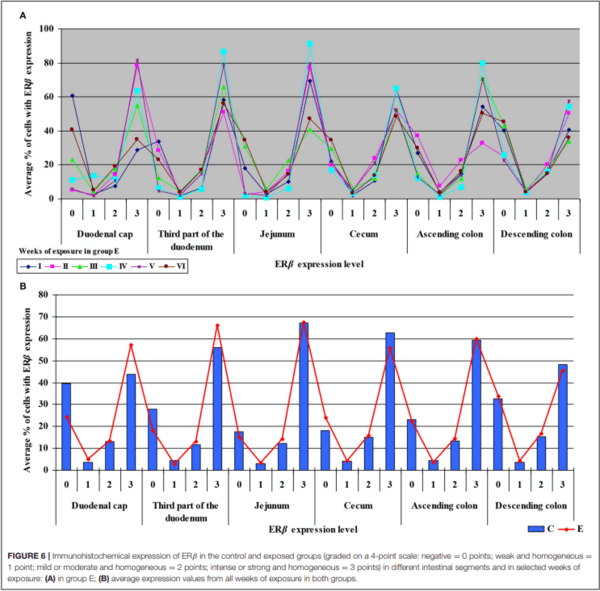
In group E, the average value of P-ERβ was determined at 23, reaching 19 and 28 in the lower and upper quartile, respectively. Based on the median, the upper and lower quartiles, the expression values were divided into four sub-groups: A – very low P-ERβ (P-ERβ < 19), B–low P-ERβ (19 < P-ERβ < 23), C–high P-ERβ (23 < P-ERβ < 28), and D – very high P-ERβ (P-ERβ > 28) (Table 2). In group E, very low (A), low (B), high (C) and very high (D) values of P- P-ERβ were noted in 7 (29%), 6 (25%), 5 (21%) and 6 (25%) cases, respectively. The statistical analysis, conducted for the mean, median, upper and lower quartile cut-off points, revealed no significant differences.
In group E, the expression of the CYP1A1 gene in the ascending colon decreased in comparison with group C, which is related to inflammation (32), except in week VI when CYP1A1 expression was significantly higher in group E. In week II, the observed difference was highly significant, and significant differences were found in weeks III, IV and V (see Figure 9A). In group E, a highly significant decrease in the expression of the GSTP1 gene was observed in weeks I to IV, and a significant decrease was noted in weeks V and VI (see Figure 9B).
In the descending colon, the expression of the CYP1A1 gene was significantly higher in group E in weeks I, IV and VI, and highly significantly higher in week V (see Figure 9C). Significant differences in the expression of the GSTP1 gene (higher values in group C) were observed only in week II. In the remaining weeks of the study, the mean values of this parameter fluctuated in both groups, but no significant differences were found (see Figure 9D).
DISCUSSION
Estrogen Receptors
There is increasing evidence to suggest that mycotoxins and their metabolites [not only mycoestrogens–(33)] are directly involved in interactions that disrupt hormonal homeostasis [including steroid hormones, as demonstrated in vitro by– (1)]. These interactions are also mediated by ER. One of the important questions that needs to be answered is whether DON, an undesirable substance of natural origin, poses potential health risks in humans (2, 24, 34) and animals (22).
TABLE 2 | ER expression at various absorption levels during the entire experiment in the analyzed segments of the gastrointestinal tract in pre-pubertal gilts.
The values of P-ERs were divided into four groups and presented for each group: very low (A), low (B), high (C) and very high (D).
Optical Density
First Analytical Approach
No differences in the expression of both ER were found in the first and last week of the experiment, which could have several explanations.
The results of this study (see Figures 3–6) can be divided into two parts, corresponding to the analyzed intestinal segments. Firstly, DON undergoes relatively rapid biotransformation (16, 35, 36) in the proximal intestinal segments (both segments of the duodenum and jejunum), regardless of the week of exposure, or a counter-response (no response) of the macroorganism to the presence of a small dose of an undesirable substance is observed (33). The absence of significant differences on the first sampling date could be attributed to a very low mycotoxin dose that is “ignored” by the body. This assumption is partially confirmed by other studies (37), and it is consistent with the Tregs theory (38) which postulates that the expression of Tregs cells is not induced when the number of infectious factors is very low. It is also possible that DON comes into short-lived contact with ER in enterocytes, or does not come into such contact at all, because the mycotoxin is too rapidly absorbed by the macroorganism. Similar conclusions were formulated in another study that investigated a different mycotoxin (23). The above could also be due to the fact that DON concentrations are initially low, and the process of tissue saturation with the mycotoxin begins only in the second week of exposure (39, 40).
Secondly, ERα expression in the descending colon in group C reached nearly 100% in week I, and it was lowest (60%) in week IV when a negative value was noted (see Supplementary Figure 2). In group E, ERα expression was mostly intense or strong and homogeneous (3 points), ranging from 80% in weeks I and VI, through 60% in week IV to 42% in week V (see Figure 4A). The above could result from a compensatory mechanism (41), where the activity of low mycotoxin doses is suppressed and initial functions are restored despite ongoing exposure. According to an in vitro study conducted by Woelflingseder et al. (42), the compensatory mechanism may interfere with the glutathione depleting effect under prolonged exposure to DON, which can potentially reduce the cytotoxic effect. On the other hand, in the present study, GSTP1 mRNA expression in both segments of the colon (see Figure 9), in particular the ascending colon (see Figure 9B), decreased in group E. The above could decrease the proliferation of epithelial cells (16), which promotes apoptosis, leading to controlled proliferation (43).
Therefore, the observed changes could be attributed to compensation as well as chemical stress resulting from exposure to DON (20).
Second Analytical Approach
This analytical approach confirms the observations made by Demaegdt et al. (1) that DON is a partial agonist of ERα. In this study, the immunohistochemical expression of ERα was mild or moderate and homogeneous (2 points) and intense or strong and homogeneous (3 points). The differences in the percentage of ERα-expressing cells were considerable (10% to 15% on average), but not statistically significant, and they were noted mainly in weeks III, IV and V (see Figure 7) in the duodenal cap, the third part of the duodenum, the jejunum and the ascending colon. These observations could be attributed to prolonged exposure to DON which disrupts physiological processes (8) and cell signaling and, consequently, leads to the inhibition of protein and nucleic acid synthesis, and disruption of steroidogenesis caused by cytochrome P450 (2). Steroidogenesis processes occur in tissues where steroidogenic enzymes are expressed, including the gastrointestinal tract (44). The disrupting effects of DON are limited to local tissues (16), as confirmed by the decrease in CYP1A1 mRNA expression in the ascending colon (see Figure 9A), but not in the descending colon in group E.
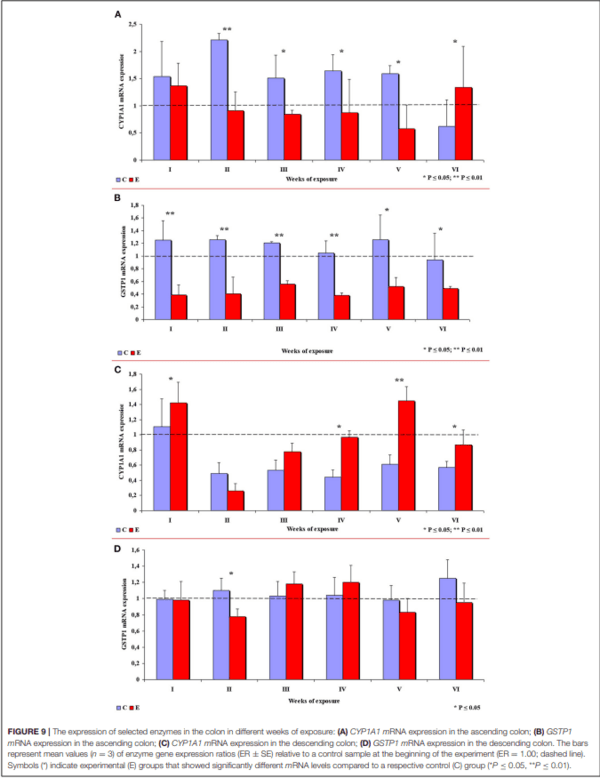
In the second analytical approach, the immunohistochemical expression of ERβ in group C was mainly negative (0 points) and intense or strong and homogeneous (3 points) (see Supplementary Figure 6). Negative expression was noted mainly in weeks II and I. Intense or strong and homogeneous expression (3 points) was observed mainly in weeks IV, V and VI in the duodenal cap, the third part of the duodenum, the jejunum and the descending colon. Considerable changes were noted in group E (see Figure 8): significant differences were found only in the duodenal cap and the ascending colon where ERβ expression was evaluated as intense or strong and homogeneous (3 points); a general decrease in expression processes was also noted in this group. In the highest percentage of ERβ-expressing cells, ERβ expression was also intense or strong and homogeneous (3 points) (see Figure 8B). An analysis of the results noted on each sampling date revealed that DON was accumulated until week V, particularly in the ascending colon. This specific situation observed at the end of the experiment may be attributed to the transactivation of ERβ (45), which could be due to intensified apoptosis (46) and proliferation in intestinal tissues as a result of Ying/Yang interactions (47). These findings could also be explained by the absence of competition (absence of ERα) in selected tissues [such as the colonic mucosa–(48)] whose functions are not affected by ERα. The colon is one of the key organs targeted by DON, which is why the observations made in this region are most pronounced. These processes are accompanied by histological changes which have been described by Pinton and Oswald (49) and Przybylska-Gornowicz et al. (50), and which take place mainly in the mucosa. Non-genomic factors that modulate regulatory membrane proteins could also play a role (47, 51).
In the second analytical approach, enhanced expression of ERβ under exposure to a low DON dose was a biological effect that inhibited ERα-related functions (52). A comparison of both analytical approaches with previous findings (9) indicates that the immunohistochemical expression of ER proceeds differently, which could be attributed to the fact that the cited study examined the effects of mixed mycotoxicosis (DON/ZEN). The exposure to both DON and ZEN increased ERα expression in the jejunum and the ascending colon, but expression levels in these intestinal segments continued to decrease during exposure. The expression of ERß decreased steadily during exposure, in particular in the cecum and the descending colon. The expression of ERß continued to increase in the remaining intestinal segments. These observations suggest that during mixed (DON/ZEN) mycotoxicosis, ERß plays a dominant role in the intestinal mucosa of pre-pubertal gilts. A comparison of previous findings (9) with the results of the present study indicates that at low concentration, DON acts as a partial agonist that only partially activates the receptors.
ER Expression Index
The ER index was calculated by comparing P-ERα/P-ERβ because both ER occur in various proportions. An increase in the ER index points to enhanced proliferative processes resulting from higher expression of ERα. A decrease in the ER index suggests that steroidogenesis processes were inhibited and that ERβ expression was enhanced. The ER expression index (PERα/P-ERβ) was calculated to determine whether DON is a potential disruptor of hormonal homeostasis at the level of nuclear receptor signaling or, in other words, whether DON can act as an exogenous ligand for both ERα and ERβ. The values of the ER expression index were higher in group E between the cecum and the descending colon. However, the graphic presentation of ER expression (see Figure 4B) revealed that ERα expression decreased whereas ERβ expression remained at a similar level in both groups (see Figure 6B). The slight differences between groups indicate that DON is a relatively weak agonist in vivo, but it is not a weak antagonist of ER as suggested by Demaegdt et al. (1). This minor difference in activity could lead to hormonal activation resulting from the fact that plant materials generally contain much higher levels of DON than other Fusarium mycotoxins (6), which suggests that the bioavailability of DON increases during exposure (16).
The fact that DON is capable of inducing the expression of ERβ at the same level as in group C (see Figure 6B) which is more abundant in intestinal walls (47), indicates that this mycotoxin can participate in steroidogenesis (2) and enhances antioxidant activity. The latter observation is justified by the fact that both ER mediate estrogen activity. An important question that should be answered is whether DON can be regarded as an antioxidant solely because it induces the expression of ER. At the same time, DON inhibits antioxidant enzymes, thus increasing the production of ROS (45), and decreasing intracellular GSH levels (42). In the present study, GSTP1 mRNA expression decreased in the colon (see Supplementary Figures 6B,D) (excluding in the descending colon in weeks III and IV–see Supplementary Figure 6D), the immunohistochemical expression of ERα decreased and ERβ expression remained unchanged.
The maintenance of ERβ expression (see Figure 6B) and supression of ERα (see Figure 4B) may lead to the stimulation of cell proliferation and promotion of apoptosis (47). The above suggests that the presence of DON in feed can offer protection against selected hormone-sensitive tumors and neoplastic changes in the large intestine in mammals.
mRNA Expression of Genes Encoding Selected Enzymes in the Colon
Unlike some other mycotoxins, DON is not biologically transformed into more toxic compounds and is not oxidized to less toxic compounds during phase I biotransformation (16).
Various DON inactivation mechanisms have been identified in phase II biotransformation. One of these mechanisms involves the induction of GSTπ subfamilies, which suggests that DON can act as a substrate for GST during phase II biotransformation (53). A different hypothesis postulates that GST activity increases due to the oxidative stress induced by DON (53).
The absence of definitive answers to the above questions was the key motivation for investigating the effect of DON on the activity of two enzymes, CYP1A1 and GSTP1, which play important roles in the biotransformation of Fusarium mycotoxins.
CYP1A1 Metabolic Enzyme
A review of the literature indicates that DON disrupts cell signaling in mammals (34) and exerts multidirectional effects by distorting gastrointestinal homeostasis and steroidogenesis with the involvement of cytochrome P450 (CYP) (2, 44). Some of CYP enzymes, e.g., CYP1A1, are involved in the conversion of procarcinogens to carcinogens (16, 17). Transcriptional activation of the CYP1A1 gene can take place under exposure to undesirable substances such as DON (16, 34, 44) or phytoestrogens (18). Detoxifying effects can be achieved by suppressing the activity of the CYP1A1 enzyme (22). This type of activity was detected in the ascending colon on nearly all sampling dates (see Figure 9A), excluding week VI. The results noted on the last sampling date are difficult to interpret because expression levels were higher in group E. It has been hypothesized that CYP1A1 alleles may increase the risk of cancer because they encode enzymes with higher metabolic activity (54). However, it should be noted that the accumulation of DON in tissues (22) increased in successive weeks of exposure. The above suggests that biotransformation processes do not evolve continuously during exposure, and the carryover factor increased from 0.012– 0.022 in weeks I to IV to 0.032–0.049 in the last week of the experiment. The above interpretation does not apply to the descending colon (see Figure 9C), where the carry-over of DON was detected only in week IV. These results can be extrapolated to suggest that the “transient dysbiosis” of the gut microbiota plays a key role in the biotransformation of DON, in particular in the large intestine of pre-pubertal gilts (40–56). However, further research is needed to validate this assumption in all mammals.
Considerable differences in CYP1A1 mRNA expression were found between the ascending colon and the descending colon, which complicates the interpretation of the results. The presence of DON in ingested feed inhibited gene expression in the ascending colon, which suggests that this mycotoxin can contribute to preventing steroid-dependent carcinogenesis. In the descending colon, DON increased gene expression, which, together with the presence of endogenous factors in the intestinal lumen, points to an increase in the enzyme’s detoxifying activity.
GSTP1 Gene Encoding Phase II Metabolic Enzymes
In the current study, an attempt was also made to determine whether prolonged exposure to a low dose of DON modifies GSTP1 mRNA expression and the associated processes in prepubertal gilts. According to Woelflingseder et al. (42), the in vitro modulatory effects of GSH on DON toxicity led to only a minor suppression of proliferative processes and cytotoxic effects. In the present study, GSTP1 mRNA expression decreased steadily in the ascending colon during the experiment (see Figure 9B). However, in the descending colon, GSTP1 mRNA expression was higher in group E in weeks III and IV (see Figure 9D). These observations could be attributed to: (i) balanced demand and supply (homeostasis) of GSTP1 mRNA which is required for the maintenance of cytoprotective and detoxifying functions during phase II biotransformation (20); (ii) excessive saturation with intracellular GSH in the ascending colon and the descending colon in response to the overexpression of GSTP1 mRNA (19); (iii) intensified transactivation of ERβ (45); or (iv) intensified apoptosis (34, 46) and/or controlled proliferation in intestinal tissues (34, 47). These observations are consistent with the results reported in vitro (42).
The exposure to a low mycotoxin dose led to a controlled decrease in GSTP1 mRNA expression, and a consensus was maintained between intestinal cells, the degree of exposure to an undesirable substance and the detoxifying effect.
Summary
The aim of this study was to determine in vivo the effect of DON, administered per os at 12 µg/kg BW to pre-pubertal gilts for 42 days, on the immunohistochemical expression of intestinal ERs and the expression of genes encoding CYP1A1 mRNA and GSTP1 mRNA in the colon on different sampling dates. The research findings indicate that exposure to the analyzed DON dose led to (i) a decrease in ERα expression by ∼15% between the jejunum and the descending colon; (ii) an increase in ERβ expression in the duodenum, and the maintenance of its expression at the same level as in group C in the remaining intestinal segments; (iii) a significant decrease in CYP1A1 mRNA expression in the ascending colon, and a significant increase in its expression in the descending colon; and (iv) a decrease in GSTP1 mRNA expression in the colon on most sampling dates.
To the best of the authors’ knowledge, the is the first study to indirectly demonstrate that the presence of the analyzed DON dose in feed (12 µg DON/kg BW per os for a period 42 days) probably offers protection against uncontrolled proliferation in the large intestine of pre-pubertal gilts, and contributes to maintaining a stable consensus between the degree of exposure to DON and the detoxifying effect in mammals.
This article was originally published in Frontiers in Veterinary Science. 8:644549. doi: 10.3389/fvets.2021.644549. This is an Open Access article distributed under the terms of the Creative Commons Attribution License (CC BY).