1. Introduction
Phytase is the enzyme that hydrolyzes phytic acid and its salt phytate (myo-inositol hexakisphosphate, IP6), resulting in the formation of inositol pentakis-, tetrakis-, tris-, bis- and monophosphate (IP5-IP1) in a stepwise manner and five inorganic phosphates (Pi) (Skoglund et al., 1998; Greiner et al., 2000). Inclusion of phytase in animal feed not only makes the phytate-bound phosphorus (0.2 to 1.0 % in feed stuff) available to the animal, but also inactivates phytate’s anti- nutritional effect, thus increasing the digestibility of protein and minerals (Reddy 2002; Cowieson et al., 2008). Phytase of microbial origin is one of the most important enzymes used in treatment of animal feed, accounting for some 50% of the feed enzyme market. Commercial phytases are mainly derived from E. coli and fungi (Weaver and Kannan, 2002; Selle and Ravidran, 2007). These histidine acid phytases active in the acidic pH range include bacterial (EC 3.1.3.26) and fungal (EC 3.1.3.8) phytases (Wyss et al., 1999; Mulaney and Ullah 2007; Tran et al., 2011). Additional desirable properties of feed phytases are its stability to pepsin digestion andin-feed thermostability during feed processing and storage.
Phytase from the bacterium Buttiauxella sp. GC21 has been reported from grass carp intestine (Shi et al., 2008). Enzymatically it shows similar properties such as optimum pH and thermo-instability to the industrially widely used E. coli phytase (Shi et al., 2008). The phytase cloned from Buttiauxella soil isolate P1-29 from Kantvik (Finland) (wtPhytase Butt) reported here is a 413 amino acid long peptide with a molecular mass of 45.4 kDa, the same length as the one isolated from Buttiauxella sp. GC21 (GenBank no. ABX80238.1) (Shi et al., 2008). wtPhytase Butt shows 48 and 88 % identity at protein sequence level, respectively, to E. coli and Buttiauxella sp. GC21 phytases (Shi et al., 2008). In the current study we report the characterization of a mutant Buttiauxella sp. phytase (mPhytase Butt) mutated from wtPhytase by protein engineering. We will report the mutation, cloning, and expression of this Buttiauxella sp. phytase elsewhere. In comparison to wtPhytase Butt and also the Buttiauxella phytase reported before (Shi et al., 2008), the current study shows that mPhytase Butt has not only improved thermostability but also exhibits high activity toward substrates of both phytic acid and phyticacid-protein complex, and improved pepsin stability.
2. Methods
2.1 Chemicals, enzymes and material.
Porcine pepsin (P7000, 250U/mg, P7012, 2190 U/mg), sodium phytate from rice (P3168, lot123k1413, purity 95 % (w/w)), and bovine serum albumin (BSA, A7906) were all from Sigma- Aldrich (St Louis, MO). Escherichia coli phytase var. 1, Peniophora lycii phytase (EC 3.1.3.26), Aspergillus niger phytase (EC 3.1.3.8) were obtained as described earlier (Tran et al., 2011).
2.2 Purification of wtPhytase Butt and mPhytase Butt
Purification of mPhytase Butt and wtPhytase Butt expressed in Trichoderma reesei was achieved using Butyl HIC column and cation exchange chromatography on Source S15 (GE Healthcare, Uppsala, Sweden). The concentrated and ultrafiltered broth of T. reesei samples (4 ml) were mixed with 2 ml of deionized water and 2 ml of 4 M ammonium sulfate. The prepared samples (8 ml) were applied onto a 5 mlButyl-HiTrap FF column mounted on Äkta Purifier (GE Healthcare) at a flow rate of 2 ml/min. The columns were pre-equilibratedin 20 mM sodium phosphate (pH 6.0) containing 1 M ammonium sulfate. The run through fraction from Butyl HIC was desalted on a PD10 column pre-equilibratedwith sodium acetate (20 mM, pH 4.5) (Buffer A). The desalted sample (3.5 ml) was applied to a 10 ml Source S15 column pre-equilibratedin Buffer A at 3.5 ml/min. The column was washed with Buffer A and bound proteins were eluted with a linear gradient of 0-0.4M NaCl in Buffer A. Active fractions having phytase activity were pooled, concentrated, analyzed on SDS-PAGE(Figure 1), and used for all experiments described in this study. The protein concentration of the active fractions was determined using Bio-Radprotein assay with bovine serum albumin (BSA) as standard according to the manufacturer’s instructions (BioRad, Hercules, CA).
Fig. 1.SDS-PAGEanalysis of mPhytase Butt on NuPAGE with a gel gradient of4-12%(Invitrogen, Naerum, Denmark). Lane 1, 3, 7 are molecular markers in kDa, Lane 2, filtered fermentation broth, Lane4-6were purified fractions of mPhytase Butt used in the current substrate characterization. Arrows indicate the mPhytase Butt protein confirmed by amino acid sequencing and having a molecular mass of 45 kDa in monomeric form.
Analysis of phytate and myo-inositol phosphate isomers by HPIC was performed as described earlier (Skoglund et al., 1998; Yu et al., 2012). Inorganic phosphate (Pi) released from phytic acid by the action of phytases at pH 2.5 and 5.5 was analyzed by traditional phosphate colorimetry using Konelab from Thermo Fisher Scientific (Slangerup, Denmark) (Tran et al., 2011). International standard phytase unit FTU is adopted, which is the release of 1 µmole Pi per min at 37oC, pH5.5 and 5 mM of sodium phytate (Engelen et al., 2011).
2.3 Assay of phytase activity
Standard phytase activity assay was performed as described earlier (Engelen et al., 2011). The reaction mixture contained in acetate buffer (0.25 M, pH5.5) 1 ml phytase sample (containing 0.03 FTU/mL) and 2 ml sodium phytate (7.5 mM), CaCl2 (1 mM), and Tween 20 (0.01%, w/v). The reaction was carried out at 37.0°C for 60 min and was stopped by the addition of 2 ml vanadate- molybdate reagent. After centrifugation at 3500g for 10 min, the absorbance of the supernatant was measured at 415nm. Preferred reading at 415nm is 0.4 -1.6. Phosphate released was quantified by using KH2PO4 as standard. When phytic acid at final concentration of 75 µM was used, released Pi was measured at 600nm using the Malachite green reagent (PiBlue™ Phosphate Assay Kit, BioAssay Systems, Hayward, CA) (Fisher and Higgins, 1994). Km and Vmax were determined at pH 4.5 and 40oC in 0.1 M acetate and a reaction time of 2 min. The sodium phytate concentration used was 0.05 to 2.00mM. Released phosphate was measured by the Malachite green assay.
2.4 Preparation of sodium phytate hydrolyzates
The reaction mixture contained sodium phytate (10.53 mM) and mPhytase Butt (0.2 FTU/ml) in glycine-HCl(0.25 M, pH2.5). The reaction was carried out at 37oC and samples of 0.5 ml were taken at 0, 10, 30, 60, 90 and 120 min and heated in a water bath for 5 min to inactivate the phytase. The hydrolyzate was analyzed forIP2-IP6 distribution by HPIC (Skoglund et al., 1998), pepsin inhibition and soy protein aggregation (Yu et al., 2012)
2.5 Assay for pepsin inhibition by phytic acid hydrolyzates
For the assay of pepsin inhibition, the reaction mixture contained 1 Megazyme AZCL-caseintablet (Megazyme Intl Ireland), 81 µl sodium phytate hydrolyzates prepared from IP6 (10.53 mM) obtained with a hydrolysis time of 0-120min, pepsin (Sigma P7012, 0.5 mg or 1095 U) and glycine- HCl (0.25 M, pH 2.5) to a final volume of 2.0 ml. For control, IP6 hydrolyzate was replaced with the glycine-HClbuffer. The reaction was performed at 40oC for 15 min and was stopped by adding 10 ml of 2% (w/v) trisodium phosphate. Absorbance was measured at 590nm as an indication of pepsin activity. Each data point is the average of 3 measurements.
2.6 Assay for protein aggregation by phytic acid hydrolyzate
The reaction system contained 1.2 µl IP6 or IP6 hydrolyzate from 84 µM of phytate, and 0.1 ml soy protein in glycine-HCl(0.25 M, pH 2.5) added to a half bottom area microplate. Absorbance at 600nm was measured at 30oC as an indication of turbidity after mixing to determine protein aggregation by phytic acid hydrolyzate. Each data point is the average of 4 measurements.
3. Results and discussion
3.1 Thermostability of mPhytase Butt
Phytase as a feed additive enzyme requires certain storage stability from the time it is produced to when it is mixed with feed and premix ingredients. High thermostability is also required because two thirds of animal feed produced today is pelleted at high temperature (75-95°C) for various reasons, including reducing microbial loads and inactivating certain digestive enzyme inhibitors (Amerah et al., 2011). Thus development of mutant phytases often has thermostability improvement as the major goal. Figure 2a shows that mPhytase retained 90% activity at 60°C for 60 min while wtPhytase Butt and E. coli phytase var. 1 had around 5% residual activity. The thermo-instability of wtPhytase Butt from Buttiauxella sp. is well in line with that of wtPhytase Butt from Buttiauxella sp. GC21 which is also thermo-instable, losing about 30% activity at 60°C in 10 min (Shi et al., 2008). Additional studies show that mPhytase Butt is stable up to 67oC when pre- incubated at various temperatures for 10 min followed by activity assay at 37°C and pH 5.5.
Fig. 2a. Thermostability of mPhytase Butt, wtPhytase Butt and the E. coli phytase. The phytases were pre-incubatedat 60oC in 0.1 M acetate buffer at pH 4.5 for 0 to 60 min. Samples were taken at various time points and measured for residual phytase activity. The reaction mixture contained 40 µl phytase in 0.1 M acetate buffer at pH 4.5 and sodium phytate at a final concentration of 75 µM in a final volume of 80 µl. The reaction was carried out at 60oC for 10min. Each data point is the average of 3 measurements. Symbols: ♦, mPhytase Butt; ?, wtPhytase Butt; ¦, the E. coli phytase.
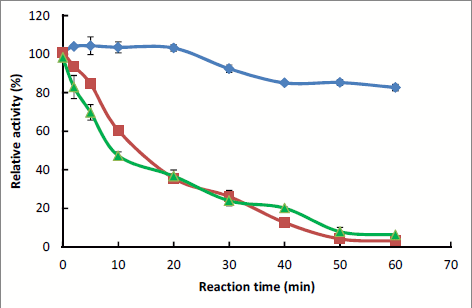
Fig 2b. The temperature optimum of mPhytase in comparison with wtPhytase Butt and E. coli phytase. The reaction mixture contained 0.1M acetate buffer (pH4.5) and sodium phytate 75 µM in a final volume of 80 µl. The reaction was carried out at various temperatures for 10min. The activity at 30oC was regarded as 100%. Each data point is the average of 3 measurements. Symbols: ?, mPhytase Butt; ?wtPhytase Butt; ¦, the E. coli phytase.
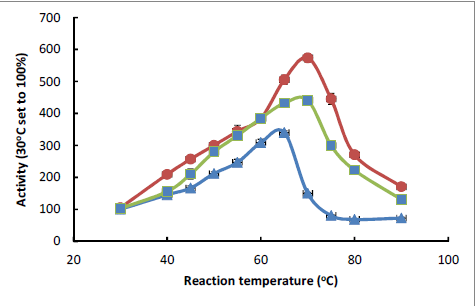
Figure 2b shows the temperature optimum of mPhytase Butt, wtPhytase Butt and the E. coli phytase. When assayed at pH4.5 and a phytic acid concentration of 75 µM mPhytase Butt showed an optimum temperature of 70°C, 5°C higher than wtPhytase Butt and 2°C higher than the E. coli phytase. It is also interesting to note that mPhytase Butt shows higher catalytic activity than the E. coli phytase and much higher activity than wtPhytase Butt at temperatures higher than 30°C (Figure 2b). For example, at the physiological relevant temperature of 37-40°C for poultry and pigs, mPhytase Butt showed about 60% and 50% higher activity than wtPhytase Butt and the E. coli phytase, respectively.
3.2 Pepsin stability of mPhytase Butt
In addition to thermostability, another preferred requirement for a feed phytase is its resistance to pepsin at physiologically low pH. Figure 3 shows that wtPhytase Butt completely lost its activity after 30 min incubation in the presence of porcine pepsin (0.25 mg/ml or 62.5 U/ml) at pH 2.0 and 23°C. In contrast, mPhytase Butt was stable for at least 120 min under these conditions. It is known in the literature that the fungal phytases from Aspergillus and Peniophora have limited pepsin stability (Greiner and Farouk, 2007).
Fig. 3. Stability of mPhytase Butt and wtPhytase Butt to porcine pepsin at pH 2.0. The incubation mixture for pepsin stability contained in 10 ml glycine-HCl(1 M, pH 2.0), porcine pepsin 2.5 mg (or 625 U), BSA 30 mg, and 5 FTU of mPhytase Butt or wtPhytase Butt. The mixture was incubated at room temperature (23 °C) and at intervals of 0, 30, 60, and 120 min 0.1 ml samples were taken and analyzed for residual phytase activity using the standard phytase assay method by measuring the release of phosphate with the exception that pH was 5.25 instead of 5.50. Each data point is the average of 2 measurements and the standard deviation is smaller than the symbols. Symbols: ?, mPhytase Butt, ?, wtPhytase Butt.
3.3 pH optimum of mPhytase Butt
The pH optimum of mPhytase Butt was around pH 4.0 (Figure 4a and 4b), which is a 0.5-1.0pH unit shift to the acidic region compared to the pH optimum of the E. coli phytase (Greiner et al., 1993; Wyss et al., 1999) and wtPhytase Butt from Buttiauxella sp. GC21 (Shi et al., 2008). It can be seen that if the activity at pH 5.5 and 5 mM of phytic acid (under which international phytase activity unit FTU is defined) is regarded as 100%, mPhytase Butt shows a 1.5 to 2.5 fold higher activity than the E. coli phytase in the pH range of pH 3 to 5 (Figure 4a). When a lower substrate concentration (75 µM phytic acid) was used, mPhytase Butt showed a 2.5-4 fold higher activity than the E. coli phytase in pH 3 to 5 (Figure 4b). The higher activity of mPhytase Butt at low pH compared to the E. coli and fungal phytases is further demonstrated using High Pressure Ion Chromatography (HPIC) by following the IP6 hydrolysis at pH 2.5 (see Figure 6 below).
Fig. 4a. The pH optimum of mPhytase Butt and E. coli phytase with sodium phytate (5 mM) as substrate. Phytase activity was assayed in glycine-HCl(pH2.0-3.0),acetate buffer (pH3.5- 5.5), Mes-NaOH(pH6) with a reaction time of 60 min. Final buffer concentration was 0.2 M, final sodium phytate concentration was 5 mM. Activity at pH 5.5 was regarded as 100%. Each data point is the average of 3 measurements. Symbols: ♦, mPhytase Butt; ¦, the E. coli phytase.
Fig 4b.The pH optimum of mPhytase Butt and E. coli phytase with sodium phytate (75 µM) as substrate. Phytase activity was assayed under conditions described in Figure 4a except that a final sodium phytate concentration of 75 µM and final buffer concentration of 0.1 M with a reaction time of 10 min were used. Each data point is the average of 3 measurements. Symbols: ♦, mPhytase Butt; ¦, the E. coli phytase.
3.4 pH optimum of mPhytase Butt using phyticacid-proteincomplex as substrate
Phytic acid may be present in complex with proteins, for example at low pH in the upper digestive tract of the animal or in plant seeds in the form of globoids (Bohn et al., 2007). The formation of phytic acid-proteincomplex is mainly due to the charge interaction between negatively charged phytic acid and positively charged proteins (Reddy et al., 1989; Kies et al., 2006; Yu et al., 2012, 19- 22). Figure 5 shows that mPhytase Butt had high activity between pH 2.7 and 4.9 when phytic acid- lysozyme complex was used as the model substrate. This study indicates that mPhytase Butt is not only active on phytic acid but also on phytic acid-proteincomplex. In Figure 5 lysozyme was used as a model protein as it can form complex with phytic acid at a wider pH range due to its high pI value (Tran et al., 2011; Yu et al., 2012). The results shown in Figure 5 are further confirmed in Table 1, which shows that mPhytase Butt had a much higher activity toward both phytic acid and phytic acid-soy protein complex at pH 3.0 than the E. coli phytase and A. niger phytase, the two industrial standard phytases being used in feed applications since the last two decades.
Fig. 5.Activity of mPhytase Butt as a function of pH using phytic acid-lysozyme complex as substrate. The reaction mixture consisted of 45 µl buffer, 3 µl lysozyme (41.4 mg/ml), 1.5 µl sodium phytate (10 mM). After shaking at 800 rpm in an Eppendorf shaker for 1 min, 1.5 µl mPhytase Butt was added and the reading at 600nm was followed every 1 min with shaking at 30oC. The buffers used were 50 mM glycine-HCl (2.7-3.1) and 50 mM acetate (3.9-5.4). Phytase activity is expressed as turbidity decrease (mOD/min) at 30oC as a function of pH. Each data point is the average of 4 measurements.
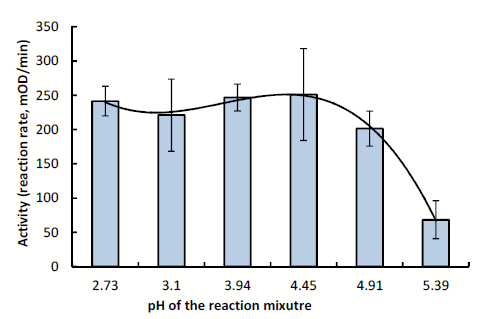
Table 1Activity of representative microbial phytases on phytic acid and phyticacid-soy protein complex as substrates at pH 3.0. Phytase activity was measured by the Malachite green assay with a final phytic acid concentration of 75 µM with and without the addition of soy protein (5 mg/mL). Total reaction volume was 80 µl in 0.1 M glycine buffer (pH 3.0) with a reaction time of 10 min. The data are expressed in average of 3 measurements plus standard deviation. Activity of mPhytase Butt with phytic acid as substrate is set as 100%.
3.5 The Km and Kcat of mPhytase Butt
The Km and Vmax for mPhytase were determined to be 0.16 mM and 447 µmole Pi released/mg protein/min, respectively, when assayed in 0.1 M acetate buffer at pH 4.5 and 40oC with a reaction time of 2 min. The calculated turnover number Kcat and the catalytic efficacy Km/Kcat were 339 [s-1] and 2.1 [106 s-1M-1], respectively. The Km and Vmax values determined for the E. coli phytase under this condition were 0.30 mM and 509 µmole Pi released/mg protein/min. The calculated Kcat and Km/Kcat for the E. coli phytase were 389 [s-1] and 1.3 [106 s-1M-1], respectively. These data indicate that two bacterial phytases showed quite similar turnover numbers and due to the lower Km of mPhytase Butt it had 60% higher catalytic efficacy. The lower Km of mPhytase Butt than the E. coli phytase may explain its higher catalytic efficacy at lower substrate concentrations (Figure 4a vs. 4b).
3.6 Hydrolysis of IP6 and IP5 by mPhytase Butt
Phytase catalyzed reaction is quite complicated because the substrate composition (IP2-IP6)and concentration change with the reaction progress as does the product composition and concentration (Pi and IP2-IP5),making HPIC an ideal method to follow the reaction as it can detect the levels of IP2-IP6in a single run (Yu et al., 2012). From Figure 6a, it is shown that the hydrolysis rate of IP6 at pH 2.5 by mPhytase Butt was much higher than the E. coli and A. niger phytase on equal activity unit (FTU), for instance, at 10 min reaction 60% IP6 had been hydrolyzed by mPhytase Butt while for the E. coli and A. niger phytases, the number was 20%. Furthermore it can be seen that Peniophora lycii phytase virtually had little or no activity at this pH (Figure 6a). Figure 6b also shows the faster turnover of IP5 by mPhytase Butt compared to the E. coli phytase and the accumulation of IP5 by A. niger phytase. mPhytase Butt also had fast turnover of IP4-IP2 than the E. coli and A. niger phytase (figure not shown). These observations are in agreement with the results of total Pi release from IP6 with time by the 4 phytases (Figure 6c), for example, mPhytase Butt released about 65 % more Pi than the E. coli and A. niger phytase at pH 2.5 at a reaction time of 100 min. The ability of mPhytase Butt to hydrolyze phytic acid was studied at pH 2.5 because the pH of the first part of the digestive tract can be as low as pH 2.5 and also A. niger phytase is known to have high activity at pH 2.5 (Wyss et al., 1999).
Fig. 6.A comparison of microbial phytases for their hydrolysis of phytate (IP6) and IP5 as well as the release of phosphate at pH 2.5. The reaction mixture contained sodium phytate (2.63 mM) and mPhytase Butt (0.05 FTU/ml reaction mixture) in glycine-HCl (0.25 M, pH2.5). The reaction temperature was 37°C and at certain time intervals 0.5 ml samples of reaction mixture were taken and mixed with 0.2 ml of HCl (1 M) to stop the reaction, filtered and used for HPIC analysis of IP6 (Fig 6a) and IP5 (Fig 6b), and the release of phosphate (Fig 6c). Each data point is the average of two HPIC runs for Fig 6a and 6b and two measurements for Fig 6c. Symbols: ?, mPhytase Butt; ¦, E. coli phytase; ? A. niger phytase; ?, P. lycii phytase.
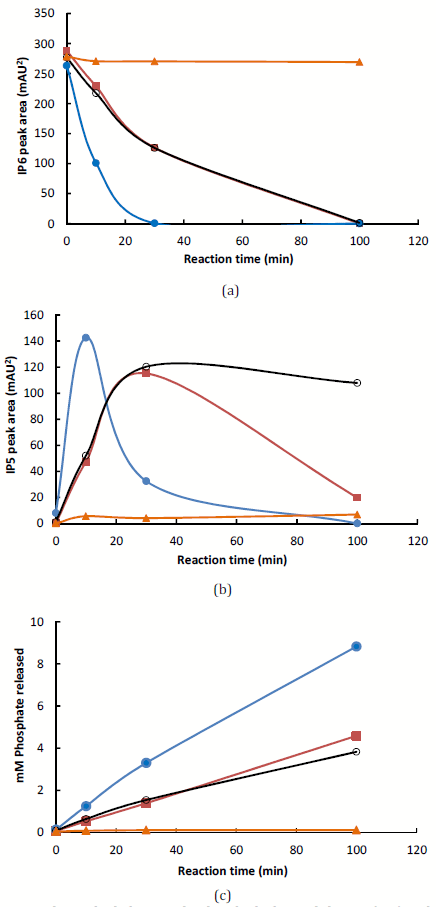
Animal feeding trials have confirmed that one of the main functional sites in pigs for supplemental phytase is the stomach (Jongbloed et al., 1992; Yi and Kornegay, 1996). It has been assumed that the initial hydrolysis of IP6 and IP5 is essential in the stomach since IP1-4,unlike IP6 and IP5, has negligible interactions with proteins (Yu et al., 2012), and may also be broken down later in the digestive tract by intestinal alkaline phosphatases (Yi and Kornegay, 1996). When the 4 microbial phytases in Figure 6 were examined for IP6 hydrolysis by HPIC dosed on equal FTU the degradation of IP6 and total phosphate released were basically identical for all the 4 phytases as it should (figure not shown).
3.7 The hydrolysis products of mPhytase Butt
Current feed phytases including phytases from E. coli and A. niger all belong to the histidine acid phytase family as their final products from 1 mole phytate are 5 mole Pi and 1 mole myo-inositol 2- phosphate (Yu et al., 2012). It would be of interest to know if mPhytase Butt shares the same reaction mechanism as phytases from E. coli and A. niger. 31P-NMR was used to follow mPhytase Butt catalyzed phytic acid hydrolysis. The final products of mPhytase Butt action on phytic acid were found to be Pi and myo-inositol monophosphate (IP1) (Figure 7). It can be seen from Figure 7 that 4 peaks characteristic of phytic acid at time 0 min all disappeared after 21 h incubation at 37°C while new peaks representing its degradation products including Pi, appeared already at an incubation time of 15 min and increased with time. The assumed IP1 peak was visible at 90 min at near 1.2 ppm as indicated by the arrow (Figure 7) (Kemme et al., 1999). These observations were also seen using phyticacid-lysozyme complex as substrate (data not shown).
Fig. 7.Phytate hydrolysis by mPhytase Butt monitored by 31P-NMR. The assay mixture contained mPhytase Butt (0.07 FTU), sodium phytate (20 mg) in 1.0 ml of glycine-HCl (D2O) (1 M, pH 3.2). The reaction temperature was 37oC. 31P-NMR spectrum was recorded at different time intervals indicated. The position of IP1 at 1.2 ppm was shown by arrows.
Further confirmation of the final products Pi and myo-inositol monophosphate was achieved by HPIC and assay for phosphate released from known molar phytic acid. HPIC analysis also showed that the initial position of hydrolysis of phytic acid was primarily position 6 on the myo-inositol ring with InsP(1,2,3,4,5) as the product. This thus establishes mPhytase Butt as a member of the 6- phytase family as E. coli phytase and different from A. niger phytase, which is a known3-phytase with initial hydrolysis at position 3 (figure not shown).
3.8 mPhytase Butt relieves inhibition of IP5 and IP6 on pepsin catalyzed protein hydrolysis
Figure 8 shows that when assayed at pH 2.5, pepsin had low activity in the presence of physiological relevant concentrations of phytic acid (0.426 mM or 0.28 % (w/v)) at reaction time zero, corresponding 70-80%inhibition compared to control. With the IP6 hydrolysis progressed, the hydrolyzate of mPhytase Butt became less and less inhibitory to pepsin and at a reaction time of 60 min when all IP6 was hydrolyzed to IP5 and IP4, only 30% of the pepsin activity was inhibited. In contrast, pepsin activity remained low (75% inhibited comparing to control) in the presence of IP6 hydrolyzate prepared by A. niger phytase even at a IP6 hydrolysis time of 120 min as there were still 24% IP6 unhydrolyzed (figure not shown). The capability of the hydrolyzates prepared by the two phytases to aggregate soy protein decreased with the increase in reaction time and IP6 hydrolysis (Figure 8). It has been proposed that lowered pepsin activity is due to phytic acid induced protein aggregation which decreased its accessibility to pepsin (Yu et al., 2012).
Fig. 8.Pepsin activity and soy protein aggregation as a function of phytate hydrolysis by mPhytase Butt. Sodium phytate (10.53 mM) was hydrolyzed by mPhytase Butt at pH 2.5 and 37oC for 2 h. Samples were taken at 0, 10, 30, 60, 90 and 120 min, heated at 100oC to stop the reaction and diluted for assaying pepsin inhibition and soy protein aggregation at pH 2.5 detailed in the Methods section. Symbols: ?, IP6 peak area;♦ , IP5 peak area; ?, pepsin activity; Δ, soy protein turbidity.
4. Conclusions
The current study shows that mPhytase Butt, a mutant derived from the wild type phytase isolated from the soil-bornbacterium Buttiauxella sp. (wtPhytase Butt), has improved thermostability. More interestingly mPhytase Butt has much higher activity at 37-40oC and pH 3 to 5 compared to the E. coli phytase. It also has improved pepsin tolerance, higher activity toward both phytic acid and phyticacid-protein complex at low pH compared to the other microbial phytases examined. The high activity of mPhytase Butt at low pH could be advantageous in animal feed for efficiently releasing phosphate from phytate, thus inactivating itsanti-nutritional effect. The overall improved performance of mPhytase Butt vs. wtPhytase Butt needs to be further understood at molecular and structural levels.
Acknowledgements
Thanks are given to Lotte Bisgaard, Trine Christensen, and many other colleagues for their technical assistance. The authors are grateful to Dr. Roopa Ghirnikar for her reading of the work.
References
Amerah AM, Gilbert C, Simmins PH, Ravindran V: Influence of feed processing on the efficacy of exogenous enzymes in broiler diets. World Poult Sci J, 2011, 67:29-46.http://dx.doi.org/10.1017/S0043933911000031
Bohn L, Josefsen L, Meyer AS, Rasmussen SK: Quantitative analysis of phytate globoids isolated from wheat bran and characterization of their sequential dephosphorylation by wheat phytase. J Agric Food Chem 2007,55:7547–7552 http://dx.doi.org/10.1021/jf071191t
Cowieson AJ, Ravindran V, Selle PH: Influence of dietary phytic acid and source of microbial phytase on Ileal endogenous amino acid flows in broiler chickens. Poult Sci 2008. 87:2287-2299http://dx.doi.org/10.3382/ps.2008-00096
Engelen AJ, van der Heeft FC, Randsdorp PH, Somers WA: Determination of phytase activity in feed by a colorimetric enzymatic method: Collaboratory study. J AOAC Int 2001,84:629–633.
Fisher DK, Higgins TJ: A sensitive,high-volume,colorimetric assay for protein phosphatases. Pharm Res 1994,11:759-63. http://dx.doi.org/10.1023/A:1018996817529
Greiner R, Carlsson N-G,Alminger ML: Stereospecificity of myo-inositol hexakisphosphate dephosphorylation by a phytate-degrading enzyme of Escherichia coli. J Biotechnol 2000, 84:53-62. http://dx.doi.org/10.1016/S0168-1656(00)00331-X
Greiner R, Farouk A-E:Purification and characterization of a bacterial phytase whose properties make it exceptionally useful as a feed supplement. The Protein J. 2007, 26:467-474.http://dx.doi.org/10.1007/s10930-007-9086-z
Greiner R, Konietzny U, Jany KD. Purification and characterization of two phytases from Escherichia coli. Arch Biochem Biophys 1993,303:107-113. http://dx.doi.org/10.1006/abbi.1993.1261
Jongbloed AW, Mroz Z, Kemme PA: The effect of supplementary Aspergillus niger phytase in diets for pigs on concentration and apparent digestibility of dry matter, total phosphorus, and phytic acid in different sections of the alimentary tract. J Anim Sci 1992,70:1159-1168.
Kemme PA, Lommen A, De Jonge LH, Van der Klis JD, Jongbloed AW, Mroz Z, Beynen AC: Quantification of inositol phosphates using 31P nuclear magnetic resonance spectroscopy in animal autrition. J Agric Food Chem 1999,47:5116-5121. http://dx.doi.org/10.1021/jf981375v
Kies AK, De Jonge LH, Kemme PA, Jongbloed AW: Interaction between protein, phytate, and microbial phytase. In vitro studies. J Agric Food Chem 2006,54:1753-1758. http://dx.doi.org/10.1021/jf0518554
Mulaney EJ, Ullah AHJ: Phytases: attributes, catalytic mechanisms and applications. In Inositol Phosphates: Linking Agriculture and the Environment. Edited by Turner BL, Richardson AE, Mullaney EJ. Wallingford, Oxfordshire, United Kingdom: CAB International;2007:97-110.
Reddy NR: Occurrence, distribution, content, and dietary intake of phytate. In Food Phytates. Edited by Reddy NR, Sathe SK. Boca Raton, FL: CRC Press;2002:25-51.
Reddy NR, Pierson MD, Sathe SK, Salunkhe DK: Interactions of phytate with proteins and minerals. In Phytates in Cereals and Legumes. Edited by Reddy NR, Pierson MD, Sathe SK, Salunkhe DK. Boca Raton, FL: CRC Press;1989:57-70.
Selle PH, Ravindran V: Microbial phytase in poultry nutrition. Anim feed Sci Technol 2007, 135:1-41.http://dx.doi.org/10.1016/j.anifeedsci.2006.06.010
Shi P, Huang H, Wang Y, Luo H, Wu B, Meng K, Yang P, Yao B: A novel phytase gene appA from Buttiauxella sp. GC21 isolated from grass carp intestine. Aquaculture 2008, 275:70–75 http://dx.doi.org/10.1016/j.aquaculture.2008.01.021
Skoglund E, Carlsson NG, Sandberg AS: High-performancechromatographic separation of inositol phosphate isomers on strong anion exchange columns. J Agric Food Chem, 1998, 46:1877-1882.http://dx.doi.org/10.1021/jf9709257
Tran T,Hatti-KaulR, Dalsgaard S, Yu S: A simple and fast kinetic assay for phytases using phyticacid-proteincomplex as substrate. Anal Biochem 2011,410:177-184 http://dx.doi.org/10.1016/j.ab.2010.10.034
Weaver CM, Kannan S: Phytate and mineral availability. In Food Phytates. Edited by Reddy NR, Sathe SK. Boca Raton, FL: CRC Press;2002:211-223.
Wyss M, Brugger R, Kronenberger A, Rémy R, Fimbel R, Oesterhelt G, Lehmann, M, van Loon, APGM: Biochemical characterization of fungal phytases (myo-inositol hexakisphosphate phosphohydrolase): Catalytic properties. Appl Environ Microbiol 1999,65:367–373.
Yi Z, Kornegay ET: Site of phytase activity in the gastrointestinal tract of young pigs. Anim Feed Sci Technol 1996,61:361-368. http://dx.doi.org/10.1016/0377-8401(96)00959-5
Yu S, Cowieson A, Gilbert C, Plumstead P, Dalsgaard S: Interactions of phytate and myo-inositol phosphate esters(IP1-5) including IP5 isomers with dietary protein and iron and inhibition of pepsin. J Anim Sci 2012,90:1824-1832. http://dx.doi.org/10.2527/jas.2011-3866