INTRODUCTION
A healthy gut can help birds to gain optimum growth performance because gastrointestinal tract (GI) is meant for digestion/absorption of nutrients and immune response. The mucosal membrane of the intestine serves as a barrier between luminal digesta and tissues and requires a dynamic balance between different components of gut like epithelial cells, microbiome, and immune system cells for proper functioning. One of the most important components that affect intestinal mucosal barrier is microbiota because gut health is mediated by commensal microbes influencing the development of structure and morphology of gut (Kamada et al., 2013). Microbiome of the intestine is responsible for biosynthesis of different vital nutrients for the host including vitamins especially B vitamins (Hooper et al., 2002; Leblanc et al., 2013) and some essential amino acids. Moreover, they are also responsible for the production of different metabolites from undigested dietary components in the small intestine (B¨ackhed et al., 2005). Microbiota produces butyrate, propionate, and acetate, which provide epithelial cells with energy and also influence mucosal barrier (Topping and Clifton 2001). The beneficial microbiota effectively supports host in the regulation of homeostasis, immune response, organ development and metabolic processes (B¨ackhed, 2013).
The intestinal microbiota has exhibited its unique effects on the development of both innate and acquired mucosal immunity (Gaskins, 1997). Therefore, the second most important functional role of the gut after nutrient absorption is pathogen defense. The gut components that mediate host immune system include an intestinal mucosal layer, epithelial cells, and immunoglobulin A, and antimicrobial peptides. The microbiota is responsible for enhancing intestinal immunity through mediation of toll-like receptor (TLR) expression (Lundin et al., 2008), antigenic response, and lymph follicles (Lee and Mazmanian, 2010; Belkaid and Hand, 2014), as well as by affecting systemic immunity (Noverr and Huffnagle, 2004). The most significant component of intestinal immunity includes a protective covering of mucus gel that contains mucin glycoproteins synthesized and secreted by goblet cells in gut epithelium (Hollingsworth and Swanson, 2004; Dharmani et al., 2009; Forder et al., 2012). This mucin layer promotes the passage rate of digesta and serves as a protective covering against injuries caused by feed, microbes, and their products. This specialized mucin protective covering has two layers in which outer loose layer allows microbial colonization, while inner compact layer inhibits attachment of bacteria acting as first line of defense against pathogenic infection (Brisbin et al., 2008; Johansson et al., 2010). The changes in normal intestinal microbiota have been associated with modification of goblets cells ultimately changing the composition of intestinal mucus. Such mediations in microbial populations and/or host-derived inflammatory mediators are coupled with alteration of expression of mucin thus directly affecting composition or secretion of mucus. Comparison of conventionally reared broiler and germ-free broiler chicks revealed differential mucin profile and increased goblet cells in the intestine (Forder et al., 2007).
Gut microbiome not only modulates antibody-mediated response but also greatly influences cell-mediated immune components. Studies have reported significant effects of complexity of intestinal microbiome on gut T cell repertoire in chicken (Mwangi et al., 2010). For example, Lactobacillus species have exhibited their ability to induce differential cytokine expression in T cells of chicken cecal tonsils that could contribute to intestinal homeostasis (Brisbin et al., 2012). Additionally, a significant decrease in expression of important cytokines (IL-2 and IFN-γ) has been observed in the response of treatment with a blend of probiotic bacteria (L. acidophilus, B. bifidum, and S. faecalis) in Salmonella typhimurium challenged broiler chicken (Haghighi et al., 2008). It is evident from different studies that commensal bacteria of the intestine (at least some of them) significantly affect the immune system of the bird along with pathogenic and probiotic strains. The predominant bacterial species in the gut mainly include: lactic acid bacteria (55%), rods (44%), and pathogens (∼1%). The relative abundance of the microbial community in the gut is affected by many factors that predominantly include feed, age, use of feed additives, and pathogenic infection. Such changes in gut microflora can potentially modulate performance of host by affecting growth rate, immune status, and maturity (Apajalahti and Vienola, 2016). Keeping in view the importance of intestinal microbiome, it seems plausible to evaluate different additives that can affect colonization of intestinal microbiota to improve immune response in chicken. Green tea has shown its potential in improving the antioxidant status and immune function in animals. Polyphenols and amino acids (L-theanine) in green tea have been suggested to have the potential to modulate the immune system and intestinal microflora. Moreover, numerous studies on the administration of green tea (L-theanine) in calves and pigs have exhibited improvement in intestinal microflora balance demonstrated by increased fecal Lactobacillus and decreased Clostridia (Hara et al., 1995; Ishihara et al., 2001). In our previous study, growth performance data of birds revealed that dietary supplementation of L-theanine at a dose rate of 200 mg/kg showed better results in terms of weight gain, meat quality, immune response, and feed conversion ratio (FCR; Saeed et al., 2018). It was hypothesized that improved performance of birds may be a result of modulation of intestinal immunity and microbiome that led to better gut health.
Keeping in view of the above findings, this study was conducted to evaluate the potential effects of different dietary levels of the L-theanine supplementation on the intestinal microbiome and immune response in broilers. To the best of our knowledge, this is the first report of the potential effects of L-theanine on gut microbiome of chicken based on 16S rRNA sequencing.
MATERIALS AND METHODS
Birds, Treatments, and Sampling
A total of 400-d-old broiler chicks (Arbor Acre) were divided into four treatments groups. There were five replicates in each treatment and each replicate had 20 birds. Fresh drinking water and feed were provided ad libitum to all birds throughout the experiment. The total rearing period of 6 wk was sub-divided into two phases: a starter phase (1 to 3 wk) and finisher phase (3 to 6 wk). L-theanine was purchased from a commercial company (Jiangsu Qiang Sheng Chemical Co., Ltd., Shanghai, China with 98% purity) and was mixed in feed mill with different concentrations at 0, 100, 200, and 300 mg/kg feed for the C, 100 LT, 200 LT, and 300 LT treatments groups, respectively. This addition did not change the pH value of diets. Basal diets were formulated according to NRC recommendations and were iso-caloric and iso-nitrogenous (NRC, Rockville, MD, 1994) as shown in Table 1. Chicks were housed in a room having 20 pens of uniform size (3 × 4 sq feet). Pens were whitewashed and disinfected with bromogeramine (1%) before the start of the experiment. For each pen, wood shaving (layer of 3 inches) was used as litter. Ambient temperature was set at 32°C at placement and then decreased gradually to achieve 24°C from week 3 onwards. Lighting was constant on the first day and then changed from day 2 to the end of the study trial to a regime 21 L:3D. Chicks were vaccinated against infectious bursal disease at the age of 8th, 18th, and 28th day while for Newcastle disease at the age of 5th and 18th days (MAFF, 1984). At 21st and 42nd day of rearing, five birds were randomly selected from each treatment group and humanely euthanized. Mucosal contents were aseptically collected from each bird and immediately stored at –20°C and promptly processed. Birds were fasted for 16 h before slaughter. Mucosal samples were scrapped aseptically from ileum (2 cm from Merkel’s diverticulum) and jejunum by sterile glass slides. Pooling of intestinal contents for each age group was performed to reduce individual variation between samples. All samples were immediately stored at −80°C until further laboratory analysis.
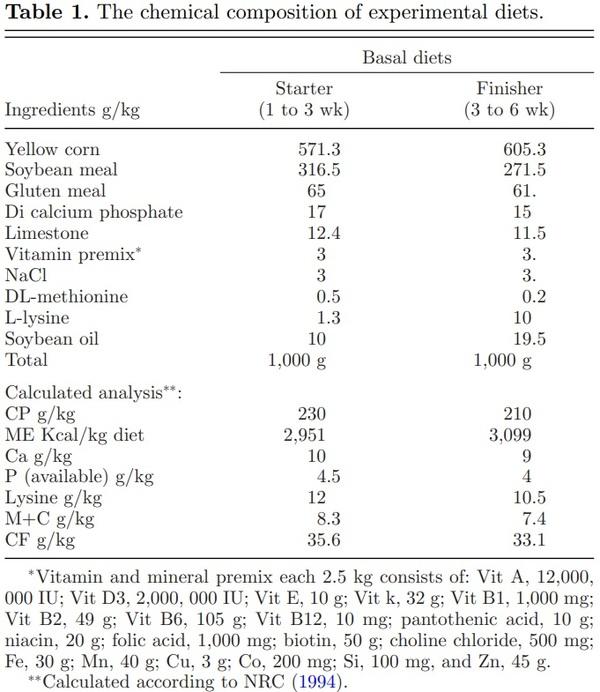
Ethical Statement
The research trial was conducted at the research station of Northwest A & F University. All broiler chicks were managed and treated as per guidelines of the Animal Ethics Committee of Northwest A & F University (T/NWSUAF, 235-2014, 2014-05-09).
DNA Extraction, 16S rRNA Amplification, and Miseq Sequencing
Genomic DNA was extracted by using the QIAamp DNA Stool Mini Kit (QIAGEN, Germany) by following the manufacturer instructions with some modifications. In our previous finding, the performance of birds was better at a dietary L-theanine dose of 200 mg/kg, so further 16S rRNA amplification and sequencing was done to better understanding the reason behind the better growth at a dose of 200 mg/kg. So, further analysis involving 16S rRNA amplification and sequencing was performed to elucidate the effect of dietary L-theanine on intestinal microbiome at a dose rate of 200 mg/kg of diet. Tag Illumina sequencing of a 468 bp in the V3-V4 region of the 16S rRNA gene was performed to construct community library. The bar-coded primers 338F (5-ACTCCTACGGGAGGCAGCA-3′) and 806R (5′-GGACTACHVGGGTWTCTAAT-3′) containing A and B sequencing adaptors were used to amplify this region. The PCR conditions were initial denaturation with 95°C for 2 min, followed by 25 cycles of denaturation at the rate 95°C for the 30 s, annealing at 55°C for 30 s, and extension at 72°C for 45 s, with a final extension phase at 72°C for 10 min. Then different barcode sequences were added at the 5′ end of the forward primer for multiplexed Illumina sequencing performed on a PE300 instrument (Shanghai Major Biopharm Biotechnology Co., Ltd., China) using a MiSeq sequencer platform by Chengdu Life Baseline Technology Co., Ltd., China.
Relative Changes in Lactobacillus as compared to Bactericides
Further to detect the relative changes in Lactobacillus as compared to bactericides, we performed qPCR by using a method of 2−ΔΔCt as reported earlier (Liu et al., 2017).
Data Analysis
Quality control of sequence data was performed to screen16S rRNA sequences for quality and extra nucleotides in beginning and at end of primers were trimmed. The data analysis of sequences was carried out using the QIIME pipeline (Caporaso et al., 2010) and all statistical analyses were performed using R v3.1.0 (R Development Core Team, 2011). Using the software COPE (Connecting Overlapped Pair-End) to carry out sequence splicing, the overlapping relationship between the two pairs of sequences was used to assemble them into a sequence. The comparison of reads between groups and assignment of high-quality sequences to taxonomic groups was carried out using Ribosomal Database Project (RDP) database with a bootstrap cut-off of 80% (Cole et al., 2009). The operational taxonomic unit (OTU)-based analysis including dendrogram, Venn diagrams, and heat maps was performed in Mothur package (using a similarity cutoff of 97% for OTU definition). The abundance information for each OTU was determined to estimate the richness of the microbial species in each sample (Schloss et al., 2009).
Expression of ZO-1, Occludin, and Claudin-3
Reverse transcription of total RNA was performed with M-MLV reverse transcriptase kit that was extracted with TRIpure Reagent kit (Takara, China). Primers were designed as given in Table S3 synthesized by Invitrogen (Shanghai, China). Quantitative PCR was performed in 20 μL reaction containing specific primers, cDNA, and SYBR Premix EX Taq (Takara, China). The levels of mRNA were normalized using β-actin. The relative expressions of ZO-1, occludin, and claudin-3 genes were determined by using a method of 2−ΔΔCt as reported earlier (Liu et al., 2017).
RESULTS
Analysis of Operational Taxonomic Units (OTUs) and Abundance of Intestinal Microbiome
A total number of 16S rRNA amplicon sequences were 303,812 with an average of 37,976 reads per sample. The average length of sequence reads (tags) was 456.5 bp (Table S1). Two approaches were used to analyze the reads including classifying reads with RDP database (Danzeisen et al., 2011) and assigning reads to OTU by using Mothur package. The taxonomic classification of intestinal microbes was determined by optimization of aggregate clusters at 97% similarity for each OUT.
The relative abundance information of OTU for each sample was calculated to determine the richness of the microbial species (Table S2). Using cutoff the value of 97% similarity, a total of 2,442 OTU were identified among eight groups. Relative change in abundance of each OTU was analyzed in jejunum and ileum for treatment groups, as compared to the control groups at 21 d and 42 d of age. Moreover, OTU were sorted by abundance and classified using the RDP database. Results revealed that the overall number of OTU increased with age at 42 d and with a treatment of L-theanine in jejunum and ileum (Table S2). The number of OTU in groups of birds ranged from 230 to 370, which increased with age and treatment of birds with L-theanine. The number of OTU in ileum decreased with age and treatment; however, a higher number of OTU was found in the treatment group at both 21 and 42 d of age as compared to the control group. Similarly, the number of OTU in jejunum decreased with age in the control group; the, however, number of OTU was higher in the treatment group and increased at 42nd day of age (Table S2). This revealed that the number of OTU was significantly higher in the treatment group as L-theanine increased microbial OTU as compared to the control group in both ileum and jejunum at 21 and 42 d of age.
Distribution of OTUs of Intestinal Mucosal Flora using Venn Diagrams
Venn diagrams were constructed to exhibit shared and unique OTU among control and treatment groups at both 21 and 42 d of age in broilers. The analysis of OTU in the ileum showed that 199 OTU (45%) were common between treatment and control groups, whereas 154 OTU (34%) were unique in the treatment group as compared to 94 (21%) in control group at 21 d of age. While at 42nd day of age, shared OTU decreased from 199 (45%) to 104 (23%), while an increase in OTU were observed in both control and treatment groups in the ileum. Moreover, analysis of the jejunum microflora showed the same trend at 21 and 42 d of age (Figure 1). Results revealed that ileum and jejunum-specific OTU were 94,154 and 140,150 (in both control and treatment groups), respectively, at 21 d of age, while at day 42 of age ileum and jejunum-specific OTU were 162, 186, and 152, 276, respectively. With the increase in age of birds from 21 to 42 d, the number of shared OTU decreased from 199 to 104 in the ileum and from 174 to 87 in the jejunum, respectively.
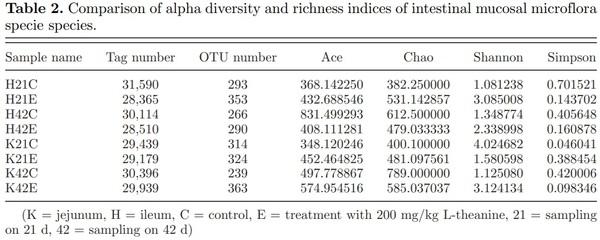
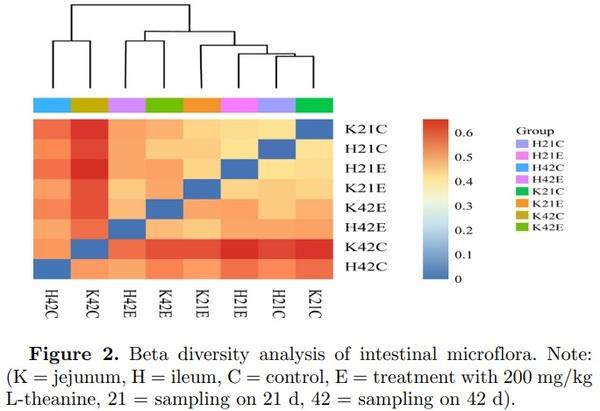
Analysis of Species Diversity Index of Intestinal Mucosal Flora
Alpha diversity analysis is used to study diversity within a specific environment or within a single sample. Common metrics include observed species index, Ace index, Chao index, Shannon index, Simpson index, and so on. Results revealed that species diversity of the jejunum and ileal intestinal mucosal flora increased with age in the control group (Table 2). However, species diversity of the jejunum and ileal mucosal flora did not increase with time in birds fed with L-theanine. The ileal mucosal bacterial community had much fewer speciesequivalent OTU, and a few of them dominated, resulting in low Shannon diversity index and evenness index in control groups at 21 and 42 d of age.
Beta diversity analysis is used to compare the size of a pair of samples in terms of species diversity. The contents of each group in the sample were to calculate the values of beta diversity between different samples. It revealed that the composition of the jejunum in the control and treated group was different at 21 and 42 d of age (Figure 2). The similar pattern of beta diversity was observed in the ileum but diversity values were quite low as compared with the jejunum in this study. It has also been reported earlier that microbial richness and diversity increases with age (Danzeisen et al., 2011).
Changes in the Microbial Community of Intestinal Mucosa in Response to Treatment
The relative abundance of intestinal microbes at each taxonomic level (phylum, class, order, family, and genus) was determined at 21 and 42 d of age in both the control and treatment group. Results revealed the almost linear correlation of microbial abundance with age and treatment with L-theanine. Analysis of 16S rRNA sequences with RDP classifier software and Green Genes database revealed that microbial colonies at the level of the phylum in jejunum mucosa were Actinobacteria, Aminicenantes, Bacteroidetes, Chloroflexi, Firmicutes, Fusobacteria, Lentisphaerae, Proteobacteria, and others. Dietary supplementation with L-theanine at 21 and 42 d of age increased relative abundance of Firmicutes from around 65% to 98%, while a decrease in abundance of Actinobacteria, Bacteroidetes, and Proteobacteria was observed (Figure S1). The relative abundance of Firmicutes decreased in control and treatment group in both ileum and jejunum, and the species diversity of microflora at 42nd day of age was smaller than that of the 21st day of age.
The distribution of microbial classes in ileum and jejunum included mainly: Bacilli, Bacteroidia, Betaproteobacteria, Clostridia, Gammaproteobacteria, Deltaproteobacteria, Epsilonproteobacteria, Erysipelotrichia, Negativicutes, and others (Figure S2). The relative abundance of microbial classes in ileum showed that Clostridia were the main class (> 85%) found at 21 d of age in control group followed by Bacteroidia, Gammaproteobacteria, Negativicutes, Bacilli, and unclassified. However, treatment of Ltheanine decreased relative abundance of Clostridia (∼22%) while increasing Bacilli (∼42%), Bacteroidia, Gammaproteobacteria, Negativicutes, and unclassified as compared to control group at 21 d of age. Contrarily, relative abundance of Clostridia in jejunum was lower (28% vs 85%); however, higher abundance of Bacteroidia (21% vs 5%), Bacilli (25% vs 2%), Gammaproteobacteria (9% vs 2%), Negativicutes (7% vs 1.5%) unclassified (4% vs 1%), and Betaproteobacteria as compared with the control group at 21 d. Similarly, the relative abundance of microbes in jejunum mucosa of birds treated with L-theanine revealed that Bacilli increased significantly (88%) while other bacteria decreased as compared to the treatment group in the ileum. This means that L-theanine showed two-fold increases in abundance of Bacilli in jejunum as compared to ileum in a broiler. At 42 d of age, a relative abundance of Bacilli increased (2% to 58%), while Clostridia decreased (90% to 37%) significantly along with all other bacterial classes in the ileal mucosa (Figure S2). But surprisingly a very high relative abundance of Bacilli (97%) was observed in the treated group that showed a very strong positive effect of L-theanine on microbial abundance in ileum mucosa. Moreover, other classes of bacteria decreased in the treated group of broiler chicken. In comparison with ileum, a microbial abundance of jejunum mucosa revealed that Bacilli increased from 58% to 65%, Clostridia decreased from 37% to 14%, Bacteroidia increased from 2% to 11%, Negativicutes increased from 1.5% to 5.5% at 42 d of age in control group. However, L-theanine-treated group showed depletion of all other bacterial classes as Bacilli colonized in jejunum mucosa by making 99% of the microbial population, thus reducing overall microbial diversity as compared to control group (P < 0.05). This remarkable increase in Bacilli and simultaneous decrease in Clostridia (> 1%) population shows potential modulation of intestinal microbiome by L-theanine, which significantly affects birds health and performance.
The analysis of relative abundance of bacterial orders in ileal mucosa at 21st day of age revealed that Clostridiales decreased (89% to 25%) in treated group as compared to control, while increase in abundance of Lactobacillales (2% to 44%), Bacteroidales (4% to 14%), Selenomonadales (1.5% to 5%), Enterobacteriales, unclassified, and Burkholderiales etc. was observed (Figure S3). In comparison, relative abundance of bacterial orders at 21 d of age in jejunum revealed that Lactobacillales increased (86% to 26%) in treated group as compared to control, while decrease in abundance of Clostridiales (29% to 5%), Bacteroidales (20% to 11%), Enterobacteriales (7% to 1%), unclassified, and Pseudomonadales etc. was observed. Dietary supplementation of L-theanine at 42 d increased the relative abundance of Lactobacillales (57% to 95%), while the abundance of Clostridiales decreased (36% to 3%) Bacteroidales (2% to 1%) and depleted all other bacterial families in the ileal mucosa (Figure S3). In comparison, the relative abundance of Lactobacillales increased (63% to 98%), while the abundance of Clostridiales decreased (13% to 1%) Bacteroidales (2 to 1%) and depletion of all other bacterial families in jejunum mucosa were observed. Dietary supplementation depleted various bacterial taxa of the jejunum mucosa significantly greater than that of the control group (P < 0.05).
Relative abundance of the bacterial families in jejunum mucosa showed that L-theanine treatment resulted in an increase in Lactobacillaceae (23% to 82%), while the decreased relative abundance of Bacteroidaceae, Ruminococcaceae, Lachnospiraceae, Veillonellaceae, Enterobacteriaceae, Rikenellaceae, and Moraxellaceae as compared to control group at 21 d of age (Figure S4). A similar trend was observed at 42 d of age as the relative abundance of Lactobacillaceae increased up to 93%, which resulted in the depletion of other bacterial families as compared to the control group. The overall diversity of the jejunum microbiome in L-theanine group in comparison with the control group was significantly lower (P < 0.05). The almost similar pattern of relative abundance of bacterial families was observed in ileum mucosa of treated birds at 42 d of age as compared to control (Figure S4). But at 21 d of age, some variations were observed in ileal mucosa as compared to jejunum as treatment group of birds showed overall higher diversity in comparison with the control group. However, increase in Lactobacillaceae was also found in ileum mucosa at a lower extent (42% vs 80%) as compared to jejunum mucosa at 21 d of age. Results revealed that L-theanine treatment exhibited more intense effects on jejunum mucosa by depleting major bacterial families and increasing Lactobacillaceae (> 93%) significantly.
Dietary supplementation of L-theanine at 21 d of age decreased relative abundance of Butyricicoccus, Acinetobacter, Sutterella, Clostridium XlVb, Clostridium XlVa, Alistipes, Faecalibacterium, Megamonas, Escherichia/Shigella, and Bacteroides, while relative abundance of Lactobacillus increased (24% to 86%) as compared to control (Figure 4, Figures S5 and S6). The overall diversity of the ileal microbiome in treated birds in comparison with the control group was significantly lower (P < 0.05) at 21 d of age. The relative abundance of Lactobacillus increased (60% to 99%) in treatment group at 42 d of age as compared to control group, while depletion of all other genera of bacteria (Butyricicoccus, Acinetobacter, Sutterella, Clostridium XlVb, Clostridium XlVa, Alistipes, Faecalibacterium, Megamonas, Escherichia/Shigella, and Bacteroides) was observed in ileum. In comparison, jejunum mucosa showed an increased abundance of Lactobacillus (2% to 42%) in the treatment group as compared to control group in which majority of bacterial genera were unclassified (25% vs 86%). However, the relative abundance of Bacteroides, Megamonas, Escherichia/Shigella, Alistipes, Faecalibacterium, Acinetobacter, and Clostridium XlVb increased in the treatment group as compared to control at 21 d of age (Figure 3 Figures S5 and S6). Moreover, at 42 d of age, jejunum mucosa showed a similar pattern of relative abundance in the treatment group as observed in ileal mucosa at 42 d of age as 95% of the bacterial population was Lactobacillus and other genera depleted significantly as compared to control group (P < 0.05).
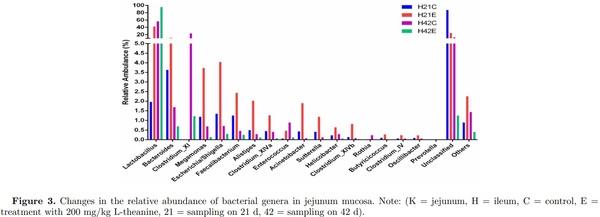
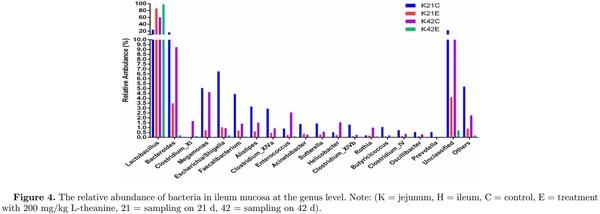
Detection of changes in Lactobacillus and Bacteroides by using qPCR
The number of copies of Lactobacillus and Bacteroides was determined to observe relative changes in both bacterial taxa using qPCR. Results revealed that the number of Lactobacillus increased with age and treatment with L-theanine in both ileum and jejunum (Figure 5). However, the number of Bacteroides decreased with age (at 42 d) and with the treatment of L-theanine in jejunum but increased at 21 d with treatment in ileum but decreased to 42 d in the control group. Moreover, a slight increase in the number of Bacteroides was observed with a treatment at 42 d of age in ileum mucosa.
Changes in mRNA Expression of Mucosal Structural Proteins
Tight junction (TJ) proteins act as the first line of defense (barrier) composed of interconnected intestinal epithelial cells. Disruption of TJ proteins results in impairment of their function as a barrier, leading to increased incidence of inflammation, and thus results in intestinal disorders. In this study, expression of mucosal protein ZO-1 was increased due to dietary supplementation of L-theanine at both 21 and 42 d of age as compared to control group. Similarly, occludin mRNA expression was upregulated by treatment of L-theanine at both 21 and 42 d in broilers as compared to control group. However, expression of claudin-3 was increased at 21 d of age in the treatment group but the nonsignificant effect of treatment was observed at 42 d as compared to control (Figure 6). Previous studies have reported that Bacillus subtilis can improve TJ barrier integrity and protect against disruption of barrier in porcine by decreasing permeability of protein’s layer. Moreover, improvement in the expression of occludin and ZO-1 has been observed during formation of TJ proteins.
Effects of L-theanine on Intestinal Immunity
Adaptive immunity in birds is significantly affected by immuno-regulatory peptides called cytokines. For example, interleukin-12 is responsible for differentiation of na¨ıve T cells into Th-1 cells, which is required for mediation of immune response to enable the host to resist pathogenic invasion (Park et al., 2008). Similarly, another cytokine IFN-γ activates lymphocytes and enhances expression of MHC class II antigens leading to regulation of acquired immunity. Additionally, a high level of IFN-γ has been observed during an immunogenic response against coccidia infection in chicken and is considered a marker of cellular immunity (Lee et al., 2008). Expression of mRNA expression of TLRs and cytokines was determined in the intestine to reveal the influence of L-theanine on the intestinal immune response. Results of this study revealed that mRNA expression of TLRs (TLR-2, TLR-4), TNF-α, IFN- γ, and IL-2 was decreased as result of treatment with L-theanine as compared to control group (Figure 7). As L-theanine also increased relative abundance of Lactobacillus in ileum and jejunum in this study, so correlation coefficient was calculated to determine the relationship of the relative abundance of Lactobacillus with mRNA expression of IL-2 and IFN-γ (Figure 8). It was observed that the increase in abundance of Lactobacillus was negatively correlated with IL-2 and IFN-γ expression in intestine and these effects were highly significant (P < 0.01).
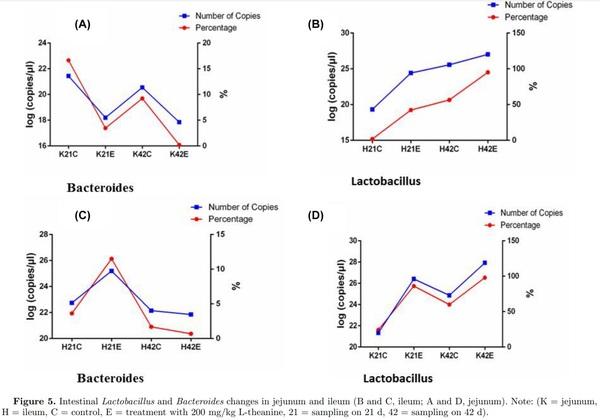
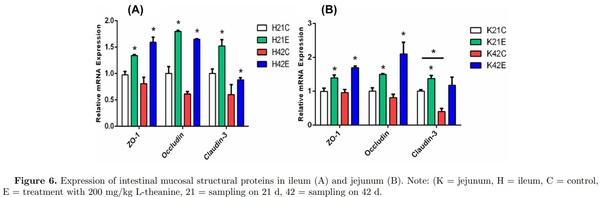
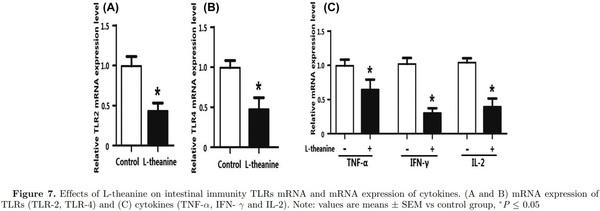
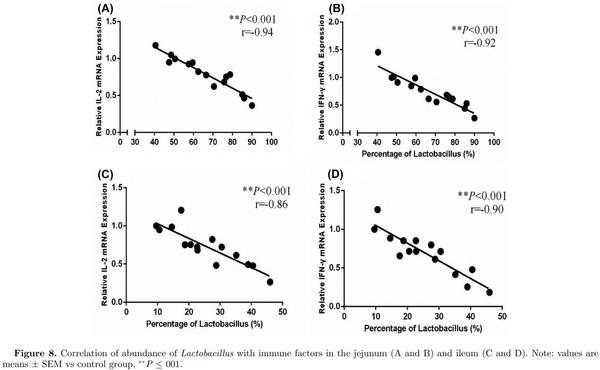
DISCUSSION
Intestinal microbiome in chicken is very diverse and varies individual to the individual being influenced by many intrinsic factors. Relative abundance of different bacterial species is also very divergent across different sections of the GT as a preference for species colonization widely varies among bacterial taxa. Results of the relative abundance of intestinal microflora observed in this study are consistent with earlier researches that reported that predominant phylum of bacteria in the chicken gut is Firmicutes. Their abundance ranges from 50% to 90% of total bacterial population in the ceca (Qu et al., 2008; Danzeisen et al., 2011), while Firmicutes (mainly Lactobacilli) generally form more than 90% of all taxa in other segments of gut like ileum and jejunum (Gong et al., 2007; Rehman et al., 2007; Qu et al., 2008; Danzeisen et al., 2011). A large variation has been observed in microbiota composition between individual broiler chickens. These differences occur even in animals managed under the same dietary and environmental conditions (Stanley et al., 2016). Conditions such as stress, immunological development, and epigenetic factors may be involved, which results in high inter-individual diversity in intestinal microbiota composition. Effect of L-theanine in increasing abundance of Firmicutes is significant in both ileum and jejunum mucosa and exhibits its potential for modulation of the gut microbiome in broilers. Recently, a study (Shaufi et al., 2015) also reported Firmicutes as most abundant bacterial phylum (> 70% of all bacterial sequences) in the ileum of chicken at all ages. They also reported that Firmicutes was also abundant phylum (48%) in caeca of chicken at 42 d of age, which has also been reported previously (Wei et al., 2013). However, a variable abundance of bacteria in fecal samples was observed by Singh et al. (2012) as Proteobacteria was abundant phylum followed by Firmicutes, Bacteroidetes, and Proteobacteria.
The fact that lactobacilli are the dominant and diverse bacteria in the crop, ileum, duodenum, and jejunum has been widely reported in culture-dependent (Fuller, 1997) and independent studies (Guan et al., 2003). Lactobacilli are commonly considered as a sign of healthy gut (Snel et al., 1995) being relatively more abundant in the chicken ileum (Lu et al., 2003). A flow of digesta is more rapid in the proximal part of the intestine (duodenum), which ultimately results in reduced numbers of bacterial harboring on average 103 to 105 cells g−1 of digesta. However, distal small intestine (ileum) harbors a greater number of bacteria (108 to 109 cells g−1 digesta) and possesses a highly diverse bacterial population. Many factors could contribute to a variable composition of intestinal microbes, among which significant factors include the type of feed, species, variable rearing and environmental conditions, and different techniques used for analysis. There are various possible modes of action like competitive exclusion and adjustment of the microbial population like Lactobacillus in intestinal microflora (Fang et al., 2000; Frece et al., 2009). The microbial diversity and structures of community of gut microbial population are reported to be significantly affected by dietary ingredients (Knarreborg et al., 2002; Lu et al., 2003) bird’s age (Lu et al., 2003; Zhu and Joerger, 2003; Amit-Romach et al., 2004) and administration of drugs like antibiotics (Knarreborg et al., 2002; Smirnov et al., 2005). Modulation of intestinal microbiome by L-theanine as revealed by this study provides strong evidence of its influence on body weight gain and immunity of broilers. Dietary supplementation of L-theanine increased relative abundance of Lactobacillus (> 90%) that ultimately made the gut environment conducive for efficient nutrient absorption, metabolism, and better intestinal immunity. L-theanine is a unique amino acid that is only found in tea naturally and is responsible for many physiological roles of green tea (Juneja et al., 1999). The precise mechanism that how Ltheanine manipulated gut microbiota is not clear but these potential effects are beneficial for chicken as a healthy gut is responsible for proper nutrient absorption, utilization, and immune response. L-theanine enhanced expression of occludin and ZO-1 that are normally required for formation of TJ proteins, which are the first line of defense against a pathogenic attack on intestinal epithelium. Both innate and adaptive immunity are critically affected by super family of tumor necrosis factors that modulate inflammatory process, apoptosis, and proliferation of cells (Kaiser and Stäheli, 2008). Supplementation of directly fed microbes containing Bacillus has been reported to modulate expression of immune-related cytokines leading to improved immune response. Decrease in expression of pro-inflammatory cytokines (IL-6) and increase in antiinflammatory cytokine (IL-10) has also been reported in the intestine of chicken fed on probiotic (L. casei, L. acidophilus, B. thermophilum, and E. faecium) supplemented diet for 21 d (Chichlowski et al., 2007). Similarly, treatment with L. fermentum reduced the TLR2 level, without affecting TLR4. Various studies have reported mediation of immune functions in response to supplementation of diet with Lactobacilli (Lopez et al., 2008; Llopis et al., 2009). These results imply that beneficial microbiota containing Lactobacilli can regulate intestinal immunity and modulate cytokines and TLR levels to ameliorate inflammation (Cao et al., 2013).
L-theanine modulates immunity and growth response by exerting two-fold effects; one by favoring beneficial gut microbiota by increasing their relative abundance that reduces pathogenic load by competitive exclusion. At the same time, L-theanine directly reduces the expression of mRNA of TLRs (TLR-2, TLR-4), and inflammatory cytokines (TNF-α, IFN-γ, and IL-2) as observed in this study. Earlier reports have also demonstrated this ability of L-theanine to inhibit stress-induced inflammatory response by decreasing serum TNF-α and IL-1β levels in mice (Jiang et al., 2012). Moreover, it was also observed that L-theanine is potentially responsible for downregulation of cytochrome P450 2E1 (CYP2E1) along with improving the anti-oxidant ability of cells affected by carbon tetrachloride (CCl4) toxicity. Theanine is not an anti-oxidant but enhances the activity of anti-oxidant enzyme activities and increases the concentration of glutathione (GSH). Many studies have shown favorable role of L-theanine on immune system as revealed by epidemiological studies and experiments on cell cultures, animals and humans (Kim et al., 2008; Chattopadhyay et al., 2011; D’Arena et al., 2013; Kuo et al., 2014; Huijun wang et al., 2014). After oral intake of L-theanine, it is absorbed in blood through the gut and hydrolyzes in glutamic acid and ethylamine in liver and intestine (Tsuge et al., 2003; Scheid et al., 2012). This ethylamine is capable of the proliferation of γδ T lymphocytes that are subsets of T-cells and act as the first line of defense in case of pathogenic attack as reported in a clinical study (Rowe et al., 2007). So these potential effects of L-theanine by enhancing proliferation of T lymphocytes while decreasing expression of pro-inflammatory response mediate immune response effectively. Results revealed that L-theanine-mediated growth and immune response in broiler by modulation of intestinal microbiome that ultimately influences better nutrient utilization and metabolism along with positively influencing intestinal immunity. This mediation of intestinal microbiome was also coupled with the reduction of pathogenic bacteria in gut and regulation of expression of inflammatory cytokines and TLRs in the intestine that provided two-fold responses in terms of growth and immune response. However, further studies are required to explore the mechanism of modulation of intestinal microbiota and T lymphocyte-mediated immune response in chicken by L-theanine. The graphical presentation of our major findings of the study is illustrating in Figure 9.
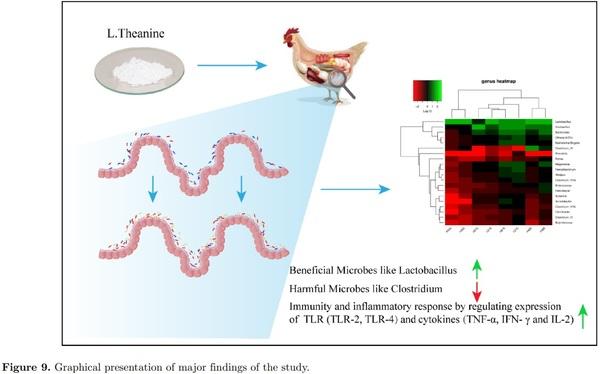
In summary, dietary treatment of L-theanine showed promising effects on the distribution of intestinal microbiome by enhancing relative abundance of beneficial taxa like Lactobacillus while decreasing harmful bacteria like Clostridium. L-theanine showed its potential to modulate intestinal mucosal immune response and ameliorate inflammation by changing expression levels of pro-inflammatory cytokines and TLRs. Supplementation of dietary L-theanine improved the integrity of the TJ barrier by enhancing expression of ZO-1, occludin, and claudin-3 in the intestine. Moreover, L-theanine showed its ability to modulate intestinal immunity and inflammatory response by regulating the expression of cytokines and TLRs. However, further studies are required to explore the mechanism of modulation of intestinal microbiota and T lymphocytemediated immune response in chicken by L-theanine.
This article was originally published in 2018 Poultry Science 0:1–13. http://dx.doi.org/10.3382/ps/pey394. This is an Open Access article under a Creative Commons license.