Introduction
Infectious diseases continue to pose significant economic losses to the animal industry. The routine use of antibiotics at subtherapeutic doses for disease prevention in animal production practices is suspected to be a major force that drives the emergence of antibiotic-resistant pathogens [1,2]. Therefore, it is imperative to develop novel antimicrobial strategies with a low risk of triggering resistance. Dietary compounds with the capacity to augment HDP synthesis and host immunity are attractive candidates as alternatives to antibiotics for disease control and prevention [3]. As a critically important first line of defense, HDPs are produced mainly by both mucosal epithelial cells and phagocytes in vertebrate animals [4,5]. These peptides are broadly active against a range of pathogens including antibiotic-resistant strains. Unlike conventional antibiotics with no immune regulatory functions, HDPs are capable of modulating the host immune system by recruiting and activating different types of immune cells [6]. Additionally, most HDPs have the capacity to dampen the bacterial infection-induced inflammatory response, because of their strong affinity for bacterial membrane components such as lipopolysaccharides [7].
Defensins and cathelicidins represent two major families of HDPs in vertebrate animals [8,9]. Multiple members of each family generally exist in most animal species. For example, 13 bdefensins and 11 cathelicidins have been reported in pigs [10,11]. Porcine b-defensin 1 (pBD1), pBD2, pBD3, pBD129, and epididymis protein 2 splicing variant C (pEP2C) are expressed in a wide range of tissues, whereas pBD4, pBD123, and pBD125 are primarily restricted to the male reproductive tissues [12,13]. Eleven members of porcine cathelicidins include proline/argininerich peptide of 39 amino acids (PR-39), cysteine-rich protegrins (PG) 1–5, proline/phenylalanine-rich prophenins (PF) 1–2, and porcine myeloid antimicrobial peptides of 23, 36, and 37 amino acids, also known as PMAP-23, PMAP-36, and PMAP-37, respectively [10,11].
Porcine cathelicidins are mainly derived from bone marrow myeloid cells, albeit with a restricted expression in many other tissues [10,11]. Besides infection and inflammation, HDPs have been found to be specifically regulated by several different dietary compounds. For example, vitamin D3 is a potent inducer of human cathelicidin LL-37 [14,15]. Several di- and monosaccharides such as lactose, trehalose, maltose, glucose, and galactose were revealed recently to stimulate LL-37 synthesis in human cells [16]. Essential amino acids including isoleucine and arginine are capable of enhancing HDP expression in humans, cattle, and pigs as well [17–20]. A trace mineral, namely zinc, has been found to augment HDP expressions in both human and porcine intestinal epithelial cells [20,21]. Moreover, probiotic lactobacilli potentiate HDP expression in human cells and piglets [22,23]. Butyrate, a major species of short-chain fatty acids (SCFAs) that are produced by bacterial fermentation of undigested dietary fiber in the large intestine, has a strong capacity to induce HDP expression in humans, rabbits, cattle, and chickens [24–27]. Several analogs of butyrate were further revealed to process a similar LL-37-inducing activity in human cells [28]. A comparison of the HDP-inducing activity among all saturated free fatty acids of different hydrocarbon chain lengths indicated that SCFAs are the most potent, with butyrate having the peak HDP-inducing activity in chicken cells [29]. Collectively, these studies raised the possibility of augmenting HDP synthesis as a novel antimicrobial approach for both humans and livestock animals [3].
However, not all animal species show a heightened production of HDPs in response to the aforementioned dietary compounds, and species-specific regulation of HDP synthesis exists. For example, vitamin D3 enhances HDP expression in humans, but not mice, rats or dogs [14]. Instead of promoting HDP production, butyrate and its derivatives are incapable of inducing mouse cathelicidin CRAMP in RAW264.7 macrophage cells (Zhang, G., et al., unpublished data) and even suppress CRAMP expression in mice following intraperitoneal administration [30]. Moreover, individual HDPs are known to be differentially regulated by dietary compounds. Among all 14 b-defensins and 4 cathelicidins in chickens, approximately a half number of HDPs are obviously induced in response to butyrate, although the magnitude of induction varies markedly among genes and cell types [26]. On the other hand, vitamin D3 was shown to induce LL-37 and bdefensin 2, but not several other defensins that were tested [14,15].
In this study, we examined whether butyrate and its analogs have the ability to stimulate HDP gene expression in pigs. Three different porcine cell types including IPEC-J2 intestinal cells, 3D4/ 31 macrophage cells, and primary monocytes were used to evaluate possible regulation of a majority of known porcine HDPs in response to butyrate. Furthermore, a panel of saturated free fatty acids with 1–18 carbons as well as butyrate analogs was examined for their HDP-inducing activity in porcine IPEC-J2 cells. We revealed that a majority, but not all, porcine HDPs can be regulated by butyrate. We also identified several butyrate analogs with a strong capacity to induce HDP expression and great potential to be further explored as alternatives to antibiotics for animal agriculture.
Materials and Methods
Ethics Statement
This study was carried out in strict accordance with the recommendations in the Guide for the Care and Use of Laboratory Animals of the National Research Council. Animal procedures reported herein were approved by the Institutional Animal Care and Use Committee of Oklahoma State University.
Chemicals
Sodium formate (C1), sodium acetate (C2), sodium propionate (C3), sodium butyrate (C4), valeric acid (C5), sodium hexanoate (C6), sodium n-octanoate (C8), sodium decanoate (C10), transcinnamyl butyrate, benzyl butyrate, glyceryl tributyrate (also known as tributyrin), 5-phenylvaleric acid, sodium 4-phenylbutyrate, hydrocinnamic acid (also known as 3-phenylpropanoic acid), 2-phenylacetic acid, and sodium benzoate were purchased from Sigma-Aldrich (St. Louis, MO), whereas sodium heptanoate (C7), sodium nonanoate (C9), sodium dodecanoate (C12), sodium tetradecanoate (C14), and sodium octadecanoate (C18) were from TCI America (Portland, OR). SCFAs (sodium formate, acetate, propionate, butyrate, and valeric acid), medium-chain fatty acids (sodium hexanoate, heptanoate, n-octanoate, nonanoate, and decanoate), sodium 4-phenylbutyrate, and sodium benzoate were dissolved in RPMI 1640 medium, whereas long-chain fatty acids (sodium dodecanoate, tetradecanoate, and octadecanoate) were dissolved in methanol, and benzyl butyrate, glyceryl tributyrate, trans-cinnamyl butyrate, and hydrocinnamic acid were dissolved in ethanol. On the other hand, 5-phenylvaleric acid and 2- phenylacetic acid were dissolved in dimethyl sulfoxide (DMSO).
Cell Culture and Treatment
Porcine intestinal epithelial cell line, IPEC-J2 [31], was kindly provided by Dr. Bruce Schultz at Kansas State University (Manhattan, KS), whereas porcine lung alveolar macrophage cell line, 3D4/31 (ATCC #CRL-2844), was purchased from American Type Culture Collection (Manassas, VA). IPEC-J2 cells were cultured in 6-well plates at 1 x 106 cells/well in DMEM/F12 medium supplemented with 10% fetal bovine serum (FBS), 100 mg/ml streptomycin, 100 U/ml penicillin, and 20 mM HEPES, pH 7.4. 3D4/31 cells were cultured in 6-well plates at 1 x 106 cells/well in RPMI 1640 medium supplemented with 10% FBS, 100 mg/ml streptomycin, 100 U/ml penicillin, and 1 mM sodium pyruvate. After overnight growth, cells were treated in duplicate with various chemicals. All chemicals were first tested for their cytotoxicity. A broad range of sub-toxic concentrations of the chemicals were then used to determine their effect on HDP gene expression, and only an optimal HDP-inducing range of concentrations were presented for each compound. A series of time-course experiments were also conducted with sodium butyrate to determine the optimal duration of stimulation. All experiments were repeated at least 2–3 times. None of the solvents including methanol, ethanol, and DMSO showed any appreciable effect on HDP expression (data not shown).
Porcine Primary Monocyte Isolation and Stimulation
Acid citrate dextrose-anticoagulated blood was freshly collected from the jugular vein of approximately 30- to 35-day-old pigs by venipuncture. Mononuclear cells were isolated by density gradient centrifugation using Histopaque 1077 (Sigma), followed by hypotonic lysis of the red blood cells, if needed. Mononuclear cells were then resuspended in RPMI 1640 medium supplemented with 10% FBS, 100 mg/ml streptomycin, 100 U/ml penicillin, and 20 mM HEPES and plated in 6-well plates at 1.5 x 107 cells/ well. After overnight at 37°C and 5% CO2, non-adherent cells were aspirated, and the plated were washed twice with PBS. Adherent cells, namely monocytes, were cultured in the same medium for mononuclear cells for 2 h prior to stimulation with different doses of sodium butyrate in duplicate for 24 h.
Analysis of Porcine Gene Expression by Real Time RT-PCR
After stimulation, cells were lysed directly in RNAzol RT (Molecular Research Center, Cincinnati, OH). Total RNA was extracted according to the manufacturer’s instructions. RNA concentrations were measured by Nanodrop. The first-strand cDNA was synthesized using QuantiTect Reverse Transcription Kit (Qiagen). Real-time PCR was performed using QuantiTect SYBR Green PCR Kit (Qiagen) in 10-ml reactions with 1/20 (for GAPDH) or 1/10 (for HDPs) of the first-strand cDNA and gene-specific porcine HDP primers (Table 1) as we previously described [26,29,32,33]. MyiQ Real-Time PCR System (Bio-Rad) was used, and the PCR program was set for initial denaturation at 95°C for 10 min, followed by 40 cycles of 94°C for 15 sec, 55°C for 20 sec, and 72°C for 30 sec. The specificity of PCR amplification was confirmed by the melt curve analysis. Relative gene expression was calculated according to the DDCt method using porcine glyceraldehyde-3-phosphate dehydrogenase (GAPDH) as the reference gene as described [26,29,32,33].
Statistics Analysis
Statistical analyses were performed using GraphPad PRISM 5 (GraphPad Software, La Jolla, CA). All pairwise comparisons were examined using unpaired Student’s two-tailed t-test. Differences were considered significant when P<0.05.
Results
Sodium Butyrate Stimulates HDP Gene Expression in Different Porcine Cell Types
Sodium butyrate is a strong inducer of HDP genes in humans, rabbits, cattle, and chickens [24–27], but not in mice [30]. To test whether butyrate could increase the HDP gene expression in pigs, we performed both dose-response and time-course experiments in porcine IPEC-J2 intestinal epithelial cells and then analyzed the expressions of multiple porcine HDP genes by real-time RT-PCR. The genes that were examined included all 11 known cathelicidins (PG1-5, PF1-2, PR-39, PMAP-23, PMAP-36, and PMAP-37) and seven b-defensins with adequate expressions in epithelial tissues (pBD1, pBD2, pBD3, pBD4, pBD123, pBD125, pBD129, and pEP2C) [12,13]. The b-defensins that are lowly expressed in most tissues (e.g., pBD104 and pBD114), as well as those that are highly specific to male reproductive tissues (e.g., pBD108), were excluded from the current study. It is noted that, because of a high sequence similarity among five protegrins, the primers were designed to simultaneously target all five members (PG1-5), rather than individual ones. The same is true for the primers for two prophenins (PF1-2).
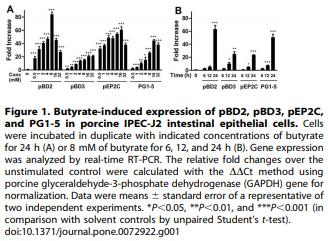
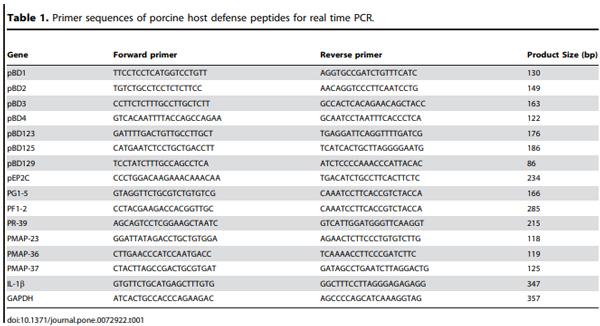
Our results indicated that a 24-h treatment with sodium butyrate markedly increased pBD2 expression in a dose-dependent manner in IPEC-J2 cells, peaking at 8 mM (Fig. 1A). Similarly, the expression levels of pBD3, pEP2C, and PG1-5 were also dose-dependently induced by butyrate in IPEC-J2 cells, with the maximal response occurring at 16 mM (Fig. 1A). However, the magnitude of induction varied obviously among the four genes, with pBD2 showing an approximately 85-fold increase, and pBD3, pEP2C, and PG1-5 showing 20-, 60-, and 45-fold induction at the peak response (Fig. 1A). An obvious time-dependent induction of pBD2, pBD3, pEP2C, and PG1-5 was also observed in IPEC-J2 cells following 8 mM butyrate treatment (Fig. 1B). A maximal response occurred at 24 h, whereas 6 and 12 h treatments only showed a marginal effect, which is consistent with earlier observations in human and chicken cells [24,26].
PMAP-23, PMAP-37, and PR-39 were also evidently induced in IPEC-J2 cells in response to butyrate treatment; however, because of our inability to detect the basal expression levels of these genes in unstimulated cells, the data were not included in the analysis. It is noteworthy that pBD1 expression was readily detected, but remained largely unaltered in IPEC-J2 cells following butyrate treatment (data not shown). We failed to detect the expressions of pBD4, pBD123, pBD125, pBD129, PF1-2, and PMAP36 in IPEC-J2 cells before and after butyrate treatment (data not shown).
To further examine whether butyrate is capable of stimulating the porcine HDP expression in other cell types, porcine 3D4/ 31lung alveolar macrophage cells were treated with different doses of butyrate for 24 h. A dose-dependent augmentation of pBD2, pBD3, and PG1-5 was observed (Fig. 2A), although the induction patterns of the three genes were in sharp contrast with those in IPEC-J2 cells (Fig. 1A), where pBD2 was more markedly upregulated by butyrate than pBD3. In contrast, pBD3 showed much more dramatic change in 3D4/31 cells, with a nearly 190- fold increase following 8 mM butyrate treatment, whereas pBD2 was only marginally induced by less than 10-fold at any dose applied. PG1-5 showed an intermediate 42-fold increase in 3D4/ 31 cells in response to 8 mM butyrate (Fig. 2A). Like in IPEC-J2 cells, PMAP-37 was obviously induced in 3D4/31 cells by butyrate; however, we could not calculate relative fold induction because of an inability to detect its expression in untreated cells. The expression of pBD1 remained unchanged in 3D4/31 cells in response to butyrate (data not shown). Other HDP genes such as pBD4, PMAP-36, and PR-39 could not be detected in 3D4/31 cells before or after butyrate treatment.
Butyrate-induced expression of porcine HDPs was further confirmed in primary monocytes. A 24-h treatment of primary monocytes with sodium butyrate dose-dependently enhanced the mRNA expression of pBD2, with 2 or 4 mM butyrate giving an approximately 150-fold increase, in comparison with the control (Fig. 2B). However, we could not reliably detect the expression of pBD3, PG1-5, and multiple other HDP genes in porcine primary monocytes.
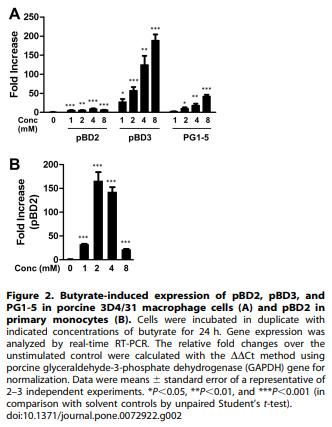
Butyrate is known to be anti-inflammatory and has been used to treat inflammatory bowel diseases [34]. To examine the influence of butyrate on the expression of a major proinflammatory cytokine, interleukin (IL)-1b, real-time RT-PCR was performed on both IPEC-J2 cells and primary monocytes. IL-1b was not detectable in IPEC-J2 cells either before or 6, 12 or 24 h after 8 mM butyrate treatment, whereas a minimal 2.5-fold increase was seen in primary monocytes 24 h after stimulation with 8 mM butyrate (data not shown), suggesting that butyrate has a minimal impact on the inflammatory response in porcine cells, which is reminiscent of our earlier observations in chickens, where IL-1b and IL12p40 expressions were largely unaffected by butyrate in HD11 macrophage cells [26]. Because of the fact that SCFAs are naturally produced in the intestinal tract and quickly metabolized and our expectation that SCFAs mainly work locally in the digestive tract rather than systemically in the circulation, IPEC-J2 cells were chosen for all subsequent studies.
Fatty Acid Chain Length is Correlated with the HDPinducing Activity of Free Fatty Acids
We previously showed that butyrate is the most potent in inducing HDP gene expression among all free fatty acids of different hydrocarbon chain lengths in chickens [29]. To compare the relative HDP-inducing efficacy of saturated free fatty acids in pigs, IPEC-J2 cells were treated first with a broad range of doses of different fatty acids with the chain length varying from C1 to C18 for 24 h, and the cytotoxicity was examined as we previously described [35–37]. Generally, fatty acids showed an enhanced toxicity as the aliphatic chain length increased (data not shown). Different doses of the subtoxic doses of fatty acids were then evaluated for their effect on the induction of pBD2, pBD3, and pEP2C by real-time RT-PCR. Obviously, each free fatty acid showed a largely dose-dependent induction of all three HDP genes tested (Fig. 3).
Furthermore, there was a clear correlation between the chain length and the HDP-inducing efficacy among all free fatty acids. For the fatty acids with 4 carbons or less, the HDP-inducing activity was gradually enhanced as the chain length increased; however, a length-dependent decrease in the HDP-inducing activity was observed for the free fatty acids with more than 4 carbons (Fig. 3). The longer the fatty acid, the weaker is its ability to induce HDP expression. Fatty acids with 3–8 carbons possessed a strong HDP-inducing activity with an obvious induction of pBD2, pBD3, and pEP2C. The peak response occurred with sodium butyrate (C4), whereas formate (C1) and long-chain fatty acids (LCFAs) ($ C12) showed only a marginal effect on regulating HDP gene expression (Fig. 3). Collectively, these results are consistent with our earlier observation in chicken HD11 macrophage cells and primary monocytes, where butyrate exhibited the most potent HDP-inducing activity [29].
Butyrate Analogs Differentially Enhance HDP Expression
Because of an unpleasant smell and a quick metabolic rate, butyrate is not a particularly attractive compound for in vivo applications [3]. Odorless and/or structurally rigid analogs of butyrate have stronger potential. Several butyrate analogs including 4-phenylbutyrate and a-methylhydrocinnamate were recently shown to be comparable to butyrate in HDP induction in human cells [28]. To identify the butyrate analogs with a potent activity in stimulating HDP expression in pigs, IPEC-J2 cells were treated for 24 h with different doses of benzyl butyrate, glyceryl tributyrate, and trans-cinnamyl butyrate (see their chemical structures in Fig. 4A). The expressions of pBD2, pBD3, pEP2C, and PG1-5 were then analyzed by real-time RT-PCR. All three analogs triggered an evident dose-dependent increase in the expressions of all four HDP genes (Fig. 4B).
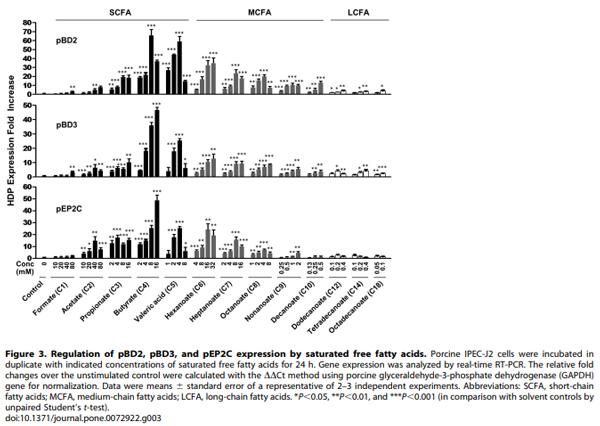
At the optimal HDP-inducing dose, glyceryl tributyrate, an odorless esterified derivative consisting of three butyric acids, showed a comparable potency to sodium butyrate in inducing all four HDPs, with a less than 2-fold difference in the peak response (Fig. 4B). Benzyl butyrate, a common ingredient of fragrances with a fruity smell, was also approaching the efficiency of butyrate in regulating pBD2, pBD3, and pEP2C at 4 mM. However, benzyl butyrate was 4-fold less efficient in stimulating PG1-5 expression than butyrate at the optimal dose (4 mM). Trans-cinnamyl butyrate was obviously weaker in inducing all four HDPs than butyrate. It is particularly the case in the induction of PG1-5. An approximately 4-fold maximal increase in the PG1-5 expression was observed with trans-cinnamyl butyrate at 2 mM, whereas a 42- fold peak increase was seen with butyrate at 8 mM (Fig. 4B). Even at 2 mM, butyrate triggered a 25-fold increase in the expression of PG1-5, suggesting that butyrate analogs vary greatly in their ability to regulate HDP gene expression.
Impact of the Aromatic Ring on SCFA-induced HDP Expression
Sodium 4-phenylbutyrate is more efficient than butyrate in inducing HDP expression in human lung epithelial cells [28], but comparable to butyrate in several chicken cell lines (data not shown). To examine the impact of the aromatic ring on SCFA-induced HDP expression in pigs, IPEC-J2 cells were stimulated for 24 h with different doses of free SCFAs (C1-C5) or their corresponding analogs with a phenyl group attached at the end of the aliphatic chain (see their structures in Fig. 5A). It became apparent that the presence of a phenyl group substantially increased the toxicity of SCFAs to IPEC-J2 cells, except for butyrate and propionate, which showed a similar toxicity to 4- phenylbutyrate and hydrocinnamic acid, respectively (data not shown). Subtoxic doses of phenyl derivatives of SCFAs were then used to identify the optimal concentration range to induce pBD2, pBD3, pEP2C, and PG1-5 by real-time RT-PCR. Similar to free SCFAs, all phenyl derivatives showed a chain length-dependent HDP expression, with 4-phenylbutyrate (C4) being the most potent. For those derivatives consisting of an aliphatic chain length of 4 carbons or less, the HDP-inducing activity decreased as the chain shortened (compare among solid bars in Fig. 5B). On the other hand, the opposite was true between 4-phenylbutyrate and 5-phenylvaleric acid (C5). Overall, 4-phenylbutyrate, hydrocinnamic acid (C3), and 5-phenylvaleric acid showed an obvious induction of all four porcine HDPs examined, whereas 2- phenylacetic acid (C2) and sodium benzoate (C1) exhibited largely a marginal effect on HDP expression.
When compared between a free SCFA and its corresponding phenyl derivative, the influence of the phenyl group on the HDPinducing activity varied markedly among individual pairs. For example, at the identical concentrations, 4-phenylbutyrate was more efficient than butyrate in inducing pBD2 expression, with more than 2-fold in difference in the peak response. In contrast, butyrate was generally 2-fold more efficient than 4-phenylbutyrate in pBD3 induction at each concentration tested. On the other hand, 4-phenylbutyrate and butyrate were largely comparable in their efficacy in inducing the expressions of pEP2C (Fig. 5B) and PG1-5 (data not shown). In the case of valeric acid and 5- phenylvaleric acid, the former was invariably more potent in inducing all four HDPs than the latter. The same trend held true between acetate and 2-phenylacetic acid, with the free fatty acid showing an augmented HDP expression. Between propionate and hydrocinnamic acid, the presence of a phenyl group obviously enhanced pBD2 expression, but suppressed pEP2C expression with a minimal impact on pBD3 expression. Collectively, the phenyl group had a mixed effect on HDP induction that varied obviously among individual genes and SCFAs.
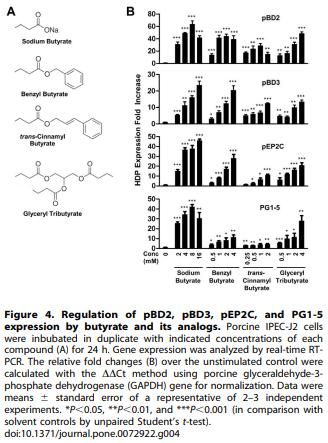
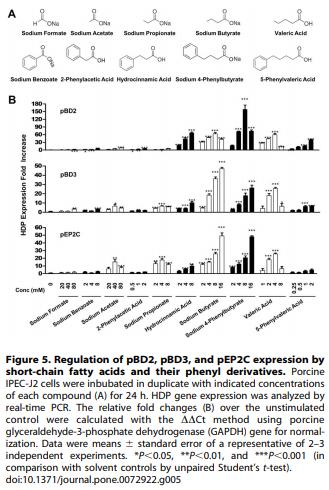
Discussion
Although many dietary compounds possess the HDP-inducing activity, species-specific differences clearly exist at least in the case of vitamin D3 and butyrate [14,30]. It is thus necessary to examine the ability of individual compounds to induce HDPs in each species. In this study, we have confirmed that butyrate is a strong inducer of multiple b-defensin and cathelicidin genes in different porcine cell types. It is known that LL-37 mRNA and protein expressions are concurrently augmented in humans and rabbits following butyrate administration [25,38], and that butyrate-induced HDP mRNA expression is associated with an enhanced antibacterial activity of monocytes and bacterial clearance in chickens [26]. It is still important to confirm the up-regulation of porcine HDPs at the protein levels, particularly in vivo, and the subsequent effect on disease resistance and pathogen reduction in pigs in the future.
In addition to species-specific regulation of HDPs by butyrate, gene- and cell-type specific regulation patterns are also evident. First, not all HDP genes are regulated by butyrate. Among all porcine HDPs that were analyzed, pBD1 is clearly un-inducible in any cell type. Secondly, for those HDP genes that are induced by butyrate, each is differentially regulated, with a different magnitude of induction in any given cell type. For example, in 3D4/31 macrophages, pBD3 was induced by 188-fold, whereas pBD2 and PG1-5 were increased only by 6.6- and 42-fold, respectively, in response to 8 mM butyrate (Fig. 2A). Thirdly, the same HDP gene may be regulated differently in different cell types. The expression of pBD2 was enhanced by 84-fold in IPEC-J2 cells following 8 mM butyrate treatment (Fig. 1A), yet a minimum 6.6-fold induction was seen in 3D4/31 macrophages (Fig. 2A). Such a gene- and cell-type specific regulation of porcine HDPs was also clearly demonstrated in humans and chickens [26,39]. It is thus important to verify the HDP regulation in different cell types.
Butyrate is the main SCFA derived from fermentation of undigested dietary starches and fiber in the large intestine of animals [40,41]. Together with other SCFAs, butyrate exerts pleiotropic effects on the pathophysiology of intestinal functions. The molecular mechanisms by which butyrate induces LL-37 gene expression have been investigated in human cells. Butyrate-mediated LL-37 induction has been found to be associated with hyperacetylation of core histones H3/H4 and a non-histone protein, high mobility group nucleosomal binding domain 2 (HMGN2) in intestinal epithelial cells [42], which could, in turn, cause DNA to be loosened up and a set of genes including LL-37 to be transactivated. In line with this, several histone deacetylase inhibitors such as trichostatin A and sulforaphane are indeed capable of inducing HDP expression in human cells [42,43]. Additionally, mitogen-activated protein kinases, transforming growth factor b type 1-receptor kinase, and vitamin D receptor are all involved in butyrate-induced LL-37 expression in human colonic epithelial cells [44]. It is important to confirm the mechanisms of butyrate-induced porcine HDP expression in the future. On the other hand, vitamin D3-mediated LL-37 induction primarily relies on translocation and binding of vitamin D receptor to the vitamin D response element on the gene promoter [14,15] and histone hyperacetylation is beneficial as vitamin D3 and histone deacetylase inhibitors such as butyrate synergizes in augmenting LL-37 expression [45].
A comparison of the HDP-inducing potency among saturated free fatty acids of various chain lengths revealed a ‘‘bell-shaped’’ curve in porcine IPEC-J2 intestinal epithelial cells, where fatty acids comprised of 3–8 carbons are highly potent, with butyrate being the most efficacious (Fig. 3). The results are reminiscent of our earlier data in chickens, where SCFAs, and butyrate, in particular, showed the most dramatic HDP induction in HD11 macrophages and primary monocytes [29]. However, although LCFAs (≥12 carbons) exhibit a negligible effect on HDP induction in porcine intestinal epithelial cells, a low but obvious HDP induction was observed in chicken primary monocytes [29]. On the other hand, stimulation of human sebocytes with LCFAs such as lauric acid (C12:0), palmitic acid (C16:0), and oleic acid (C18:1, cis-9) drastically induced human b-defensin 2 expression, but not b-defensin 1, b-defensin 3 or cathelicidin LL-37 [46]. The results collectively implied the magnitude of HDP expression induced by different fatty acids is species-, cell type- and/or gene-dependent. In comparison with saturated LCFAs, unsaturated fatty acids appear to be stronger in inducing HDP expression in chicken monocytes [29]. It will be interesting to investigate the impact of the saturation status on the HDP-inducing activity of fatty acids in pigs.
Although 4-phenylbutyrate is more potent in LL-37 induction than butyrate in human VA10 bronchial epithelial cells [28], it is only true with pBD2 in porcine IPEC-J2 intestinal epithelial cells. In fact, a comparable efficacy in inducing three other porcine HDPs, namely pBD3, pEP2C, and PG1-5, was observed between 4-phenylbutyrate and butyrate, indicating a gene-specific response (Fig. 5B). Moreover, there is no clear pattern on the impact of the phenyl group on the HDP-inducing activity among free SCFAs. Phenyl SCFA derivatives showed varied efficacies in HDP induction as compared with the corresponding free SCFAs even for the same HDP gene (Fig. 5B). We also revealed that, in addition to 4-phenylbutyrate, glyceryl tributyrate and benzyl butyrate showed a comparable efficacy in the induction of all four porcine HDPs (Fig. 4B). The ability of these compounds to enhance HDP expression in porcine intestinal epithelial cells is particularly beneficial for strengthening the mucosal antimicrobial barrier, especially in newborn and weaning piglets, which have an immature immune system, are susceptible to infections, and often suffer from diarrhea and growth retardation if infected [47].
Sodium 4-phenylbutyrate is an odorless butyrate analog that has been approved for treatment of urea cycle disorders and is under investigation for multiple other clinical indications [48]. Glyceryl tributyrate (also known as tributyrin) is another odorless butyrate prodrug with more favorable pharmacokinetic properties than butyrate [49]. On the other hand, benzyl butyrate is a commonly used ingredient in fragrances with a pleasant plum smell and extensive animal toxicity data [50]. In conclusion, identification of these butyrate analogs with favorable odors, known safety features, and/or improved pharmacokinetic properties will expedite their application as non-antibiotic, immune boosting dietary additives for infectious disease control and prevention in pigs and perhaps in other animal species including humans as well.
Acknowledgments
We thank Dr. Bruce Schultz at Kansas State University for kindly providing porcine IPEC-J2 intestinal epithelial cell line.
Author Contributions
Conceived and designed the experiments: GZ. Performed the experiments: XZ LTS WJ MB SC XM GZ. Analyzed the data: XZ GZ. Wrote the paper: XZ SQ GZ.
This article was originally published in PLoS ONE 8(8): e72922. doi:10.1371/journal.pone.0072922. This is an Open Access article distributed under the terms of the Creative Commons Attribution License.