Summary
Management of the sow herd has direct and indirect effects on the performance of their progeny and consequently, on herd feed conversion (HFC). Reducing sow replacement rate has a small direct effect on HFC due to changes in breeder feed consumption. However, the indirect impact of the associated reduction in the proportion of gilts and their progeny in the breeding herd has a large effect on HFC. Compared with the progeny of pluriparous sows, the progeny of gilts are lighter at birth and weaning and are more susceptible to disease.
The indirect effect of reduced pre- and postweaning mortality and growth associated with a reduction in the proportion of gilts in a breeding herd with a low turnover is equivalent to a 10% reduction in HFC. Thus, improvement of reproductive performance and sow turnover will improve HFC. The consequences of birth weight on progeny performance and replacement gilt reproductive performance are discussed with reference to recent research findings.
Introduction
The reproductive performance of sows in the breeding herd is critical for the success of pig production. As, the sow herd accounts for 20% of feed costs in most piggeries, it has less of a direct impact on total herd feed conversion (HFC) than the grower-finisher population. However, sow productivity has a substantial impact on the throughput of market pigs and on the utilization of space and associated fixed costs. Sow productivity is influenced by genetics, parity, seasonal infertility, nutrition and health. During the past decade, much effort has been devoted to improving sow productivity, especially by increasing sow longevity and lifetime performance. Although some herds in Australia are able to wean 26 pigs per sow per year (Close et al., 2005), the average Australian herd weans 19.7 pigs per sow per year, which is similar to the average for all other countries except Denmark (23.5 pigs weaned per sow per year(Davidson, 2005). It is economically sound to keep sows in the breeding herd for as long as possible.
Theoretically, if a sow produces an average of 11.0 liveborn piglets per litter over a lifetime of nine parities,she should produce about 100 piglets in total. However, sows in most production herds produce 30-40 piglets during their lifetime in the herd (Close et al., (2005).
A high sow turnover rate has a direct effect on breeder feed consumption because replacement gilt must be maintained for 6-12 weeks before their first mating. In addition, it is now recognized that the ability of gilts to sustain foetal development in utero and to support the growth and immune competence of their progeny during lactation affects the subsequent health and growth of the progeny. Improvements in sow longevity and the growth and health of their progeny will improve HFC.
Direct effects of sow replacement rate on herd feed efficiency
Most sows are culled because of low reproductiveperformance. In a review of sow longevity, Hughes and Varley (2003) concluded that sow replacement rate has not improved over time, whereas other sow productivity traits such as litter size have improved steadily as a result of advances in genetics (Bunter, 2009) and management (Thorup, 2009). The average sow replacement rate in Australia is 49%, and ranges from 16% to 68% (APL, 2010). Thus, a target sow replacement rate of 40%, equivalent to 14% gilt matings, can be achieved by many piggeries in Australia. The heritabilities of breeding traits that determine sow longevity have recently been quantified, enabling the emphasis within breeding programs to be adjusted.
Traits such as leg conformation and soundness and sow retention to parity 2 are moderately heritable (Rathje, 2007). When producers retain more gilts to maintain mating volume, HFC (feed consumed per unit carcass weight produced) is increased (less efficient) because of increased breeder feed consumption or reduced output of pigs per year. In addition to reproductive performance, mortality and management decisions such as destocking and repopulating for health or genetic reasons affect sow turnover. A lower sow replacement rate due to improved reproductive performance and lower mortality will improve HFC (Table 1).
Most of the cost of maintaining extra gilts or sows to sustain a constant mating budget is associated with the space required to house them, which may reduce the space available for the finisher herd, and with the purchase cost of replacement gilts. A high stocking rate in the mating shed may result in a high frequency of anoestrous, especially during summer and autumn.
Gilt litters and the mortality and growth of their progeny
A high sow turnover rate is associated with an increase in the proportion of litters born to gilts. Gilts generally have a low litter size and farrowing rate (Hughes and Varley, 2003; Levis, 2005), which can reduce the number of pigs weaned. However, in commercial production units, the number of matings is adjustedaccording to a desired weaner production rate. A lower sow replacement rate and improved reproductive performance will reduce the need for replacement gilts and consequently, reduce the proportion of piglets born to gilts. The output of weaners would also increase, as the preweaning mortality of the progeny of multiparous sows is less than that of the progeny of gilts because the former have a more developed immune system and shed fewer pathogens (Holyoake, pers. comm.).
The progeny of gilts are smaller at birth than the progeny of multiparous sows (Gatford et al., 2010; Miller, 2006). Progeny of gilts also experience greater postweaning morbidity, which affects survival and weight gain to market age (Holyoake, 2006; Larriestrar et al., 2002; Moore, 2001). In a Pork Co-operative Research Centre (CRC) investigation into factors affecting pre- and postweaning survival and growth, we showed that sow parity and piglet birth weight are significant factors contributing to weaning weight and survival, and that they ultimately determine the lifetime growth and survival of the progeny. Sow parity accounted for 26% of the variation in birth weight and 12% of the variation in weaning weight. We also observed that 27% of deaths between weaning and finisher age were due to low weight at birth. Much research has been conducted by the Pork CRC on ways to increase the birth weights of piglets born to gilts.
Progeny of gilts are 200 g lighter at birth than progeny of sows (Gatford et al., 2010; Geale et al., 2009; Miller, 2006) and grow about 13% slower, with the result that they are 1 kg lighter than the progeny of sows at weaning at 26 days of age (Geale et al., 2009; Smits and Collins, 2009). This weight difference increases as the pigs approach market age (Table 2), which is consistent with the results of (Dunshea et al., 2003).
Progeny of gilts have a greater mortality rate than progeny of sows in commercial herds. We observed significant differences between the progeny of gilts and sows in mortality before weaning and during the weaner (4-10 weeks of age) and grower (11-16 weeks) phases (Table 3; Smits and Collins, 2009). There was also a trend for lower mortality among the progeny of sows than among the progeny of gilts during the finisher phase.
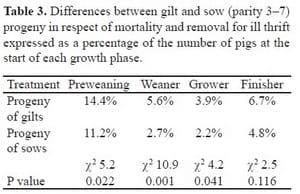
Miller (2006) showed that the progeny of gilts grew slower than the progeny of sows irrespective of birth weight differences and suggested that this difference may be associated with differences in immune competence, and thus, susceptibility to disease. In a subsequent experiment, they demonstrated that gilts transfer less IgG to their progeny during lactation than sows and suggested that this could explain why the progeny of gilts are more susceptible to disease than the progeny of sows (Miller et al., 2009). The composition of the colostrum (Geale et al., 2009) and milk (Beyer et al., 2007; Geale et al., 2007) of gilts differs from that of older sows. Although (Beyer et al., 2007) reported that the milk yield of gilts was less than that of multiparous sows, there were small differences in litter weightgain between gilts and older sows in studies in which demand for milk by the piglets was equal (Boyce et al., 1997; Smits and Collins, 2009). Thus, birth weight and neonatal health are likely to determine the growth and health of the progeny over their lifetime and to affect HFC through their effects on mortality and sale weight.
Table 4 shows that the direct effects of sow replacement combined with the indirect effects of a reduced proportion of progeny of gilts in the herd are equivalent to 10% of HFC.
Effects of increased litter size on progeny growth and development Across all parities, an improvement in reproductive performance in traits such as litter size and farrowing rate will increase the number of progeny produced per year and hence defray the costs and feed usage of the breeder herd. Genetics, nutrition, management and health have interrelated effects on the number of weaners produced per sow per year. An annual genetic increase in litter size of 0.07 pigs born alive has been achieved, as has a corresponding increase in phenotypic litter size (Hermesch, 2006). The potential negative effects of selection for more prolific sows on birth weight, foetal development and growth have been investigated (Foxcroft et al., 2006; Town et al., 2004; Vonnahme et al., 2002). In some selection indexes, this effect has been addressed by including birth weight and the number of piglets surviving to 5 days of age as variables (Stumpf et al., 2011). Breeding companies are now recognizing that the number of progeny that survive until weaning is an important economic trait, although it is difficult to measure accurately when fostering is practiced.
Advances in nutrition have also contributed to improvements in litter size. Examples of these include the inclusion of ractopamine in lactation diets (van Wettere et al., 2009), dietary inclusion of betaine (van Wettere and Herde, 2009), supplemental folate and vitamin B12 in diets of gestating sows (van Wettere, unpubl.), omega-3 polyunsaturated fatty acid supplementation during lactation (Smits et al., 2011b) and during lactation and early gestation (Smits et al., 2011a) and reduced suckling demand in parity 1 litters (Hewitt et al., 2009).
Foetal and neonatal programming for lifetime performance
Regardless of whether litter size is increased by genetic or nutritional means, there is increasing evidence that increased litter size has adverse consequences for the growth and development of the progeny. The foetal programming effect of litter size has not been widely investigated, but it has been established that uterine capacity can limit foetal nutrient uptake and growth, resulting in slower postnatal development as well as lower birth weight (Foxcroft et al., 2006; Town et al., 2004). It has recently been shown that the environment (stalls vs pens) in which future breeding sows are reared and their neonatal litter size (≤7 vs ≥10) have carry-over effects on their reproductive performance.
Estienne (Estienne, 2011) reported that fewer female progeny born to gilts reared in gestation stalls reached puberty by 165 d of age than female progeny born to gilts reared in group pens during gestation. The neonatal health status of replacement gilts has also been observed to influence their breeding performance 30 weeks later (Table 5).
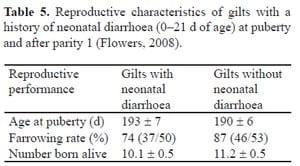
Flowers (2008) reported that first-litter sows are especially vulnerable to the drain of lactation of body reserves in that parity 2 litter size is inversely related to first-litter weaning weight. This finding is supported by the study of (Hewitt et al., 2009), in which a reduction in litter size and thus use of body reserves for lactation in first-litter sows increased parity 2 litter size. Flowers (2011) presented the results of a large research project in which on-farm observations were conducted to determine whether neonatal growth has a lifetime effect on reproductive performance. Future breeding gilts born to litters consisting of 10 to 12 piglets were manipulated by allocating them within 24 h of birth to groups of either ≤7 piglets or ≥10 piglets during lactation, after which they were managed identically until mating. Gilts from the small lactation litters had greater preweaning growth rates and weaning weights than those from the large lactation litters. These traits were significantly correlated with sow longevity, and birth weight tended to be correlated (P < 0.10) with sow longevity. After six parities, 38% of the sows that were reared in the small litter group during lactation remained in the herd compared with only 16% of those reared in the large group during lactation. The average farrowing rate of sows that were reared in the small litter group during lactation was significantly higher than that of sows that were reared in the large group during lactation (88.7% vs 83.3%, respectively), as was the average number of piglets born alive to them (11.0 vs 10.5, respectively).
These results provide evidence of the impact of the early neonatal growth of the sow and gestational housing and stressors on the reproductive potential of gilts selected for breeding.
Foetal development and neonatal growth also have consequences for progeny destined for slaughter. Compared with piglets with normal birth weight, light birth-weight piglets have lower muscle mass, grow slower and less efficiently postweaning and tend to have fatter carcases as finishers (Gatford et al., 2010; Gondret et al., 2006). Dunshea et al. (2003) showed that pigs with a 0.5 kg higher birth weight were 13 kg heavier at 23 weeks of age, but had a backfat level similar to pigs born light and sold at the same age.
There are few reports on the effects of birth weight or weaning weight on progeny feed efficiency. In a Pork CRC project to investigate the lifetime performance of light weaners, Morrison et al. (pers. comm.) observed that heavy weaners had a 7% greater FCR (i.e., were 7% less efficient) than light weaners (P < 0.05), which decreased to a tendency (P = 0.10) for a 4% greater FCR during the grower-finisher period. This finding was somewhat surprising, but may be due to assessment of feed efficiency according to age rather than live weight.
In their Pork CRC project on the use of recombinant somatotropin (pST) to increase birth weight, Gatford et al. (pers. comm.) also evaluated progeny feed efficiency based on age. They also found there was a trend for light birth-weight piglets to be more efficient than their medium and heavy birth-weight counterparts (Table 6).
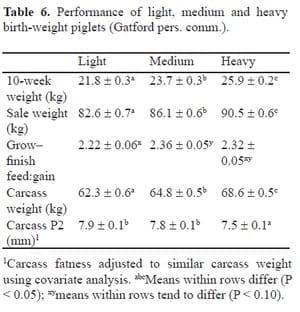
The results of Morrison et al., who evaluated feed efficiency based on age, may have differed had they assessed feed efficiency between standardized live weights (i.e., between 60 kg and 90 kg live weight). As the general commercial practice is to sell pigs at a targeted market weight, feed efficiency between the grower and finisher weight ranges would be more appropriate than efficiency traits assessed between ages when assessing the relationship between birth weight or weaning weight on progeny feed efficiency.
The number of foetal muscle fibres during early to mid-gestation determines postnatal lean tissue mass. Piglets with low birth-weight as a result of uterineovercrowding (Foxcroft et al., 2007) are likely to have low numbers of myofibres that hypertrophy abnormally (structurally abnormal fibres of extreme size) during postnatal growth (Rehfeldt and Kuhn, 2006). The carcasses of low birth-weight progeny are fatter and meat from these carcasses is less tender and has greater drip loss (Gondret et al., 2006). Gatford et al. (2004) investigated the possibility of treating the dam with pST during gestation to reduce the incidence of low birth-weight piglets. They found that treatment of gilts with pST from day 25 to day 100 of gestation increased birth weight, whereas short-term pST treatment (days 25-50) did not. In a follow-up experiment, they again showed that long-term treatment was successful in increasing birth weights of progeny of gilts and sows, but litter size was decreased by the treatment (Gatford et al., 2010). Gatford et al. (2004) reported that maternal dietary protein level did not affect foetal development or the birth weight of the progeny. In other studies, manipulation of the composition of diets fed during gestation has not affected birth weight (Bee, 2004; Dwyer et al., 1994) or weaning weight (Mahan, 1998).
Changes in gestational feeding level and nutrition may elicit greater responses in sow herds in which low birthweights occur because of uterine crowding. The effects of birth weight and weaning weight on sale weight and HFC are presented in Table 7. The predicted consequence of progeny growth on carcass weight sold, and consequently HFC demonstrates the importance of progeny weight in production systems. Note that HFC does not account for the adverse effects of high growth rate or carcass weight on carcass fat penalty or stocking rate. However, in terms of HFC, improvement of growth rate, either through increased birth weight or weaning weight, has the potential to produce substantial benefits.
Conclusion
Sow herd management affects progeny performance and HFC. Although sow replacement rate has a direct effect on feed consumption per breeder, its greatest effect is an indirect effect associated with the proportion of progeny of gilts on pre- and postweaning health and growth rate. There is evidence that piglets with low birth-weights because of impaired gestational development havelow slaughter weights and are fat. Recently, low birth weight and poor neonatal growth were found to have
repercussions for the breeding potential of replacement gilts.
Acknowledgements
Financial assistance from the Australian Pork CRC for funding of several projects where data was sourced in preparation for this manuscript is gratefully acknowledged.
References
APL (2010). Production Benchmarking Project. Australian Pork Limited. Canberra, ACT.
Bee G (2004) Effect of early gestation feeding, birth weight, and gender of progeny on muscle fibre characteristics of pigs at slaughter. Journal of Animal Science 82, 826-836.
Beyer M, Jentsch W, Kuhla S, Wittenburg H, Kreienbring F, Scholtze H, Rudolph PE, Metges C (2007) Effects of dietary energy intake during gestation and lactation on milk yield and composition of first, second and fourth parity sows. Archives of Animal Nutrition 61, 452-468.
Boyce JM, Smits RJ, Campbell RG, King RH (1997) The interrelationship between parity and litter weight on the milk production of sows. In: Manipulating Pig Production VI, (ed. Cranwell PD) p. 74. Australasian Pig Science Association, Locked Bag 4, Bentley Delivery Centre, WA 6983, Australia.
Bunter KL (2009) Managing consequences of increasing litter size: A genetic perspective. In: Manipulating Pig Production VII (ed. van Barneveld RJ) pp. 149-156. Australasian Pig Science Association, Locked Bag 4, Bentley Delivery Centre, WA 6983, Australia.
Close WH, Turnley K, Mullan BP (2005) Achieving production and economic targets on farm: The Premier Pig Program. In: Manipulating Pig Production X (ed. Paterson JE) p. 166. Australasian Pig Science Association, Locked Bag 4, Bentley Delivery Centre, WA 6983, Australia.
Davidson AA (2005) The use of baseline production data in order to benchmark New Zealand with key international trading partners. In: Manipulating Pig Production X (ed. Paterson JE) p. 166. Australasian Pig Science Association, Locked Bag 4, Bentley Delivery Centre, WA 6983, Australia.
Dunshea FR, Kerton DK, Cranwell PD, Campbell RG, Mullan BP, King RH, Power GN, Pluske JR (2003) Lifetime and postweaning determinants of performance indices of pigs. Australian Journal of Agricultural Research 54, 363-370.
Dwyer CM, Stickland NC, Fletcher JM (1994) The influence of maternal nutrition on muscle fibre number development in the porcine fetus and on subsequent postnatal growth. Journal of Animal Science 72, 911-917.
Estienne MJ (2011) Gilt productivity based on her mother''''''''''''''''s experiences. Journal of Animal Science 89(ed. van Barneveld RJ), 9.
Flowers WL (2008) New ideas about gilt development and management. http://www.gov.mb.ca/ agriculture/livestock/pork/pdf/bab19s06.pdf .Accessed 8 April 2011.
Flowers WL (2011) Preweaning management of replacement gilts and sow longevity. Journal of Animal Science 89(ed. van Barneveld RJ), 8.
Foxcroft, G R, Bee G, Dixon WT, Hahn M, Harding JE, Patterson JL, Putman CT, Sarmento S, M. Smit, Tse W-Y, Town SC (2007) Consequences of selection for litter size on piglet development. Paradigms in Pig Science, Nottingham, UK:207-229.
Foxcroft GR, Dixon WT, Novak S, Putman CT, Town SC, Vinsky MDA (2006) The biological basis for prenatal programming of postnatal performance. Journal of Animal Science 84 (e-supplement), E105-E112.
Gatford KL, Boyce JM, Blackmore K, Smits RJ, Campbell RG, Owens PC (2004) Long-term, but not short-term, treatment with somatotropin during pregnancy in underfed pigs increases the body size of progeny at birth. Journal of Animal Science 82,
93-101.
Gatford KL, Smits RJ, Collins CL, Argent C, De Blasio MJ, Roberts CT, Nottle MB, Kind KL, Owens JA (2010) Maternal response to daily maternal porcine somatotropin injections during early-mid pregnancy or early-late pregnancy in sows and gilts. Journal of Animal Science 88, 1365-1378.
Geale PF, Miller YJ, Dhand N, Holyoake PK, Sheehy PA, Wynn PC (2009) Rearing dam parity affects piglet preweaning growth rate. In: Manipulating Pig Production XII (ed. van Barneveld RJ) p. 45. Australasian Pig Science Association, Locked Bag
4, Bentley Delivery Centre, WA 6983, Australia.
Geale PF, Thomson PC, Wynn PC, Sheehy PA (2007) Differences in colostrum and milk composition between gilts and sows. In: Manipulating Pig Production XI (eds Paterson JE, Barker JA) p. 49. Australasian Pig Science Association, Locked Bag
4, Bentley Delivery Centre, WA 6983, Australia.
Gondret FL, Lefaucheur L, Juin H, Louveau I, Lebret B (2006) Low birthweight is associated with enlarged muscle fiber area and impaired meat tenderness of the longissimus muscle in pigs. Journal of AnimalScience 84, 93-103.
Hermesch S (2006) From genetic to phenotypic trends. In: AGBU Pig Genetics Workshop, pp. 59-65. Animal Genetics and Breeding Unit, University of New England, Armidale, NSW, Australia.
Hewitt RJE, Chick S, van Barneveld RJ (2009) The effect of diet density and lactation demand during the first lactation on subsequent reproductive performance. In: Manipulating Pig Production XII (ed. van Barneveld RJ) p. 141. Australasian Pig Science Association, Locked Bag 4, Bentley Delivery Centre, WA 6983, Australia.
Holyoake PK (2006) Dam parity affects the performance of nursery pigs. In: International Pig Veterinary Society Conference, Denmark, p. 149.
Hughes PE, Varley MA (2003) Lifetime performance of the sow. In: Perspectives in Pig Science (eds Wiseman J, Varley MA, Kemp B) pp. 333-355. Nottingham University Press, Nottingham, UK.
Larriestrar AJ, Wattanaphansak S, Neumann J, Bradford J, Morrison R, Deen J (2002) Pre-existing conditions as predictors of mortality and slow growth in nursery pigs. In: Allen D Leman Swine Conference, Minneapolis, Minnesota, pp. 231-
233.
Levis DG (2005) Biological and economical evaluation of sow longevity. In: Manipulating Pig Production X (ed. Paterson JE) pp. 171-179. Australasian Pig Science Association, Locked Bag 4, Bentley Delivery Centre, WA 6983, Australia.
Mahan, DC (1998) Relationship of gestation protein and feed intake level over a five-parity period using a high-producing genotype. Journal of Animal Science. 76:533-541.
Miller YJ, Collins AM, Smits RJ, Begg D, Emery D, Holyoake PK (2009) Effect of dam parity on maternal transfer of specific IgG into colostrum and milk following vaccination with tetanus toxoid. In: Manipulating Pig Production XII (ed. van Barneveld RJ) p. 100. Australasian Pig Science Association, Locked Bag 4, Bentley Delivery Centre, WA 6983, Australia.
Miller YV (2006) Improving the performance of Progeny of gilts. In: Proceedings of the Australian Pig Veterinarians Conference, Melbourne, p. 64. Australian Veterinary Association, Unit 40, 6 Herbert Street, St Leonards, NSW 2065.
Moore C (2001) Segregated production: How far could we go? In: Allen D Leman Swine Conference, Minneapolis, Minnesota, p. 203. University of Minnesota.
Rathje TA (2007) Future breeding policies from an applied perspective. I In: Paradigms in Pig Science(eds. Wiseman J, Varley MA, McOrist S, Kemp B) pp. 245-261. Nottingham University Press, UK.
Rehfeldt C, Kuhn G (2006) Consequences of birth weight for postnatal growth performance and carcass quality in pigs as related to myogenesis. Journal of Animal Science 84 (e-supplement), E113-E123.
Smits RJ, Collins CL (2009) Progeny reared by their birth dam do not outperform progeny crossfostered to a similar parity dam. In: Manipulating Pig Production XII (ed. van Barneveld RJ) p. 143. Australasian Pig Science Association, Locked Bag
4, Bentley Delivery Centre, WA 6983, Australia.
Smits RJ, Luxford BG, Mitchell M, Nottle MB (2011a) Sow fertility is improved by feeding diets supplemented with omega 3 fatty acids from fish oil during lactation through to early pregnancy. Journal of Animal Science 89 (e-supplement 2), 71.
Smits RJ, Luxford BG, Mitchell M, Nottle MB (2011b) Sow litter size is increased in the subsequent parity when lactating sows are fed diets containing omega 3 fatty acids from fish oil. Journal of Animal Science (in press).
Stumpf TT, Rathje TA, Sonderman JP (2011) Danbred North America. ASAS Midwest Animal Science Conference 89 (e-supplement 2), 8.
Thorup F (2009) Piglet survival in large litters. In: Manipulating Pig Production XII (ed. van Barneveld RJ) pp. 168-172. Australasian Pig Science Association, Locked Bag 4, Bentley Delivery Centre, WA 6983, Australia.
Town SC, Patterson JL, Pereia CZ, Gourley G, Foxcroft GR (2004) Embryonic and foetal development in a commercial dam-line genotype. Animal Reproduction Science 85, 301-316.
van Wettere WHEJ, Herde P (2009) Supplementing gestation diets with betaine increases litter size of summer mated sows. In: Manipulating Pig Production XII (ed. van Barneveld RJ) p. 140. Australasian Pig Science Association, Locked Bag
4, Bentley Delivery Centre, WA 6983, Australia.
van Wettere WHEJ, Pain SJ, Hughes PE (2009) Supplementary ractopamine during lactation reduces sow weight loss and improves reproductive performance. In: Manipulating Pig Production XII (ed. van Barneveld RJ) p. 46. Australasian
Pig Science Association, Locked Bag 4, Bentley Delivery Centre, WA 6983, Australia.
Vonnahme KA, Wilson ME, Foxcroft GR, Ford SP (2002) Impacts on conceptus survival in a commercial swine herd. Journal of Animal Science 80, 553-559.
This article was originally published in Recent Advances in Animal Nutrition- Australia Volume 18 (61-67). Engormix.com thanks the author and the organizing committe for this huge contribution.