Introduction
The growth, development and functionality of the gastrointestinal tract (GIT) is a dynamic and evolving process that prepares the young pig, both before and after parturition, for its future growth, development and ultimately, survival. The perinatal development of the GIT can be divided into three phases: the prenatal phase, characterized by minimal stimulation from the GIT lumen; the neonatal phase, associated with changes caused predominately by colostrum and milk intake; and the post-weaning phase, associated with marked changes to, and the adaptation of, the GIT to solid feed (Pluske, 2016). This review focuses only on the neonatal phase.
Discussion of the impacts of colostrum and milk composition on post-natal gastrointestinal tract development and production is highly relevant to the worldwide pork industry mainly because there has been a steady increase in litter size in the last years, commensurate at times with little or no change in pre-weaning survival. Between 1990 to 2010, litter size on average increased from 11 to 14 piglets born per sow per litter with some countries reaching an average more than 16 piglets. In hyperprolific sow lines, it is not uncommon to have litters up to 18–20 piglets, or more, born alive (summarised in Oliviero et al., 2019). This increased number of piglets has become a major challenge for both sow physiology during pregnancy, at parturition and during lactation, as well as for postnatal growth and survival. Larger litters have a direct impact on the piglets at birth, with the higher the number of piglets born in a litter resulting in both a lower piglet birthweight and a higher variation in piglet birthweight within the litter (summarised in Oliviero et al., 2019). A greater number of piglets born than the available teats at the sow's udder, a lower birthweight and a greater birthweight variation all increase the piglets’ competition for colostrum intake (Declerck et al., 2017). A reduced colostrum intake in the first 24 hours of life has negative effects on piglet survival (Devillers et al., 2011), especially for piglets of low birth weight and low weight gain, and hence is one of the major causes of neonatal mortality. Similarly, lower birthweight and long farrowing duration are associated with lower piglet vitality at birth, which can delay the access to the udder (e.g., Islas‐Fabila et al., 2018). Furthermore, and in larger litters, an increased number of piglets born with signs of intrauterine growth retardation is also described (e.g., Matheson et al., 2018).
Newborn piglets are born with functionally mature innate immunity, but without the protection of immunoglobulins because of the epitheliochorial nature of the placenta and with polarised Th2‐type immunity. The Th2‐type immunity is mediated by high levels of progesterone and Th2 cytokines produced in the maternal–foetal interface (Oliviero et al., 2019). Neonate piglets must therefore procure maternal immunoglobulins from ingested colostrum for passive immune protection, before they start to adequately produce their own immunoglobulins at approximately 3–4 weeks of age (Rooke & Bland, 2002).
Colostrum and milk composition
Colostrum is obviously an important secretion of the sow and hence its composition typically reflects the physiological biology of the sow at the time, and the evolutionary requirements of her progeny (Pluske et al., 1995). As described originally by Klobasa et al. (1987), and seemingly with little major changes since this time (Theil & Hurley, 2016; Zhang et al., 2018), colostrum total solids and protein contents in the first 6 hours of lactation were higher, while fat and lactose contents were lower, than in mature milk. Decreased total protein and whey protein contents and the parallel increased fat and lactose contents, with a nearly unchanged total solids level, indicates the compositional changes associated with the transition from colostrum to mature milk. The high protein content of colostrum is largely due to immunoglobulin (Ig), and indeed and during the first 6 hours of life, IgG accounts for nearly all of the protein in colostrum but plays a decreasing role in sow milk as lactation proceeds. After 2 weeks of life, IgA levels begin to increase and at the end of lactation, IgA constitutes ~40% of the total whey protein (Figure 1).
More recently, Picone et al. (2018) used a 1H–NMR-based metabolomics approach to explore colostrum compounds with a maximum 10 kDa molecular weight in three pig breeds: Large White, Landrace and Duroc, respectively. Colostrum sampling was performed during parturition, after the birth of the first piglet and before the parturition of the last, and across all teats. A total of 25 metabolites were identified, representing monosaccharides, disaccharides (such as lactose), organic acids (lactate, citrate, acetate and formate), nitrogenous organic acids (such as creatine) and other compounds including nucleotides. Principal Components Analysis showed clustering due to breed and seasonal effects, with lactose being the main compound determining the assignment of samples into different clusters according to sow breed. Among the identified metabolites, acetate and taurine showed positive effects on piglets’ performances from birth to day 3 of age and on piglets’ survival rate, while dimethylamine and cis-aconitate exerted a negative influence on the new-borns’ capacity to survive. Nevertheless, a preparation step for the analysis eliminated immunoglobulins and other proteins with high molecular weight, hence the absence of their well-known effects in this particular study.
Figure 1. Relative total amounts and proportions of Ig G, A, and M in sow mammary secretions during lactation. Circles for 6 hours through ≥12 days represent the total Ig content in mammary secretions compared with the content at 0 hours (after Hurley, 2015).
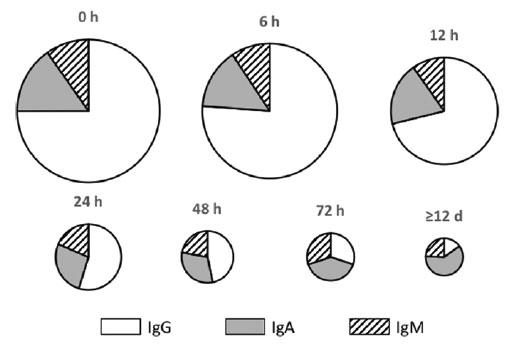
Traditionally, porcine milk has been considered to be mainly composed of carbohydrates, lipids, and proteins (immunoglobulins, caseins and whey) with small proportions of minerals, vitamins, leukocytes and somatic cells. Proteins in sow mammary secretions include those associated with the milk fat membranes, caseins, mammary-derived whey proteins, immunoglobulins, hormones and growth factors, enzymes, and a wide range of other proteins. Concentrations of most milk-specific proteins typically are lower in colostrum than in milk, while concentrations of immunoglobulins and other bioactive proteins often are enriched in colostrum compared with mature milk (Theil & Hurley, 2016). In this regard, the plethora of (largely uncharacterised) compounds such as growth factors, hormones, cells, exosomes, oligosaccharides and antimicrobial factors including lactoferrin, lysozyme, lactoperoxidase, and cytokines (e.g., IL-1β, IL-6, TNF-α, IFN-γ and IL-1ra), which in one way or another are involved in the stimulation and regulation of the growth and development of the GIT after birth (Pluske, 2016), represents an area of research that is currently largely overlooked. These antimicrobial and immunomodulatory components of porcine milk have been suggested to compensate for the immature neonatal immune systems and to mitigate infectious pathogens (Zhang et al., 2018). Interestingly, milk macronutrient components do not seem to have significantly increased with enhanced reproductive performance in sows as current concentrations of protein, fat and lactose in colostrum are similar to those of 30 years ago, i.e., approximately 16% protein, 3% lactose and 5% fat (Zhang et al., 2018).
The importance of colostrum and milk intake
Not surprisingly, therefore, the growth, development, immunity and biological functions of the GIT in the postnatal period are determined predominately by the amount of the colostrum and milk the piglet consumes, as well as its composition (Le Dividich & Herpin, 2005; Devillers et al., 2011; Figure 2). Also, the importance of initial postnatal microbial colonization of the GIT to its subsequent structure and function must also be recognized (e.g., Chen et al., 2018; Wang et al., 2019). Collectively, it is of critical importance that piglets consume colostrum as quickly as possible after birth. The ability of the epithelium to take up macromolecules, including immunoglobulins, and to then transport these molecules into the circulation concludes within the first 48 hours after parturition (Sangild, 2003). Failure by the piglet to consume and absorb sufficient quantities of immunoglobulins impacts negatively not only on viability, vitality and pre-weaning survival, but also on future growth and disease.
Colostrum also supplies energy in the form of macronutrients (as lactose, fat and proteins), and during the first days of life, it’s essential that the piglet consumes sufficient energy intake because the piglets’ energy body reserves, especially fat, are low at birth (Theil et al., 2014). Low energy intake coupled with an inability to thermoregulate can cause morbidity and death (Pluske et al., 1995; Theil et al., 2014). Colostrum intake consequently plays a major role in providing energy for thermoregulation.
Figure 2. Relationships (a) between immunoglobulin G (IgG) concentration in piglet plasma 24 h after the onset of farrowing (T24) and estimated colostrum intake, and (b) between total amount of IgG in piglet plasma at T24 and estimated IgG intake. Black circles represent piglets that were still alive at weaning, and white squares represent piglets that died between T24 and weaning (after Devillers et al., 2011).
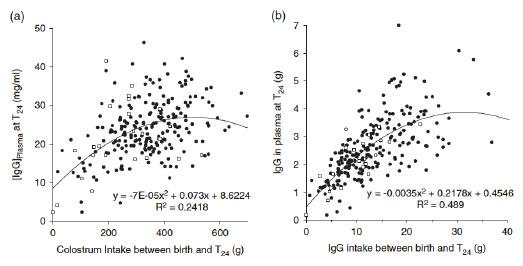
The intake of colostrum, and subsequently milk, causes rapid organ and body growth. For example, and in a neonatal piglet weighing on average 1.45 kg at birth, small intestinal and pancreas weights contribute approximately 3.1% and 0.14%, respectively, of the total body weight (Table 1). However, and within the first four weeks after birth the weight of the piglet increases more than five-fold, with the GIT organs growing faster than many other organs (Zabielski et al., 2008). Widdowson and Crabb (1976) showed the magnitude of changes to body weight, GIT weight, size and DNA content, that occur in newborn piglets during the first day of postnatal life, compared to piglets only offered water. Zabielski et al. (2008) described these changes being associated with increased local blood flow to the GIT with a reduction in basal vascular resistance, accumulation of colostral proteins in enterocytes, and changes in epithelial cell turnover, specifically increased mitosis and inhibition of apoptosis. Numerous subsequent studies have shown that the stomach, small and large intestine, liver and pancreas grow disproportionately faster than the rest of the body (positive allometric growth) during the early postnatal period. For example, the stomach gains 26-28% in weight during the first day compared with a 7-8% increase in body weight during the same period (summarized in Cranwell, 1995). There is also a profound functional maturation of the stomach during the early postnatal period (Cranwell, 1995).
The major driver of piglet growth in established lactation is milk intake (Pluske et al., 1995). Estimates of milk consumption by piglets vary considerably, but in general, each piglet consumes from ~ 40-60 grams of milk at each suckle (Pluske and Williams, 1996), and there is evidence that piglets sucking from modern-day first-litter sows will drink, on average, between ~ 45 grams of milk per day (Hojgaard et al., unpublished data). However, milk production of sows differs between weeks of lactation and between herds and depends on many factors including the proportion of unsuccessful sucklings, stage of lactation, parity, litter size, voluntary food intake during lactation, body composition of the sow at farrowing, genotype, and environmental conditions and health.
Table 1. The contribution of the selected organs to the total body weight (BW) in Landrace x Pietrain crossbred piglets (from Zabielski et al., 2008).

Sow milk dry matter is converted to piglet live-weight gain at a ratio of 0.75-0.80:1, or approximately 4 g of milk per gram of liveweight gain (summarised by Pluske et al., 1995). This does not seem to have changed significantly over the last ~ 40 years (Hojgaard et al., unpublished data). In energetic terms, Hodge (1974) calculated that, in artificially-reared pigs fed cows' whole-milk powder (reconstituted to 20% dry matter (DM)) between 10 and 30 days of age, 50 to 57% of the metabolizable energy (ME) intake was recovered in the empty body dependent upon feeding level, with more energy being retained at ad libitum intake. In sucking pigs slaughtered at 21 days of age, Noblet and Etienne (1987) found that between 52 and 57% of ME intake and 85% of the nitrogen supplied in sows' milk were recovered in the empty body. Furthermore, they determined that the daily rates of deposition of protein and fat were linearly related to daily empty bodyweight gain during suckling, with the deposition of 1 g of protein or fat being associated with 5.2 or 1.2 g of weight gain, respectively. An interesting feature of this work was the relatively low value obtained for the respiratory quotient (0.84). These authors commented that this value was most probably related to the high percentage of fat contained in sow's milk and to the large proportion of milk fat catabolized for energy purposes (only 54% of fat ingested was retained in the empty body at weaning; Table 2).
Nevertheless, many experiments conducted with ad libitum-fed, artificially-reared piglets weaned shortly after birth show that, compared to sucking piglets, offering milk liquid diets increases growth rate (summarised in Pluske et al., 1995). Presently, the growth potential of pre-weaned piglets only be fully realised when they are offered a liquid diet, because the young pig is physically unable to achieve as high a dry matter intake for dry food as when offered a liquid diet.
There are two major reasons why piglets sucking the sow are restricted in their growth rate. First, and unsurprisingly, sow milk production limits the growth of the pig. Harrell et al. (1993) calculated that milk production becomes limiting to the sucking piglet at around 8-10 days of age and that the difference between need and supply progressively increases as lactation proceeds (Figure 3). These authors estimated that, at day 21 of lactation, the sow needs to produce in excess of 18 kg per day of milk in order to supply piglets with enough energy to grow at rates comparable to artificially-reared piglets of the same age. Modern-day sows still suffer from an inability to produce enough milk and are expected to suckle more piglets also, placing additional demands on their metabolism and subsequent rebreeding performance.
Table 2. Energy, nitrogen and fat balance of suckling piglets between birth and weaning at 22 days of age (n = 20; results correspond to a litter and are expressed per piglet per day) (from Noblet and Etienne, 1987).
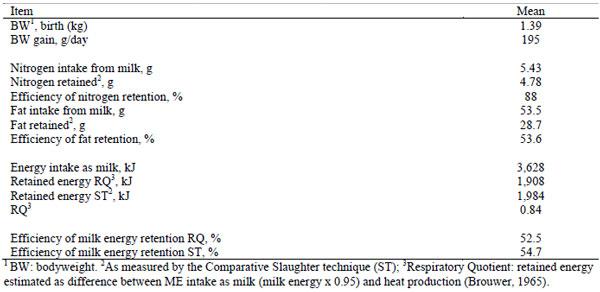
Second, the potential for lean tissue growth is restricted by the composition of sows’ milk. The newborn piglet is small in body size and has a large surface area to body weight ratio relative to other farm animals and is born with small amounts of body lipid [1-2% of total body weight (Mellor & Cockburn, 1986)]. Hence, liver glycogen stores and protein in its skeletal muscle represent the main energy stores to maintain body temperature. Sows’ milk is well designed for the survival of piglets because it is high in fat and it is delivered at frequent intervals (once each hour) by the sow. Sows’ milk is also low in protein and, because of this relatively low protein to energy ratio (9.2 to 10.4 g MJ-1 GE), it encourages the piglet to deposit body fat. A higher dietary protein intake reduces body fat deposition (Williams, 1976; Figure 4). Sows’ milk, therefore, is designed primarily to promote the deposition of fat in the piglet rather than lean tissue, and this seems to be the case for at least the first three weeks of life (Pluske et al., 1995). This phenomenon does not seem to have changed over the last 45 years (Hojgaard et al., unpublished data), and whilst early fat deposition aids the survival of piglets reared outdoors or those reared in suboptimal indoor conditions, it is doubtful whether piglets need to deposit fat so early in life when reared in modern commercial facilities where the physical and microbiological environment are more closely controlled (Pluske et al., 1995).
Figure 3. Voluntary food intake (MJ ME pig-1 day-1) of pigs either suckled by the sow (•--------•) or fed milk replacer (o--------o) following weaning at 2-3 days of age (redrawn from Harrell et al., 1993).
Be this as it may, the sow-suckled pigs (Figure 4) contained twice as much body fat as the leanest of the pigs which were artificially reared (0.46 v. 0.92 kg). This difference of 0.5 kg of body fat at 6.4 kg live weight might be quantitatively very important at weaning because, relative to the size of the piglet, it represents a large supply of energy which might help it adjust to the post-weaning milieu that occurs. Extra fat laid down during suckling becomes progressively less important as the pig grows because it is likely to have little effect other than to add to the total fat stores in the body. An extra 0.5 kg of fat, therefore, makes a small contribution to the fat stores of pigs at conventional slaughter weights of 80 to 120 kg (Pluske et al., 1995).
Figure 4. The effect of dietary protein on the body fat of piglets reared artificially on either 1.3 (•--------•) or 2.1 (o--------o) MJ of gross energy per kg live weight0.75 per day from 1.8 to 6.4 kg live weight. The amount of body fat contained in sow-suckled piglets killed at 6.4 kg live weight is represented by (< ) and corresponds to a protein content in sow's milk of ≈ 200 g kg-1 (redrawn from Williams, 1976).
Conclusions
Organ maturation in the piglet occurs rapidly in the perinatal period in association with the transition from placental to enteral nutrition, with the enteral intake of nutrients from colostrum and milk in newborn piglets causing profound and significant structural and functional changes in the GIT. The importance of colostrum composition and colostrum intake by the piglet cannot be understated given its critical roles in the subsequent growth and maturation of the GIT. Early postnatal growth and development of the GIT is coincidental with changes in the development of the intestinal immune system, being necessary for subsequent growth, performance and survival.
Colostrum and milk perform, therefore, the following major functions: (i) provide the young pig with a source of nourishment ideally adapted to its digestive and metabolic requirements; (ii) provide the young pig with protection against microbes via the intake of immunoglobulins contained in both colostrum and milk; (iii) suppress inflammatory reactions in the GIT of the piglet; (iv) supplement the digestive enzymes of the young pig; (v) stimulate cell division and differentiation in the GIT; (vi) exercise a degree of control over metabolism; (vii) modulate the endocrine system; and (viii) contain biologically-active compounds that have the potential to influence the behaviour of the young pig. Such a suite of functions ensures piglets are provided with good sources of nutrition. However, given that the composition of colostrum and milk has changed little over time, coupled to the characteristics of the modern-day, hyperprolific sows giving birth and rearing significantly more piglets than in the past, then research into these fields is required to continue.
Published in the proceedings of the International Pig Veterinary Society Congress – IPVS2020. For information on the event, past and future editions, check out https://ipvs2022.com/en.