We sincerelly thank the unconditional collaboration of the authors, and the kind disposition of the Mexican Association of Animal Nutrition (AMENA), and the Latin American College of Animal Nutrition (CLANA). Because of their support, Engormix.com brings closer the result of years of international research to the service of the animal producer.
E. J. Clowes1, K. T. Soltwedel2, T. S. Stahly3, F. X. Aherne4, and J. E. Pettigrew5.
1Alberta Agriculture, Food and Rural Development, Edmonton; 2University of Illinois, Urbana; 3Iowa State University, Ames; 4 Emeritus, University of Alberta, Edmonton
Introduction
Inadequate nutrient intake by the sow during lactation impairs subsequent fertility. Evidence for this can be found in both the results of controlled experiments (reviewed by Prunier and Quesnel, 2000) and in practical experience. This symposium deals with the biological mechanisms that connect nutrient intake to fertility, and this paper focuses on those mechanisms at the whole-animal level. We also give some attention to the influence of body nutrient stores at farrowing on the sow’s response to inadequate nutrient intake.
Inadequate nutrient intake occurs when the sow fails to consume enough nutrients to meet the enormous needs for milk production. Sow unit managers often restrict feed to lactating sows, especially during the early stages of lactation. However, the bigger concern is that sows fail to consume enough feed even when they have ad libitum access to it. We believe that the sow’s voluntary feed intake is not fixed, but that it is subject to management. Proper management of the animal and its environment, by controlling the effective thermal environment, feeding management, and diet formulation, can increase voluntary feed intake in lactating sows in many cases (Pettigrew and Esnaola, 2000). We also believe that pig producers around the world have increased the voluntary feed intake of their lactating sows substantially during the past decade, largely through attention to these management factors. We suspect that this increase in feed intake has contributed in some measure to the recent widespread improvement in reproductive efficiency in the USA (National Agricultural Statistics Service, 2003). The logical conclusion to this is that the problem of inadequate nutrient intake, and the associated mobilization of body tissues, is less severe than it was a decade ago.
The striking exception to this conclusion lies in hot climates. It is impossible to manage the environment to eliminate heat stress in many Latin American climates. In those situations, it is imperative that managers of lactating sows employ all management techniques at their disposal to enhance feed intake, but inadequate intake and excessive mobilization of body tissues may still occur. That fact focuses attention on the subject of this symposium.
Body Condition vs. Metabolic State
The detrimental effects of inadequate nutrient intake on fertility may be mediated through effects on at least the following:
1. Metabolic state, broadly defined;
2. Rate of mobilization of body protein and/or fat;
3. Amount of body protein and/or fat in the sow at weaning/rebreeding.
The concept of metabolic state as used here is quite broad. It encompasses the concentrations of several metabolites and metabolic hormones, a subject reviewed in depth in this symposium by Dr. Quesnel, and tissue sensitivity to these hormones. Tissue sensitivity to these hormones is an important component of metabolic state, which can alter the signal transmitted by the circulating hormones, and affects the hypothalamo-pituitary-ovarian axis either directly or indirectly. Metabolic state also encompasses the concept of total oxidizable substrates (Wade and Schneider, 1992; Wade et al., 1996), and measures of the metabolic conditions of body tissues (especially protein and fat) themselves, such as the size of the tissue reserve and the rate of tissue mobilization (synthesis/degradation). The fact that many factors are involved in the regulation of reproductive function is not a surprise, as most complex functions have multiple regulators. So it would be unusual if only one component of metabolic state regulated such an important and complex physiological function as reproduction.
It is practically important to know whether metabolic state (1), and its associated effects on rate of body tissue mobilization (2), or the amount of reserves remaining at weaning (3) is more important for subsequent fertility. If a sow’s subsequent fertility depends largely on a high level of body reserves at weaning, it would be practical to feed the sow liberally during pregnancy to ensure development of adequate body tissues to compensate for tissue mobilization during lactation. However, excessive feeding during pregnancy reduces feed intake during lactation, presumably because of excessive fatness (Xue et al., 1997; Revell et al., 1998; Sinclair et al., 2001), and this results in an increased rate of tissue mobilization. To help determine the appropriate feeding strategy during pregnancy it is necessary to discover whether metabolic state, and its associated effects on the rate of tissue mobilization, or the amount of body protein and/or fat remaining at weaning/re-breeding is more important. As described below, it is likely that all these factors are important.
Frisch (1984) proposed that a threshold amount of body fat is necessary for fertility. But, no consistent threshold of body fat has been associated with post-weaning reproductive problems. This, together with the fact that these observations are purely correlative, reduces the confidence in the hypothesis that the size of the body fat reserve is a primary controller of fertility in the pig. However, we do present evidence that the size of body protein stores at farrowing impacts on the sow’s subsequent reproductive performance.
Experimental treatments imposed only during lactation cannot distinguish between metabolic state and its associated effects on the rates of tissue mobilization and the amount of tissue remaining at weaning, because the two are perfectly confounded. However, direct experimental evidence indicates that the metabolic state (or rate of tissue mobilization) of the sow during lactation may be more important than the size of the sow’s body stores of protein and/or fat in influencing fertility. Two examples of such evidence are described below.
First, Mullan and Williams (1989) fed first-parity sows at three levels of intake during pregnancy, then at two levels during lactation. Two of the treatments (high intake during pregnancy and a low intake during lactation vs. medium intake in gestation and a high intake during lactation) had similar body weights (~125 kg) and composition (~23mm P2 backfat depth) at weaning, but very different subsequent reproductive performance. The clear advantage lay with the treatment that had a high feed intake during lactation. These sows likely exhibited a lower rate of tissue mobilization, and were in a less severe catabolic state during lactation.
Second, Zak et al. (1997a,b) fed sows to appetite during the first 21 d of lactation and then restricted intake to 50% of the to appetite level during the last week, or restricted feed intake during the first 21 d of lactation and fed to appetite during the last week. Sows on both treatments lost a similar amount of body weight and backfat depth during lactation. However, follicular fluid taken at proestrus (~3 d post-weaning) from sows restrict-fed at the end of lactation supported poorer generic oocyte maturation and the oocytes from these sows matured to a lesser degree in generic follicular fluid (Table 1). Sows on this treatment also showed lower mean LH levels at the end of lactation and a lower embryo survival rate (Table 1). These sows lost more body weight in the last week of lactation and perhaps had a higher protein mobilization rate compared to sows on the other treatment. This indicates that a more catabolic state during the last week of lactation enhances both the environment (follicular fluid) surrounding the oocyte as well as the potential of the oocyte to mature, resulting in increased embryo survival. However, any period of nutritional insult in lactation is likely to impede follicular fluid quality and oocyte maturation, because an antral follicle may be recruited into the pre-ovulatory pool in the span of 19 to 21 days (Morbeck et al., 1992). But if the nutritional/metabolic insult is too severe, improved nutrition just prior to weaning will only allow partial recovery of the sow’s reproductive status (Table 1).
Body Tissue Mobilization
A reduction in subsequent reproductive performance (Aherne and Kirkwood, 1985; Prunier et al., 1993) occurred in sows after loss of 10 to 15% of their body weight during lactation; a loss of 18 to 26 kg of body weight in a 180 kg sow. This body weight loss is composed of both protein and fat containing tissue. A review of the literature available several years ago (King, 1987) indicated that excessive protein mobilization during lactation is more detrimental to subsequent fertility than is excessive fat mobilization. More recently, a regression analysis of data from 16 published experiments indicates that the fractional loss of body protein during lactation accounts for almost half the variation in the sow’s post-weaning reproductive performance (wean-to-estrus interval; Figure 1a). In contrast, less than a quarter of the variation in the same measure was accounted for by loss of body fat (Figure 1b). Post-weaning reproductive performance also appears to decline when sows are fed less than 500 to 600 g CP/d in lactation (Figure 1c).
Further analysis of this data set suggests that loss of more than 16% of a sow’s body protein mass is associated with a progressive decline in subsequent reproductive performance, as indicated by an extended wean-to-estrus interval (Figure 2). Mobilization of about 15% of an animal’s protein mass has also previously been implicated in reduced lactational performance in the dairy cow (Botts et al., 1979) and rat (Pine et al., 1994abc). Similarly, marked reductions in many indices of ovarian function at weaning (Clowes et al., 2003a) were associated with loss of 12% or more of the sow’s protein mass. A slight impairment of the reproductive axis appeared to occur in sows that sustained about a 9% loss in body protein (Table 2). Furthermore, the increase in subsequent litter size achieved by breeding first-parity sows at their second rather than first estrus after weaning was attributed to changes in the sow’s protein metabolism (Clowes et al., 1994). These observations confirm the detrimental effects to fertility of the metabolic conditions associated with rapid protein mobilization.
Physiological mechanisms of effects of protein loss on reproduction. Subsequent litter size is a function of ovulation rate and embryo survival. Amino acid deficiency during lactation has equivocal effects on ovulation rate in the sow, and causes either no effect (King and Williams 1984ab; Yang et al., 1989; Zak et al., 1998) or a reduction (Zak et al., 1997a; van den Brand et al., 2000; Mejia-Guadarrama et al., 2002) in ovulation rate. Thus, rapid maternal protein loss likely reduces the sow’s subsequent reproductive performance by reducing embryo survival. These effects of body protein loss on reproduction are presumed to impact the hypothalamic-pituitary axis and/or the ovary, causing poor follicle and oocyte development, and ultimately reducing embryo survival and subsequent litter size.
Central inhibition of the reproductive axis is indicated by a reduction in LH pulsatility, probably due to a reduction in the rate of GnRH release from the hypothalamus. Lower LH pulsatility in lactation (King and Martin, 1989; Jones and Stahly, 1999b, Yang et al., 2000b) and after weaning (King and Martin, 1989) was observed in first-parity sows fed similar energy levels but low protein (~ 400 g/d) and total lysine (16 g/d) intakes in lactation, compared to adequately fed controls. These restricted sows likely mobilized a larger proportion of their protein mass, and had higher rates of muscle protein mobilization throughout lactation.
The quality of the follicle, and the oocyte within that follicle, are important for normal oocyte maturation and early embryonic development (Ding and Foxcroft, 1994). The oocyte’s ability to be fertilized and develop into an embryo is influenced by the concentration of a complex mixture of serum proteins and factors such as IGF-1 and EGF, and steroid hormones such as estradiol (Gougeon, 1996; Driancourt and Thuel, 1998) in follicular fluid. These factors may be altered by nutrition, and this provides a direct connection between nutritional inadequacy and inhibition of the reproductive axis at the ovarian level.
Three recent studies have implicated excessive maternal protein loss in lactation and/or a higher protein mobilization rate at the end of lactation with reduced ovarian function post-weaning (Zak et al., 1997b; Yang et al., 2000a, Clowes et al., 2003a). The first experiment (Zak et al., 1997b) was described in an earlier section. It showed detrimental effects of severe feed restriction during the last week of lactation on the quality of follicular fluid and the oocyte.
In the second experiment, follicular fluid taken at pro-estrus from first-parity sows fed 16 compared to 36 and 56 g/d of total lysine was less able to support oocyte maturation (Yang et al., 2000a). These sows lost the most body protein during lactation and had a higher fractional myofibrillar protein breakdown rate (5.6 vs. < 4.2%) at the end of the 15-day lactation. Sows on this experiment were fed isoenergetic diets (~ 46 MJ ME/d) during lactation, lost only a small amount of body fat (-1.4 to -2.2mm of backfat), but as dietary lysine intake decreased, sows lost progressively more live weight (-15, -19, and –22 kg).
In the third experiment, few differences were observed between sows that lost 9% and those that lost 7% of their protein mass, in a variety of ovarian variables at weaning and the ability of follicular fluid to advance in vitro maturation of oocytes (Clowes et al., 2003a; Table 2). However, marked reductions in many indices of ovarian function were observed in sows that had lost more (~15%) body protein and exhibited higher rates of muscle protein mobilization (Clowes et al., 2000). A poor uterine environment may also be implicated in the reduced embryonic survival in sows with excessive protein loss, especially when superimposed upon embryos developing from poorly matured oocytes.
The Importance of Body Protein Reserves at Farrowing
While there is ample reason to believe that the sow’s metabolic state during lactation, is a critical link between nutrition and fertility, there is also convincing evidence that the amount of body stores at farrowing is important. Two examples of that evidence are described below.
First, the factorial experiment of Mullan and Williams (1989) evaluating the effects of feeding level during both pregnancy and lactation found the poorest reproductive performance in sows given the very low feed allowances during both stages.
Second, a more recent experiment by Clowes et al. (2003b; Table 3) repeated this observation, but with three important differences. First, the sows were managed to have at farrowing similar relative fatness but different levels of body weight (193 vs. 165 kg) and body protein (30.0 vs. 24.3 kg). Second, the measurements were extended to include specific measures of ovarian development. Third, measures of gene expression confirmed that sows that were small at farrowing and restricted during lactation had the greatest up-regulation of gene expression in the main proteolytic pathway in muscle, and the lowest capacity for protein synthesis in muscle (Clowes et al., 2002).
Pettigrew et al. (1992) proposed a mathematical model of the metabolism of lactating sows that incorporated two key concepts related to the amount of body protein reserves in the sow. There was (and remains) inadequate quantitative data to support parameterization of these aspects of the model with confidence, but the concepts remain useful.
The first of these concepts is that both protein synthesis and degradation can be expressed per unit of protein mass. As undernutrition depletes the amount of body protein during lactation, the body protein mass shrinks. In this case, a constant fractional rate of net mobilization would therefore result in a reduced rate of total amino acid release. Maintenance of a constant supply of amino acids then requires a stronger catabolic stimulus to achieve a greater fractional rate of mobilization. At some point, the protein reserve may become so depleted and/or the rate of mobilization may reach a physiologic maximum that the total supply of amino acids:
- will not meet the needs of the lactating mammary gland to maintain milk production
- will not provide sufficient amino acid precursors for biosynthesis of proteins associated with ovarian function
As a result, milk production will fall and/or milk protein composition will decline, and embryo survival will also decline.
A corresponding relationship holds with regard to initial body protein mass. Sows with smaller body protein stores would require a stronger catabolic stimulus to provide the same supply of amino acids to the mammary glands. This more catabolic state may have severely detrimental effects on the reproductive system. Recent data on gene expression in muscle (Clowes, 2001; Clowes et al., 2002) appear to confirm the predicted impact of both initial protein mass and degree of protein mobilization on the fractional protein mobilization rate.
The second concept is that the animal protects itself from excessive depletion of its body stores. The physiological mechanisms for sensing the degree of depletion (or of remaining tissue) and orchestrating the protective response are unknown, as are the quantitative relationships between rate of mobilization and the size of the remaining tissue reserve. However, the result is the protection of the sow’s body at the expense of both milk production and the metabolic conditions necessary for subsequent fertility.
Both of these concepts suggest that depletion of body reserves would progressively reduce the supply of nutrients supplied from further tissue mobilization, and there is strong empirical support for this relationship. Several studies (Mullan and Williams, 1989; Jones and Stahly, 1999a; Kusina et al., 1999; Clowes et al., 2003a) have shown that excessive tissue depletion progressively reduces milk yield as lactation continues, and that both the amount of stores at farrowing and the nutrient supply during lactation are important in this regard. Furthermore, a loss of body protein, beyond a 9 to 12% loss, results in a continued decline in milk yield, and may even result in a reduction in milk protein composition (Clowes et al., 2003 a,b). But, feeding first-parity sows to above their ad libitum intake appeared to have no beneficial effect on milk production (Pluske et al., 1998) or reproductive performance (Zak et al., 1998) in some situations.
The implication of the importance of body stores at farrowing is that sows should be fed generously enough during pregnancy to develop a protective level of body stores. Excessive fatness reduces feed intake during lactation, so the aim should be to develop a larger body protein reserve but a moderate body fat reserve (Pettigrew and Yang, 1997). Producing heavier gilts at farrowing, by breeding at a heavier weight and/or feeding a higher energy/protein intake during gestation, could be a useful management tool if poor appetite and subsequent reproductive performance are a problem in a commercial herd. However, the economic impact of these findings and the effects on overall animal productivity need to be further studied.
Management to Maximize Feed Intake
A general theme of this discussion is that adequate nutrient intake of the lactating sow relative to her needs is important for achieving the desired metabolic state that will support subsequent fertility. As mentioned above, we believe that proper management is important in encouraging a high level of feed intake. The challenge of getting sows to eat enough during lactation is more acute when the sow is heat-stressed, but proper management can help.
We suggest the proper management factors to encourage a high level of feed intake fall into three categories (Pettigrew and Esnaola, 2000):
Minimizing heat stress.
The first requirement is for adequate and effective ventilation. Natural ventilation systems in hot climates should incorporate high roofs, with the roof extending far enough to provide shade to all animals. The buildings should be far enough apart to allow adequate air movement. Among fan-powered ventilation systems, tunnel ventilation is especially effective in high temperatures. Circulating fans within the building are also effective.
Evaporative cooling can be useful. Evaporative systems include the enormously effective dripping of water on the sow, wetting the roof, and pulling incoming air through water-soaked pads. Floors made of materials that conduct heat away from the sow contribute to cooling.
Feeding management.
It is important to feed lactating sows frequently; we suggest at least three times daily. Ensure feed is available during the coolest times if daytime temperatures are high. Use feeders that allow comfortable access to the feed. An adequate supply of water is essential, and that includes an adequate flow rate through nipple waterers. Wet/dry feeding systems, in which the sow can mix feed and water, appear to increase feed intake in both finishing pigs and lactating sows. Various methods of wet-feeding, providing feed mixed with water to form a gruel, are used effectively to encourage a high level of feed intake, especially in hot weather.
Diet formulation.
It is necessary to provide adequate dietary levels of all essential amino acids. It is also important to minimize the total protein content of the diet, to minimize the heat production associated with elimination of excess nitrogen. For these reasons, crystalline amino acids are especially useful during hot weather.
The heat increment of fat is low, so fat supplementation of lactation diets is especially important during hot weather. The heat increment of fiber is high, so fibrous diets should be avoided.
Excessive levels of minerals should be avoided, and providing an appropriate cation:anion ratio may be useful.
Summary
Excessive mobilization of body tissues by the lactating sow appears avoidable in many situations, but probably not in hot climates. The metabolic state of the sow and its associated rate of mobilization of body tissues appears more important for subsequent fertility than the amount of body stores in the sow at weaning/re-breeding. Rapid mobilization of body protein during lactation appears more detrimental than excessive mobilization of body fat. Sows can mobilize up to about 10% of their initial body protein mass during lactation without significant detriment to fertility, but more severe mobilization is damaging. The consequences of inadequate amino acid intake appear to include effects on the hypothalamic/pituitary axis and direct effects on the ovary that reduce the quality of the developing follicles. A large body protein reserve at farrowing appears to be protective. Management practices to encourage a high level of feed intake by lactating sows are offered.
Literature Cited
Aherne, F.X., and R.N. Kirkwood. 1985. Nutrition and sow prolificacy. J. Reprod. Fert. Suppl. 33:169-183.
Baidoo, S. K., F. X. Aherne, R. N. Kirkwood, and G. R. Foxcroft. 1992. Effect of feed intake during lactation and after weaning on sow reproductive performance. Can. J. Anim. Sci. 72:911-917.
Botts, R.L., R. W. Hemken, and L. S. Bull. 1979. Protein reserves in the lactating dairy cow. J. Dairy Sci. 62: 433-440.
Clowes, E. J., F.X. Aherne and G.R. Foxcroft. 1994. Effect of delayed breeding on the endocrinology and fecundity of sows. J. Anim. Sci. 72: 283-291.
Clowes, E. J., V. E. Baracos, and F. X. Aherne. 2000. Skeletal muscle mobilization in lactating sows with divergent lactational protein loss. J. Anim. Sci. 78 (Suppl. 1):174
(Abstr.)
Clowes, E.J. 2001. Lactational muscle protein mobilization and sow performance. PhD thesis from the University of Alberta
Clowes, E. J., F.X., Aherne, A.L. Schaefer, and V. E., Baracos. 2002. Evaluation of maternal muscle protein mobilization during lactation in first-litter sows of differing body size. J. Anim. Sci. 80 (Suppl. 1): 218 (Abstr. 867)
Clowes, E. J., F. X. Aherne, G. R. Foxcroft, and V. E Baracos. 2003a. Selective protein loss in lactating sows is associated with reduced litter growth and ovarian function. J. Anim. Sci. 81: 753-764
Clowes, E. J., F. X. Aherne, A. L. Schaefer, G. R. Foxcroft, and V. E Baracos. 2003b. Parturition body size and body protein loss during lactation influence performance during lactation and ovarian function at weaning in first-parity sows. J. Anim. Sci. 81(in press)
Ding, J., and G. R. Foxcroft. 1994.Conditioned media produced by follicle shells of different maturity affect maturation of pig oocytes. Biol. Reprod. 50:1377-1384.
Driancourt, M-A., and B. Thuel. 1998. Control of oocyte growth and maturation by follicular cells and molecules present in follicular fluid. A review. Reprod. Nutr. Dev. 38:345-362.
Frisch, R. E. 1984. Body fat, puberty and fertility. Biol. Rev. 59:161-188.
Gougeon, A. 1996. Regulation of ovarian follicular development in primates: facts and hypotheses. Endocrine Reviews 17:121-155.
Jones, D. B., and T. S. Stahly. 1999a. Impact of amino acid nutrition during lactation on body nutrient mobilization and milk nutrient output in primiparous sows. J. Anim. Sci. 77:1513-1522.
Jones, D. B., and T. S. Stahly. 1999b. Impact of amino acid nutrition during lactation on luteinizing hormone secretion and return to estrus in primiparous sows. J. Anim. Sci. 77:1523-1531.
King, R.H. 1987. Nutritional anoestrus in young sows. Pig News and Information, 8:15-22.
King, R. H., and A. C. Dunkin. 1986a. The effect of nutrition on the reproductive performance of first-litter sows. 3. The response to graded increases in food intake during lactation. Anim. Prod. 42:119-125.
King, R. H., and A.C. Dunkin. 1986b. The effect of nutrition on the reproductive performance of first-litter sows. 4. The relative effects of energy and protein intakes during lactation on the performance of sows and their piglets. Anim. Prod. 43:319-325.
King, R.H. and G. B. Martin. 1989. Relationship between protein intake during lactation, LH levels and oestrus activity in first-litter sows. Anim. Reprod. Sci. 19:283-292.
King, R. H., and I. H. Williams. 1984a. The effect of nutrition on the reproductive performance of first-litter sows. 1. Feeding level during lactation, and between weaning and mating. Anim. Prod. 38:241-247.
King, R. H., and I. H. Williams. 1984b. The effect of nutrition on the reproductive performance of first-litter sows. 2. Protein and energy intakes during lactation. Anim. Prod. 38:249-256.
Koketsu, Y, G. D. Dial, J. E. Pettigrew, and V. L. King. 1996. Feed intake pattern during lactation and subsequent reproductive performance of sows. J. Anim. Sci. 74:2875-2884.
Kusina, J., J. E. Pettigrew, A. F. Sower, M. E. White, B. A. Crooker, and M. R. Hathaway. 1999. Effect of protein intake during gestation and lactation on the lactational performance of primiparous sows. J. Anim. Sci. 77:931-941.
Mao J., L. J. Zak, J. R. Cosgrove, S. Shotak, G. R. Foxcroft. 1999. Reproductive, metabolic, and endocrine responses to feed restriction and GnRH treatment in primiparous, lactating sows. J. Anim Sci. 77:724-35.
Mejia-Guadarrama, C.A., A. Pasquier, J.Y. Dourmad, A. Prunier, and H. Quesnel. 2002. Protein (lysine) restriction in primiparous lactating sows: Effects on metabolic state, somatotropic axis, and reproductive performance after weaning. J. Anim. Sci. 80: 3286-3300.
Morbeck, D. E., K. L. Esbenshade, W. L. Flowers, and J. Britt. 1992. Kinetics of follicle growth in the prepubertal gilt. Biol. Reprod. 47:485-491.
Mullan, B. P., and I. H. Williams. 1989. The effect of body reserves at farrowing on the reproductive performance of first-litter sows. Anim. Prod. 48:449-457.
National Agricultural Statistics Service (NASS), Agricultural Statistics Board, United States Department of Agriculture. 2003. Quarterly Hogs and Pigs, Released June 27, 2003. http://jan.mannlib.cornell.edu/reports/nassr/livestock/php-bb/2003/hgpg0603.pdf. Accessed July 3, 2003.
Pettigrew, J.E. and M.A. Esnaola. 2000. Alimentacion y manejo de la cerda bajo condiciones de estres de calor. Proceedings, II Congreso Nacional de Porcicultura & Expo Porcina, pp 77-91.
Pettigrew, J.E., M. Gill, J. France and W.H. Close. 1992. A mathematical integration of energy and amino acid metabolism of lactating sows. J. Anim. Sci. 70:3742-3761.
Pettigrew, J.E., and H. Yang. 1997. Protein nutrition of gestating sows. J. Anim. Sci. 75:2723-2730.
Pine, A. P., N. S. Jessop, and J. D. Oldham. 1994a. Maternal protein reserves and their influence on lactational performance in rats. Br. J. Nutr. 71: 13-27.
Pine, A. P., N. S. Jessop, G. F. Allan, and J. D. Oldham. 1994b. Maternal protein reserves and their influence on lactational performance in rats. 2. Effects of dietary protein restriction during gestation and lactation on tissue protein metabolism and Na+, K+-ATPase (EC 3.6.1.3) activity. Br. J. Nutr. 72:181-197.
Pine, A. P., N. S. Jessop, G. F. Allan, and J. D. Oldham. 1994c. Maternal protein reserves and their influence on lactational performance in rats. 4. Tissue protein synthesis and turnover associated with mobilization of maternal protein. Br. J. Nutr. 72:831-844.
Pluske, J.R., I.H. Williams, L. Zak, E.J. Clowes, A.C. Cegielski, and F.X. Aherne. 1998. Feeding lactating primiparous sows to establish three divergent metabolic states. 3. Milk production and pig growth. J. Anim. Sci. 76:1165-1171.
Prunier, A., J.Y. Dourmad, and M. Etienne. 1993. Feeding level, metabolic parameters and reproductive performance of primiparous sows. Livest. Prod. Sci. 37:185-196.
Prunier, A. and H. Quesnel. 2000. Nutritional influences on the hormonal control of reproduction in female pigs. Livest. Prod. Sci. 63:1-16.
Revell, D. K., I. H. Williams, B. P. Mullan, J. L. Ranford, and R. J. Smits. 1998. Body composition at farrowing and nutrition during lactation affect the performance of primiparous sows: I. Voluntary feed intake, weight loss and plasma metabolites. J. Anim. Sci. 76:1729-1737
Sinclair, A. G., V. C. Bland, and S. A. Edwards. 2001. The influence of gestation feeding strategy on body composition of gilts at farrowing and response to dietary protein in a modified lactation. J. Anim. Sci. 79:2397-2405.
Tritton, S. M., R. H. King, R. G. Campbell, A. C. Edwards, and P. E. Hughes. 1996. The effects of dietary protein and energy levels of diets offered during lactation on lactational and subsequent reproductive performance. Anim. Sci. 62:573-579.
van den Brand, H., S. J. Dieleman, N. M. Soede, and B. Kemp. 2000. Dietary energy source at two feeding levels during lactation of primiparous sows: I. Effects on glucose, insulin, and luteinizing hormone and on follicle development, wean-to-estrus interval, and ovulation rate. J. Anim. Sci. 78:396-404.
Wade, G.N. and J.E. Schneider. 1992. Metabolic fuels and reproduction in female animals. Neuroscience and Behavioural Rev. 16:235-272.
Wade, G. N., J. E. Schneider, and H-Y Li. 1996. Control of fertility by metabolic cues. Am. J. Physiol. 270:E1-E19.
Whittemore, C. T. and H. Yang. 1989. Physical and chemical composition of the body of breeding sows with differing body subcutaneous fat depth at parturition, differing nutrition during lactation and differing litter size. Anim. Prod. 48:203-212.
Xue, J.L., Y. Koketsu, G.D. Dial, J.E. Pettigrew and A.F. Sower. 1997. Glucose tolerance, luteinizing hormone release, and reproductive performance of first litter sows fed two levels of energy during gestation. J. Anim. Sci. 75:1845-1852.
Yang, H., E.R. Eastham, P. Philips, and C.T. Whittemore. 1989. Reproductive performance, body weight and body condition of breeding sows with differing body fatness at parturition, differing nutrition during lactation and differing litter size. Anim. Prod. 48:181-201.
Yang, H., G. R. Foxcroft, J. E. Pettigrew, L. J. Johnson, G. C. Shurson, A. N. Costa, and L. J. Zak. 2000a. Impact of dietary lysine intake during lactation on follicular development and oocyte maturation after weaning in primiparous sows. J. Anim. Sci. 78:993-1000.
Yang, H., J.E. Pettigrew, L. J. Johnson, G. C. Shurson, J. E. Wheaton, M. E. White, Y. Koketsu, S. F. Sower, and J. A. Rathmacher. 2000b. Effects of dietary lysine intake during lactation on blood metabolites, hormones, and reproductive performance in primiparous sows. J. Anim. Sci. 78:1001-1009.
Zak, L. J., J. R. Cosgrove, F. X. Aherne, and G. R. Foxcroft. 1997a. Pattern of feed intake and associated metabolic and endocrine changes differentially affect postweaning fertility in primiparous lactating sows. J. Anim. Sci. 75:208-216.
Zak, L. J., X. Xu, R. T. Hardin, and G. R. Foxcroft. 1997b. Impact of different patterns of feed intake during lactation in the primiparous sow on follicular development and oocyte maturation. J. Reprod. Fert. 110:99-106.
Zak, L. J., I. H. Williams, G. R. Foxcroft, J. R. Pluske, A. C. Cegielski, E. J. Clowes, and F. X. Aherne. 1998. Feeding lactating primiparous sows to establish three divergent metabolic states: I Associated endocrine changes and postweaning reproductive performance. J. Anim. Sci. 76:1145-1153.
Table 1. Effects of pattern of feeding over a 28-day lactation on post-weaning fertility in first-parity sows bred at first post-weaning estrus (from Zak et al., 1997ab)
Within a row, means without a common superscript letter differ by xy P < 0.01, xz P < 0.054.
Change in weight and backfat depth between d 21 and 28, and the # of embryos at d 28 were not statistically analysed in these papers
Table 2. Lactational and reproductive performance of first-parity sows that lost a high, moderate, or low amount of body protein in lactation (From Clowes et al., 2003a)
a Body protein and fat mass predicted from the equations of Whittemore and Yang (1989).
b Average follicular fluid volume from the largest 16 follicles (largest eight from each ovary) measured at weaning..
c Follicular fluid variables were measured on the largest eight follicular fluid volumes collected at weaning.
d Tissues were collected 2 to 4 h after weaning, on d 23 of lactation, at the time of slaughter.
xy Within a row, means without a common superscript letter differ by the P-value in that row.
Table 3. Reproductive performance, and muscle parameters at weaning, in first-parity sows with either a standard (165 kg) or high (193 kg) body mass at farrowing, that lost a high amount of protein during lactation (From Clowes et al., 2002 and 2003b)
a Body protein and fat mass calculated from the equations of Whittemore and Yang (1989)
xy Within a row, means without a common superscript letter differ by P < 0.05
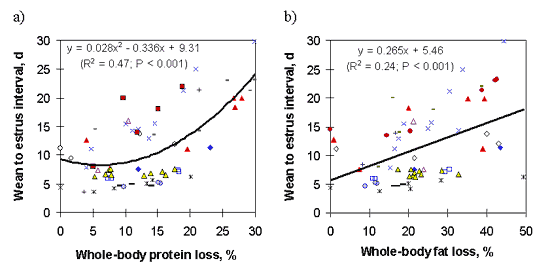
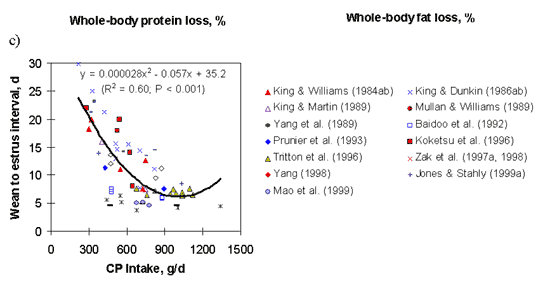
Figure 1. Regression analysis of subsequent wean-to-estrus interval against the sow’s estimated whole-body a) protein and b) fat loss in lactation, and c) lactational crude protein intake. Body protein and fat mass are presented as a percentage of the parturition tissue mass, and were estimated from the equations of Whittemore and Yang (1989).
Adapted from Clowes, (2001)
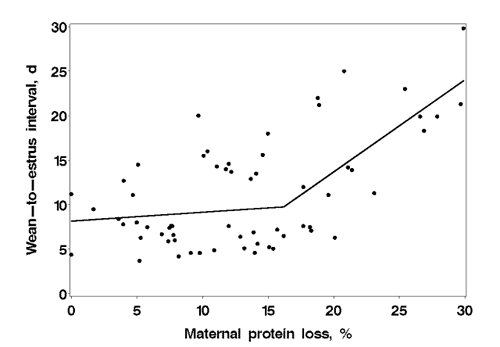
Figure 2. Break–point analysis of maternal protein loss versus wean-to-estrus interval (WEI) on retrospective data from 16 published experiments using two-phase regression. Adapted from Clowes et al. (2003a).