INTRODUCTION
Iowa leads the nation in corn and ethanol production. For corn-based ethanol plants, a primary co-product of the process is distillers dried grains with solubles (DDGS). DDGS have been reported to contain high levels of digestible energy and metabolizable energy, digestible amino acids, and available phosphorus (Shurson et al., 2003; Honeyman et al., 2007). Generally, DDGS have been found to contain 2 to 3.5 times more amino acids, fat, and minerals than corn (Honeyman et al., 2007). Animal nutritionists have suggested including up to 20% DDGS in nursery, grow-finish, and lactating sow diets and up to 40% in gestating sows and boars (Honeyman et al., 2007). However, the decision to feed DDGS is generally based on economics. At the current DDGS and corn prices the inclusion of DDGS in swine diets has provided a cost savings over traditional non-DDGS diets.
It has been hypothesized that sulfur levels in DDGS could result in increased hydrogen sulfide (H2S) emissions from stored swine manure when pigs were fed rations containing DDGS. However, comparative data from full-scale swine production systems is needed to confirm any impacts on air emissions from feeding DDGS. The increased usage of DDGS at swine facilities has led several researchers to examine the effect of DDGS on emissions, odors, and manure composition, but these studies have been at lab or at noncommercial scales and the data from these studies have not produced consistent data.
Spiehs et al. (2000) performed a 10-week trial on 20 barrows receiving either a DDGS (at a 20% inclusion rate) or non-DDGS ration. The pigs were housed, based on diet, in two fully-slatted pens within the grow-finish room of a swine research facility. The non-DDGS diet was a typical corn-soybean meal; total phosphorus and total lysine were held constant in both diets within each phase of feeding. The study was conducted to evaluate differences in odor, H2S, and Ammonia (NH3)from stored manure as a result of the pig's diet. The stored manure that was evaluated for emissions was maintained in a container to simulate deep pit storage. Air samples were collected from the headspace of storage containers. Over the 10-week period, this study reported that DDGS (at a 20% inclusion level) did not affect odor, H2S, or NH3 emissions in the stored manure.
Conversely, Powers et al. (2008 & 2006) completed a study in 2006 that included 48 barrows in 8 chambers at Iowa State University. In the study, the animals received increasing amounts of DDGS in their ration (from 0 – 30%) as they progressed through their feeding phases; corn based control diets were also included. The diets were formulated to contain similar amounts of lysine and energy. Manure collection pans were placed under the animal pens and were partially cleaned twice weekly to remove manure and prevent overflow. Air samples were collected from within the animal chambers. The reported results indicated that the NH3 and H2S emission rates from the chamber were higher as a result of the DDGS ration, but methane emissions were reduced.
The results of these studies cannot be directly compared because of differences in rations, animal housing, manure storage, and analytical methods. However, in general, the studies provide conflicting results. Besides differences in the experimental design of the two studies, the conflicting results may also be affected by scaling issues. The objective of this paper was to quantify the impact on gaseous emissions of feeding DDGS to finishing pigs in two commercial deep-pit swine facilities. To meet this objective NH3, greenhouse gases (GHG) (carbon dioxide – CO2, nitrous oxide – N2O, and methane – CH4), and H2S concentrations were measured and emission data were collected using a mobile air emissions monitoring unit (MAEMU).
METHODS AND MATERIALS
Site and Instrumentation Description
Two 12.5 x 57 m (50 x 190 ft) co-located wean-to-finish deep pit swine barns, designated as barn 1 and barn 2, located in central Iowa were monitored in this study. Pigs started in the barns at 5.5 kg (12 lbs) and were marketed at 118 kg (260 lbs) with two turns completed a year. The barns have a rated capacity of 1,300 marketed head. Both barns were doubled stocked initially, meaning during the wean to grow phase both barns held 2600 pigs, roughly. When the pigs weighed 27 kg (60 lbs), approximately half the pigs were moved off site to another facility. Each barn had four 0.6 m (24 in.) pit fans, two 0.6 m (24 in.) endwall fans and sidewall curtains on both sides to provide natural ventilation when needed. The barns were equipped with three space heaters 66 kW (225,000 BTU/h) each, 20 brooder heaters 5 kW (17,000 BTU/h) each and 20 bi-flow ceiling inlets. Both barns were managed identically with the exception of feed rations. Barn 1 received a traditional corn based diet (non-DDGS) while Barn 2 received a DDGS (22%) ration. The producer provided weekly pig performance data, including mortality and average body weight for the duration of this project.
A MAEMU was used to continuously collect emissions data from two deep pit wean-to-finish swine barns. The instruments and data acquisition system were housed in the MAEMU. A detailed description of the MAEMU and operation can be found in Moody et al. (2008). To date this study has monitored NH3, CO2, N2O, CH4, and H2S aerial emissions for three months. A photoacoustic multi-gas analyzer (INNOVA Model 1412, INNOVA AirTech Instruments A/S, Ballerup Denmark) was used to measure NH3, CO2, N2O, and CH4 concentrations. H2S concentrations were measured using an Ultraviolet Fluorescence H2S analyzer (Model 101E, Teledyne API, San Diego, CA). Instruments were challenged weekly with calibration gases and recalibrated as needed. All calibration gases were certified grade with ± 2% accuracy
Air samples were collected in 30-s cycles for four cycle periods (120 s) at each location. The fourth reading from each sampling cycle was used as the measured pollutant concentration. Use of the fourth reading was due to the fact that the Innova and API had T98 and T95 response time of 120 s and 100 s, respectively. Air samples were drawn from three composite locations (north pit fans, south pit fans, and endwall fans) in each barn and an outside location to provide ambient background data (Figure 1). Each composite sampling location was chosen to match the fan stages used at this facility. Pit fan sampling points were located below the slats directly below each fan. Endwall sample ports were placed approximately 1.0 m (3.28 ft) in front of each endwall fan. Sample locations and placement of sampling ports were chosen to ensure representativeness of the air leaving the barns. Each sampling point had three consecutive dust filters (60, 20, 5 µm) to keep large particulate matter from plugging or contaminating the sample lines, the servo valves, or the delicate instruments.
Figure 1: Schematic representation of the monitoring system layout.
A positive-pressure gas sampling system (P-P GSS) was used in the MAEMU to minimize introduction of unwanted air into the sampling line. The P-P GSS consecutively pumped sample air from each sampling location using individual designated pumps. Air samples from each location were collected sequentially over a 2-min period via the controlled operation of servo valves of the PP-GSS. Each barn sampling location was sampled continuously every 14 min. A background ambient air sample was collected every two hours for 8 minutes. All pumps and the gas sampling system were leak checked weekly to ensure no contamination was occurring.
Pit fans at this facility had variable speeds, while the endwall fans had a single speed. All fans were calibrated in situ at multiple operation points to develop a performance or airflow curve for each fan. The in situ calibration of the exhaust fans was conducted with a fan assessment numeration system (FANS) (Gates et al. 2004). For single-speed fans (endwall), their airflow was a function of static pressure, whereas for variable-speed fans the airflow was a function of static pressure and fan speed (revolution per minute or RPM). Runtime of each fan was monitored continuously using an inductive current switch (with analog output) attached to the power cord of each fan motor (Muhlbauer et al. 2006). Each current switch's analog output was connected to the data acquisition system. Both barns were equipped with static pressure sensors located on the west end of each barn. Each pit fan's speed was continuously measured using Hall Effect speed sensors (GS100701, Cherry Corp, Pleasant Prairie, WI).
Gaseous Emission Rate Determination
Constituent emission rates were calculated as the mass of the gas emitted from the barn per unit time and expressed in the following form:
Where
ER[g] = Gas emission rate for the house, g hr-1 house-1
Qi , Qe = Incoming and exhaust ventilation rate of the house at field temperature and barometric pressure, respectively, m3 hr-1 house-1
[G]i ,[G]e = Gas concentration of incoming and exhaust ventilation air, respectively, ppmv
wm = molar weight of the gas, g mole-1 (e.g., 17.031 for NH3)
Vm = molar volume of gas at standard temperature (0°C) and pressure (101.325 kPa) or STP, 0.022414 m3 mole-1
Tstd = standard temperature, 273.15 K
Ta = ambient air temperature
ρi , ρe = density of incoming and exhaust air, respectively, g/cm3
Pstd = standard barometric pressure, 101.325 kPa
Pa = atmospheric barometric pressure at the monitoring site, kPa
Data presented in this paper cover the period of November 22, 2009 to March 11, 2010. Statistical analysis was performed using SAS 9.2. Data were analyzed using single factor ANOVA and considering each day as a repeated measure during the period. The dietary effect was considered significant at P-value < 0.05.
RESULTS AND DISCUSSION
The diets used during this study were formulated to meet the pigs' requirements as they grew towards market weight (NRC, 1998); the only difference between the control diet and the treatment diet was the inclusion of 22% DDGS. Including DDGS resulted in higher levels of crude protein, crude fiber, acid detergent fiber and sulfur compared to the traditional diet. The results to date are for nursery, starter and two finish phase diets. The nursery phase diets for either barn did not include DDGS.
The number of pigs in each barn was different, but the weight and age of the pigs were similar (Table 1). The average ventilation rate for barn 1 (Non DDGS) was 17966 m3 /hr and 16679 m3 /hr for barn 2 (DDGS). The ventilation rates for the two barns were not significantly different (Pvalue =0.0665).
The average daily ventilation rate for each barn and the corresponding outside temperature are shown in Figure 2a. The average in-house gas concentrations measured to date are shown in Table 1. Average NH3, CO2, H2S, N2O, and CH4 concentrations were 18 ppm, 3901 ppm, 337 ppb, 0.17 ppm and 140 ppm, respectively, for barn 1. For barn 2, the average concentrations for NH3, CO2, H2S, N2O, and CH4 were 24 ppm, 3719 ppm, 575 ppb, 0.2 ppm and 121 ppm, respectively. There was no significant difference in CO2 or N2O concentrations between the barns. However, NH3, H2S, and CH4 concentrations were significantly different (P-values: NH3 & H2S = 0.0001, CH4 =0.0393).
Figure 2
Table 1: Average number of pigs, ventilation rate, and in-house gas concentration values for wean-finish swine barns fed traditional (non-DDGS) or DDGS diet.
Emission Rates
Emission rates were expressed in kg/barn-d and g/pig-d. A statistical analysis was completed to determine if difference in emission rates between the two barns was significant.
The average emission rates for NH3, CO2, H2S, N2O, and CH4 in kg/barn-d are shown in Table 2. NH3, CO2, H2S, and CH4 were all significantly different with P-values less than 0.05. Although CO2 and CH4 emissions were significantly different between the two barns in terms of kg/barn-d, they were not significantly different in terms of g/pig-d (i.e., when the number of animals in the two barns was accounted for). N2O was not significantly different between the barns. Emission rates for NH3, CO2, H2S, N2O, and CH4 expressed in g/pig-d are presented in Table 3.
Table 2: Average gaseous emission rates (kg/barn-d) of the monitored W-F swine barns fed non-DDGS or DDGS diet
Table 3: Average gaseous emission rates (g/pig-d) of the monitored W-F swine barns fed non-DDGS or DDGS diet
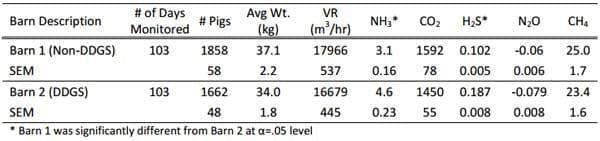
NH3 and H2S emission rates were significantly different between the two barns after the number of pigs was considered. This was likely caused by the higher in-house gas concentrations found in barn 2 (DDGS) for NH3 and H2S. Figure 3a and 3c show the average daily emissions for NH3 and H2S for the reported period. These results were comparable to similar studies investigating the effects of feeding dried distillers grains with solubles (Powers et al. 2008 & 2006). The increase in H2S concentrations could be attributed to the addition of sulfur contained in the DDGS diet, especially since the two barns shared the same water source. More investigation is needed to determine if sulfur from feedstuffs is the only influencing factor. Higher ammonia concentrations in barn 2 (DDGS) could be caused by the increase of ammoniacal nitrogen excreted when pigs are fed more dietary protein (Kerr et al. 2006), as is the case when feeding DDGS.
According to Pedersen and Sallvik (2002), manure accounts for only 4% of CO2 produced in livestock facilities. Therefore, the inclusion of DDGS was not expected to affect the CO2 emissions from barn 2 (DDGS). The significant difference in CO2 emissions per barn was no longer significant when the emissions were expressed on a per-pig basis (P-value=0.1391). The difference was likely caused by the higher animal numbers in barn 1(Non DDGS) and increased respiration. CH4 emissions reported as kg/barn-d were significantly different between the two dietary regimens. This difference was likely caused by the increased amount of manure excreted in barn 1, which became non-significant when the number of pigs in each barn was considered. Both CO2 and CH4 emissions increased with pig weight, as shown in Figure 3b and 3e, caused by increased metabolic rate (thus respiratory CO2 production) and manure excretion as pigs grow.
Nitrous oxide was not significantly different between the barns. As shown in Figure 3d, the N2O emission rates ranged from -0.02 to 0.01 g/pig-d. The detection limit of the instrument for N2O measurement was 0.5 ppm. Since the concentrations measured inside the barns and outside were lower than the detection limit of the instrument (Table 1), it is likely that the range of N2O emissions stemmed from the instrument noise.
CONCLUSIONS
The results from this study indicated feeding DDGS increased aerial ammonia emission from 3.1 g/pig-d to 4.6 g/pig-d and hydrogen sulfide emissions from 0.10 g/pig-d to 0.19 g/pig-d, but had no effect on greenhouse gases (GHG) emissions. These results are comparable to Powers et al. 2006 & 2008. CO2 emissions were not different between barns, and when reported as g/pig-d, there was no difference between the diets (P-value = 0.1391). The amount of nitrous oxide emitted from the barns and present in the atmosphere was below the detection limit of the instrument used and therefore any variation was attributed to instrument noise. There was no significant difference found for methane emissions between barns in terms of g/pig-d. However, there was a significant difference in methane concentrations between barn 1 and barn 2 with a P-value of 0.0393. By feeding DDGS, the methane concentrations were lower in barn 2 than barn 1. This is comparable to a study by Powers et al. (2006) which reported DDGS diets produced lower methane concentrations than traditional corn diets.
This is an ongoing study that will provide more data to determine if the findings in this paper are representative of aerial emissions year round or if there is seasonal variation for emissions from swine facilities feeding DDGS. There also needs to be more investigation into other causes of higher hydrogen sulfide and ammonia in-house concentration levels.
Figure 3: Aerial emissions in g/pig-d for a. Ammonia, b. Carbon Dioxide, c. Hydrogen Sulfide, d. Nitrous Oxide, e. Methane. Gaps represent time periods of power or instrument failure.
Acknowledgements
We would like to thank the National Pork Board for the funding to complete this study.
REFERENCES
1. Gates, R. S., K. D. Casey, H. Xin, E. F. Wheeler, J. D. Simmons. 2004. Fan Assessment Numeration System (FANS) design and calibration specifications. Transactions of the ASAE, 47(6):1765-1778.
2. Honeyman, M., P. Lammers, S. Hoyer. 2007. Feeding bioenergy co-products to swine. Iowa State University Extension Publication #IPIC 11a. Iowa Pork Industry Center. May 2007.
3. Kerr, B., C. Ziemer, S. Trabue, J. Crouse, T. Parkin. 2006. Manure composition of swine as affected by dietary protein and cellulose concentrations. Journal of Animal Science 2006. 84:1584-1592
4. Li, H., H. Xin, Y. Liang, R. S. Gates, E. F. Wheeler, and A.J. Heber. 2005. Comparison of direct vs. indirect ventilation rate determinations in layer barns using manure belts. Transactions of the ASAE 48(1): 367-372.
5. Moody, L., H. Li, R. Burns, H. Xin, R. Gates. 2008 A Quality Assurance Project Plan for Monitoring Gaseous and Particulate Matter Emissions from Broiler Housing. ASABE #913C08e. St Joseph, Michigan.
6. Muhlbauer, R. V., T. A. Shepherd, H. Li, R. T. Burns, H. Xin. 2006. Development and testing of a fan monitoring system using induction operated current switches. ASABE Technical Paper, St. Joseph, Michigan
7. NRC. 1998. Nutrient Requirements of Swine. 10th rev. ed. Natl. Acad. Press, Washington, DC.
8. Pedersen, S., and K. Sallvik, eds. Climatization of Animal Houses Heat and Moisture Production at Animal and House Levels. Rep. International Commission of Agricultural Engineering, Section II, 2002.
9. Powers W. J., S. B. Zamzow, B. J. Kerr. 2008. Diet Modification as a Mitigation Tool for Swine Production. Proceedings of the Livestock Environment VIII Conference. ASABE. #701P0408. Iguassu Falls, Brazil.
10. Powers. W., B. Kerr, K. Stalder. 2006. Influence of corn co-products on air emissions and nutrient excretions from grow-finish swine. NPB #05-111. Research Report. National Pork Board Research Database.
11. Powers, W. 2003. Gaseous emissions from animal agriculture. Iowa State University Extension Publication #PM 1935. March 2003. http://www.extension.iastate.edu/Publications/PM1935.pdf
12. Shurson, G.C., M.J. Spiehs, J.A. Wilson, and M.H. Whitney. 2003. Value and use of 'new generation' distiller's dried grains with solubles in swine diets. Presented at the 19th International Alltech Conf., Lexington, KY. May13, 2003. http://www.ddgs.umn.edu/info-swine.htm
13. Spiehs M.J., M. H. Whitney, G. C. Shurson, R. E. Nicolai, J. A. Renteria-Flores. 2000 Odor Characteristics of Swine Manure and Nutrient Balance of Grow-Finish Pigs Fed Diets with and without Distillers Dried Grains with Solubles. Journal of Animal Science 78:69 (Suppl. 2)
14. Sutton A., K. Kephart, M. Verstegen, T. Canh, P. Hobbs. 1999. Potential for reduction of odorous compounds in swine manure through diet modification. Journal of Animal Science 1999. 77:430:439