IntroductionMycotoxins are secondary products of fungal metabolism that may beproduced in contaminated feeds during production and storage. Thesemetabolites are generally associated with a group of ubiquitous fungibelonging to the Fusarium, Aspergillus, Penicillium and Claviceps speciesthat grow on forages and grains in the field and during storage (Ledoux andRottinghaus, 1999). It has been estimated that at least 300 fungal metabolitesare potentially toxic for man and animals, and that as much as 25% of theworld's cereal grains are contaminated with measurable levels of mycotoxins(Devegowda et al., 1998). Fungal growth in silages, stored forages and byproductscan result in toxin formation. As a result, mycotoxins can be foundin many types of feed materials and are commonly encountered in livestockproduction systems. As a group, mycotoxins are chemically diverse andhave a broad range of physiological activities in animals. These toxins aretypically present at low concentrations, even in highly contaminated feeds.However, many are produced at concentrations known to have significanteffects on animal health and may have a major impact on animal production.The negative influence on the growth and health of livestock makes them amajor problem in many production systems.
There are a broad array of fungal metabolites that can be toxic (Figure 1).Among these, aflatoxins are the most common in the tropical and subtropicalregions where warm environmental temperatures allow for the growth ofthe Aspergillus species during storage. These toxins can cause liver damage,decrease reproductive performance, cause tumor formation and suppressimmune functions.
In contrast, fusarium toxins are more commonly encountered in temperateregions, where temperatures between 45º and 75ºF allow the fungus toinfect not only stored feeds, but also the growing corn plants before harvest.
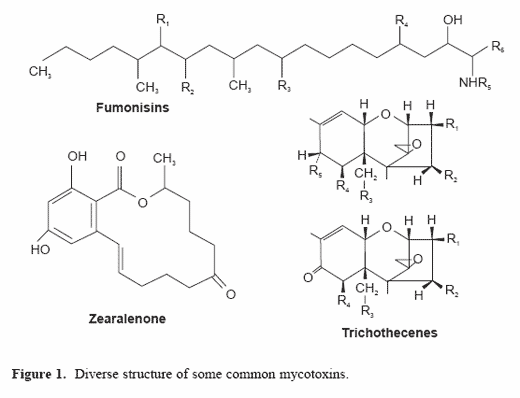
The fungi in the Fusarium species produce more than 100 metabolites thatare potentially toxic in animals. These include trichothecenes (deoxynivalenol,T-2 toxin, HT-toxin and diacetoxyscirpenol), zearalenone, fumonisins,moniliformin and fusaric acid. It is unlikely that any one of these toxinswould be produced individually in great quantities. As a result, toxicosisassociated with these fungi is often a complex process involving a numberof toxins (Smith et al., 2000). The fusarium toxin, deoxynivalenol (DON), isknown for its ability to inhibit protein synthesis and has been shown toincrementally decrease intake in pigs at concentrations above 2 ppm(Newman, 2000). It can also influence reproductive performance and isassociated with immunosuppression (Johnson et al., 1997). T-2 and relatedtoxins can cause irritation and hemorrhages in the gastrointestinal tract. Insevere cases, T-2 causes oral lesions in poultry and swine. Zearalenone isknown for its estrogenic activities and its ability to impair reproductiveperformance. At low concentrations it influences the development ofreproductive organs and causes rectal and vaginal prolapses. Fumonisin isknown for its ability to impair immune function and cause kidney and liverdamage. As a result, it can have a significant impact on animal performanceand the incidence of disease in many species.
Other fungal-associated toxins have been identified in forages. These areoften not traditionally classified with the mycotoxins associated with grainsand prepared animal feeds, but are the result of synergistic metabolic activitiesthat develop from endophytic fungal infections in forages. These toxins are commonly associated with grazing animals maintained on tall fescue andperennial ryegrass. They also include the ergot alkaloids found in sorghum(Deo et al., 1999).
Controlling mycotoxicosesA number of approaches have been used to control the adverse effects ofmycotoxins in animal production systems. Many of the strategies that preventthe formation of mycotoxins in feeds have merit and provide an initial line ofdefense against toxin-induced health problems. The objective of thesestrategies is to prevent the growth of fungi and the formation of toxin byaltering feed management practices. Practices that keep feed moisture levelslow or that prevent damage of grains during processing can be effective indecreasing the prevalence of mycotoxin-producing fungi in the feeds, butmay not always eliminate toxin formation.
Strategies that use microbial or thermal inactivation of toxins, physicalseparation of contaminated feedstuffs, irradiation, ammoniation and ozonedegradation have all been examined as tools for destroying or modifyingmycotoxins (CAST, 1989; McKenzie et al., 1998). However, these strategiestend to be costly or time consuming and have not been found to be ofpractical use. A number of strategies are based on nutritional manipulationthat can help overcome the toxic effects of ingested toxins. These strategiesare based on the fact that many subtle effects of mycotoxins can beovercome by maintaining animals in a healthy, disease-free environment.The use of antioxidants to control tissue damage associated with mycotoxinshas been investigated by a number of research groups (Lin et al., 1994;Atroshi et al., 1995; Grosse et al., 1997; Ibeh and Saxena, 1998). It is clearfrom these studies that antioxidants can act as mycotoxin antagonists andhelp maintain the tissue integrity of animals exposed to certain types ofmycotoxins. Other nutritional approaches have used specific phenoliccompounds to detoxify mycotoxins (Aboobaker et al., 1994); and compoundslike aspartame (Baudrimont et al., 1997), piperine (Reen et al., 1997),coumarin (Goeger et al., 1998), chlorophyll derivatives (Dashwood et al.,1998) and serotonin antagonists (Prelusky et al., 1997) have been used aschemoprotectants against the negative effects of mycotoxins. However,most studies of these potential strategies have been performed in basicbiochemical test systems, and it is not clear how the protective effects ofsuch strategies can be used to define nutritional approaches for control ofmycotoxin-associated toxicosis. As a result, such strategies have not becomewidespread practical applications in animal production systems.
Another approach for attenuating the effects of mycotoxins is based onthe use of specific materials that adsorb mycotoxins in animal feeds. Thesehave become some of the most practical methods for controlling mycotoxinsin feeds. They are based on the ability of the adsorbents to 'tie up' or 'bind' the toxins. This allows the toxins to pass through an animal's digestive tractwithout being absorbed. Both inorganic and biological adsorbents have beenexamined and used to control the bioavailability of mycotoxins. Inorganicclay-based adsorbents and activated charcoal have been shown to adsorbspecific mycotoxins and are attractive as feed supplements because theyare relatively inert from a nutritional standpoint. These include hydratedsodium calcium aluminosilicates (HSCAS), zeolites, bentonites, specific claysand activated charcoals prepared from different sources (Piva et al., 1995).For the most part, these strategies are known for their ability to bind aflatoxins,but adsorption to other types of mycotoxins has also been examined (Table1).
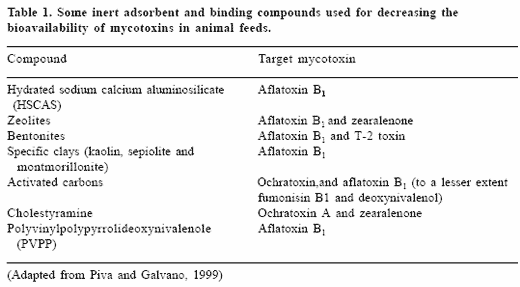
There has been a great deal of interest in using natural biological productsto reduce the bioavailability of mycotoxins in animal production systems.One available strategy for attenuating the effects of some groups ofmycotoxins uses the unique adsorptive capacity of the carbohydratecomplexes in the yeast cell wall. The potential usefulness of these types ofmaterials was first demonstrated in poultry in the early 1990s. Initially usedas a nutritional aid and a growth promoter, a commercially available viableyeast culture preparation based on Saccharomyces cerevisiae strain 1026(Yea-Sacc), was found to improve hatchability (McDaniel, 1991) and broilerbody weights (Stanley et al., 1993). Investigators attributed the yeast culturepreparation's ability to alter growth patterns of poultry to its ability to bindtoxins found in the diets used in these studies. In controlled studies, viableyeast cultures added to broiler diets containing aflatoxin resulted in significantimprovement in weight gain and enhanced immune response (Devegowdaet al., 1995). Additionally, in vitro studies clearly established up to 90%adsorption of aflatoxin to yeast cells in a dose-dependent fashion (Devegowdaet al., 1994). This work was the basis for a set of new strategies that use yeast-derived products to overcome problems associated with mycotoxincontaminated feeds.
Studies with whole yeast cells have led to a closer evaluation of specificcomponents in yeast cultures and their ability to bind mycotoxins. Modificationin manufacturing techniques have allowed for the production of a specificmodified yeast cell wall preparation with the ability to bind a range ofmycotoxins (Table 2). The yeast cell wall-derived glucomannan product,Mycosorb, has also been repeatedly shown to reduce the toxic effects ofmycotoxin-contaminated grains in poultry (Smith et al., 2000), leaving littledoubt about the nature of the active components. Data from these andsimilar studies suggest that organic adsorbents prepared from the yeast cellwall of specific strains of S. cerevisiae may play a critical role in strategiesfor controlling the toxicity of mycotoxins in poultry feeds.
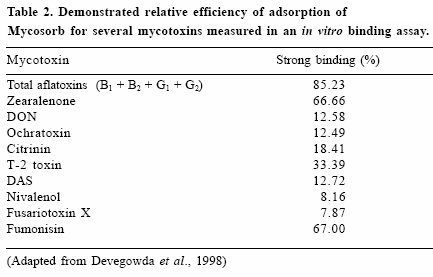
In many respects, the organic adsorbent derived from yeast overcomes theinherent drawbacks associated with using some of the inorganic adsorbentsthat have traditionally been used to address intoxication problems. Claybasedadsorbents are typically used at high concentrations (>1.0% of thediet) in animal feeds. This can decrease the nutrient density of the diet andprovide an excess adsorbent capacity that may decrease the bioavailabilityof important micronutrients. In contrast, the high adsorptive capacity of theyeast cell wall-derived binders make it possible for them to provide beneficialactivities at much lower concentrations (<0.1% of the diet). Since thesepreparations are designed to adsorb to mycotoxins at low concentrations,their inclusion would have minimal effect on the nutrient density of thediets. In addition, these adsorbents would not be expected to influence theconcentrations of micronutrients that are typically present in the diet atconcentrations greater than those of contaminating mycotoxins.
In vitro measures of mycotoxin bindingIn order to understand the best way to use specific mycotoxin adsorbents, itis important to understand the basic mechanisms of interactions with specifictoxins. While in vitro adsorption assays may not always be indicative of thein vivo responses to specific mycotoxins, these types of laboratory studiescan be used to define approximate dose requirements for an adsorbent anddefine a strategic mechanism for using a particular adsorbent in an animalsystem.
A number of techniques have been used to measure in vitro mycotoxinbinding or adsorption. The simplest of these tests measure adsorption ofpurified toxin preparations in an aqueous medium. In these systems, a knownamount of mycotoxin is reacted with a known amount of adsorbent in water.The amount of toxin remaining in the liquid after separation of the mycotoxinbindercomplex is then quantitatively determined and the amount of adsorbedtoxin is estimated by difference. Because of the relative insolubility of themycotoxins, these tests are generally run at very low mycotoxinconcentrations. The ability of the assay system to accurately measureadsorption is limited by the detection limits of the mycotoxin assay system.The increased sensitivity of high performance liquid chromatographictechniques has made routine evaluation of many mycotoxin adsorbents morepractical for many laboratories. These types of assay systems have beenused to quantitatively study the binding of adsorbents to aflatoxins (Ledouxand Rottinghaus, 1999).
Other test systems used to evaluate mycotoxin binding are based onmeasuring adsorption in a two-step process. These systems attempt tomeasure the strength of the mycotoxin binding by initially measuring thequantity of toxin bound in an aqueous system, and then measuring thedesorption of the mycotoxin after exposure to a second solvent system.The adsorption efficiency compares the initial binding (weak binding) withthe binding after desorption (strong binding). Such tests for strong mycotoxinbinding have been used to measure the relative efficacy of the yeast cellwall-derived glucomannan (Table 2). As might be expected from the diversestructural differences in mycotoxins, the yeast cell wall material displaysvariations in adsorption efficiencies, depending on the mycotoxin. Whilesuch tests are useful in demonstrating in vitro binding, they do not providemuch information for comparing different types of adsorbents or anunderstanding of how a specific adsorbent can be used in a practical feedmanagement program. Since an ideal adsorbent would be most effective atthe relatively low mycotoxin levels found in the gastrointestinal tract, thesein vitro tests at relatively high mycotoxin concentrations give a poorrepresentation of the adsorbent’s true usefulness.
Considerable information on the adsorption of mycotoxins to specificbinders can be obtained by using adsorption isotherms (Ramos andHernandez, 1996; Grant and Phillips, 1998). These systems recognize thatmycotoxin adsorption in biological systems is a reversible process that can be characterized as a chemical equilibrium. As a result, adsorption is aconcentration-dependent process influenced by mycotoxin concentration,the amount of adsorbent, and the relative affinity of the adsorbent for themycotoxin. Adsorption isotherms plot the amount of mycotoxin adsorbedper unit weight of adsorbent versus the mycotoxin concentration in solutionat equilibrium and constant temperature (Figure 2). These tests can be usedto compare the relative binding capacity and affinity of adsorbents. Theyclearly can be used to demonstrate differences in clay-based adsorbentsand those derived from yeast cell wall preparations.
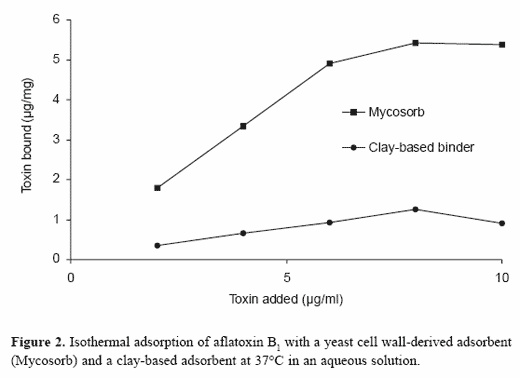
Grant and Phillips (1998) were able to use multiple isotherm equations todetermine not only full capacity and affinity, but also average capacity,enthalpy of binding, heterogeneity coefficient, multiple site distributioncoefficients, and multi-site capacity of HSCAS for aflatoxin B1. Similarly,we have used these techniques to compare the adsorption of aflatoxin B1 tothe yeast cell wall preparation, Mycosorb, and to a clay-based adsorbent(Figure 2). In these studies, major differences were noted in the overalladsorption capacity of the two commercial mycotoxin clay-based adsorbentsand that of Mycosorb at high mycotoxin concentrations. A measure of theefficiency of the mycotoxin binder over a wide range of specific mycotoxinconcentrations can also be used to describe and compare the role of specificadsorbents at low mycotoxin concentrations. Mycosorb was a more effectiveadsorbent at low mycotoxin concentrations than were either of the clay-based binders tested (Figure 3). This reflects the higher affinity of Mycosorbfor aflatoxin and suggests that this material would be more effective at thelow mycotoxin concentrations found in the gastrointestinal tract. The yeastcell wall-derived adsorbent also tended to bind a greater proportion ofmycotoxin at relatively high mycotoxin concentrations. This suggests thatthese types of adsorbents have a greater overall capacity to bind themycotoxin and can be used at lower inclusion rates. The combination ofhigh affinity for aflatoxins and lower inclusion rates makes Mycosorb anattractive supplement for controlling aflatoxicosis. These observations areconsistent with the efficacy of the product in controlling aflatoxin uptake indairy cattle (Whitlow et al., 2000).
Characteristics of aflatoxin binding
In vitro studies have also helped to characterize the interactions betweenthe yeast cell wall-derived adsorbents and specific mycotoxins. Serial elutionof the aflatoxins adsorbed to the glucomannan clearly demonstrates thatthe adsorption of the mycotoxin is reversible, and that aflatoxins are notmodified during the adsorption process. This is important, since it clearlyestablishes the basic kinetic mechanisms associated with adsorption andlack of an extensive chemical modification during adsorption. Aflatoxinadsorption is also influenced by the pH (relative acidity) in the aqueousenvironment. Maximum binding occurs at a pH of approximately 4.0. Inaddition, adsorption is also influenced by the relative phosphate concentrationsin an aqueous environment (Figure 4). Maximum binding was observed in a phosphate buffer that contained 0.5 M phosphate. Both pH and phosphateconcentration optima are consistent with those found in the gastrointestinaltract and suggest that the conditions in the gastrointestinal tract wouldenhance adsorption and not decrease the mycotoxin-adsorbent interactions.
Improving mycotoxin binding using yeast cell wall derivative Recent studies have examined the effects of chemical modifications of yeast cell wall glucans on the basic adsorption of a wide variety of mycotoxins. Specific chemical modification of the surfaces associated with the basic glucan structure has made it possible to tailor derivatives with activities that are mycotoxin-specific. One such derivative is specific for zearalenone (Figure 5). This particular derivative has a greater capacity to adsorb zearalenone across a broad concentration range and has a greater affinity for zearalenone than the original glucomannan preparation. These chemical modifications can be made without influencing the particulate nature of the adsorbent and allow for the development of mycotoxin-specific activities based on the structure of the toxins. Since the surface area of the modified yeast cell wall is not significantly different from the original yeast cell wall preparations, it appears that adsorption in these systems is not always correlated with the surface area, but is in some cases related to the chemical nature of the adsorbent surface. These studies suggest that the basic glucan structure derived from crude yeast cell wall preparations may provide a basic structural unit for more advanced mycotoxin adsorbents in the future.
Conclusions
Mycotoxins and their associated health problems are common in modernlivestock and poultry production systems. Adsorbents that bind mycotoxinsand decrease their bioavailability show a great deal of promise as tools foruse in strategies that attenuate mycotoxin-induced toxicosis. The high affinityand high adsorption capacity of yeast-derived glucomannan preparationsmake their use as adjuncts for controlling naturally occurring mycotoxins infeeds attractive.
ReferencesAboobaker, V.S., A.D. Balgi and R.K. Bhattacharya. 1994. In vitro effectof dietary factors on the molecular action of aflatoxin B1: Role of nonnutrientphenolic compounds on the catalytic activity of liver fractions. InVivo 8:1095-1098.
Atroshi, T., A. Rizzo, I. Biese, M. Salonen, L.A. Lindberg and H. Saloniemi.1995. Effects of feeding T-2 toxin and deoxynivalenol on DNA and GSHcontents of brain and spleen of rats supplemented with vitamin E and Cand selenium combination. J. Anim. Phys. Anim. Nutr. 74:157-164.
Baudrimont, I., A.M. Betbeder and E.E. Creppy. 1997. Reduction of theochratoxin A-induced cytotoxicity in Vero cells by aspartame. Arch.Toxicol. 71:290-298.
Council for Agricultural Science and Technology (CAST). 1989. Task ForceReport #16-Mycotoxins: Economic and Health Risk. Ames, IA.
Dashwood, R., T. Negishi, H. Hayatsu, V. Breinholt, J. Hendricks and G.Bailey. 1998. Chemopreventative properties of chlorophylls towardaflatoxin B1: A review of the antimutagenicity and anticarcinogenicitydata in rainbow trout. Mutat. Res. 399:245-514.
Deo, P., B.J. Blaney and J.G. Dingle. 1999. Effects of mineral and organicadsorbents in meat chicken diets contaminated with sorghum ergot alkaloid.Queensland Poult. Sci. Symp. Vol. 8.
Devegowda, G., B.I.R. Aravind, K. Rajendra, M.G. Morton, A. Baburathnaand C. Sudarshan. 1994. A biological approach to counteract aflatoxicosisin broiler chickens and ducklings by the use of Saccharomyces cerevisiaecultures added to feed. In: Biotechnology in the Feed Industry, Proceedingsof the 10th Annual Symposium (T.P. Lyons and K.A. Jacques, eds).Nottingham University Press, Loughborough, Leics, UK. pp 235-245.
Devegowda, G., B.I.R. Aravind, M.G. Morton and K. Rajendra. 1995. Abiotechnological approach to counteract aflatoxicosis in broiler chickensand ducklings by the use of Saccharomyces cerevisiae. Proc. FeedIngredients Asia ’95, Singapore. pp 161-171.
Devegowda, G., M.V.L.N. Raju and H.V.L.N. Swamy. 1998. Mycotoxins:Novel solutions for their counteraction. Feedstuffs, December 7, 1998;12:15.
Grant, P.G. and T.D. Phillips. 1998. Isothermal adsorption of aflatoxin B1 onHSCAS clay. J. Agric. Food Chem. 46:599-605.
Goeger, D.E., K.E. Anderson and A.W. Hsie. 1998. Coumarinchemoprotection against aflatoxin B1-induced mutation in a mammaliancell system: A species difference in mutagen activation and protectionwith chick and rat liver S9. Environ. Mol. Mutgen. 30:64-74.
Grosse, Y., L. Chekir Ghedira, A. Huc, S. Obrecht Pfumio, G. Dirheimer,H. Bacha and A. Pfohl Leszkowicz. 1997. Retinaol, ascorbic acid andalpha-tocopheroal prevent DNA adduct formation in mice treated withmycotoxins ochratoxin A and zearalenone. Cancer Lett. 114:225-229.
Ibeh, I. N. and D. K. Saxena. 1998. Effect of alpha-tocopherol supplementationon the impact of aflatoxin B1 on the testes of rats. Exp. Toxicol.Pathol. 50:221-224.
Johnson, P.J., S.W. Casteel, and N.T. Messer. 1997. Effect of feedingdeoxynivalenol (vomitoxin)-contaminated barley to horses. J. Vet. Diagn.Investigation. 9:219-221.
Ledoux, D.R. and G.E. Rottinghaus. 1999. In vitro and in vivo testing ofadsorbents for detoxifying mycotoxins in contaminated feedstuffs. In:Biotechnology in the Feed Industry. Proceedings of the 15th AnnualSymposium. (T.P. Lyons and K.A. Jacques, eds), Nottingham UniversityPress, UK. pp 369-379.
Lin, Z.H., S.G. Li, L.Y. Wu, S. Sun and Q.W. Lu. 1994. Antagonistic effectof Se on the T-2 toxin-induced changes in the ultrastructure andmitochondrial function of cultured chicken embryonic chondrocytes. J.Clinical Biochem. Nutr. 17:119-132.
McDaniel, G. 1991. Effect of Yea-Sacc1026 on reproductive performanceof broiler breeder males and females. In: Biotechnology in the FeedIndustry. Proceedings of the 7th Annual Symposium. Alltech TechnicalPublications, Nicholasville, Kentucky, USA.
McKenzie, K.S., L.F. Kubena, A.J. Denvir, T.D. Rogers, G.D. Hitchens,R. H. Bailey, R.B. Harvey, S.A. Buckley and T.D. Phillips. 1998.Aflatoxicosis in turkey poults is prevented by treatment of naturallycontaminatedcorn with an ozone generated by electrolysis. Poultry Sci.77:1094-1102.
Newman, K. 2000. The biochemistry behind esteried glucomannans- tiratingmycotoxins out of the diet. In: Biotechnology in the Feed Industry.Proceedings of the 16th Annual Symposium. (T.P. Lyons and K.A. Jacques,eds), Nottingham University Press, UK.. pp 369-382.
Piva, A. and F. Galvano. 1999. Nutritional approaches to reduce the impactof mycotoxins. In: Biotechnology in the Feed Industry. Proceedings of the15th Annual Symposium. (T.P. Lyons and K.A. Jacques, eds), NottinghamUniversity Press, UK.. pp 381-399.
Piva, G., F. Galvano, A. Pietri and A. Piva. 1995. Detoxification methods ofaflatoxins. A review. Nutr. Res. 5:689-715.
Prelusky, D.B., B.A. Rotter, B.K. Thompson and H.L. Trenholm. 1997.Effects of appetite stimulant cyproheptadine on deoxynivalenol-inducedreductions in feed consumption and weight gain in the mouse. J. Environ.Sci. and Health. 32:429-448.
Ramos, A.J. and E. Hernandez. 1996. In vitro aflatoxin adsorption by meansof a montmorillonite silicate. A study of adsorption isotherms. AnimalFeed Sci. Technology 62:263-269.
Reen, R.K., F.J. Wielbel and J. Singh. 1997. Piperine inhibits aflatoxin B1-induced cytotoxicity and genotoxicity in V79 Chinese hamster cellsgenetically engineered to express rat cytochrome P4503B. J.Ethnopharmacology 58:165-173.
Smith, T.K., M. Modirsanei and E.J. MacDonald. 2000. The use of bindingagents and amino acids supplements for dietary treatment of Fusariummycotoxicoses. In: Biotechnology in the Feed Industry. Proceedings ofthe 16th Annual Symposium. (T.P. Lyons and K.A. Jacques, eds),Nottingham University Press, UK. pp 383-390.
Stanley, V.G., R. Ojo, S. Woldesenbet and D.H. Hutchinson. 1993. The useof Saccharomyces cerevisiae to suppress the effects of aflatoxicosis inbroiler chicks. Poultry. Sci. 72:1867-1872.
Whitlow, L.W., D.E. Diaz, B.A. Hopkins and W.M. Hagler. 2000.Mycotoxins and milk safety: The potential to block transfer to milk. In: Biotechnology in the Feed Industry. Proceedings of the 16th AnnualSymposium. (T.P. Lyons and K.A. Jacques, eds), Nottingham UniversityPress, UK. pp 391-408.