INTRODUCTION
Preservation of alfalfa as silage is becoming convenient due to the ease of mechanization and reduced susceptibility to weather damage (Khadem et al. 2009). Calberry et al. (2003); Bhandari et al. (2008) and Khadem et al. (2009) reported that cows fed on ration containing chopped alfalfa hay had lower intake than those fed on a diet containing chopped alfalfa silage. But similar milk yield and composition (Calberry et al. 2003; Khadem et al. 2009). There are reports that reduction in dietary particle size (PS) decreases milk fat percentage (MF), chewing activity, acetic acid and rumen pH (Teimouri et al. 2004; Bhandari et al. 2008; Beauchemin et al. 2008; Khadem et al. 2009). Particle size reduction can leads to an increase in consumption due to an enhancement in the passage rate and bites taken (Calberry et al. 2003; Teimouri et al. 2004; Bhandari et al. 2008).Boerman and Lock (2014) also showed that adding fat in diet has not negative affect on dry matter intake (DMI), milk yield (MY), milk composition, blood metabolite and ruminal parameters. Schroeder et al. (2002) indicated that adding fat to the diet reduce C18:2 and C18:3 amounts, but C18:1 and C18:0 remain unchanged. Onetti et al. (2004)illustrated that supplementing tallow has negative effects on dry matter intake, milk yield, milk fat, rumen fermentation and C18:3 in milk of high producing dairy cows in mid-lactation. Therefore, our hypothesis was that decreasing alfalfa silage particle length and fat supplementation to dairy cow rations would depress ruminal pH, which in turn, would reduce ruminal fatty acids biohydrogenation, thus potentially increasing unsaturated fatty acids flow to the small intestine and their incorporation into milk fat.
MATERIALS AND METHODS
Alfalfa hay and silage
Alfala hay during second cut was harvested having 190 g dry matter per kg of fresh matter. The regional humidity was 30% and there was no rainfall during the harvest. The forages were field-wilted for 12 h to reach at about 300 g/kg dry matter content before ensiling. The rest of the crop was kept on the farm for 72 h before being baled and stored as alfalfa hay (AH). Before storage, both the hay and silage forms of alfalfa forage were chopped at 15 mm and 25 mm sizes using a chopper (Jaguar 62, Germany). Molasses was added to the alfalfa during silage making in trench silos and ensiled for 60 days. Samples of hay and silage forms of alfalfa were collected and stored at -20 ˚C for chemical analysis. Fat was added to their respective concentrates prior to addition of forages, followed by thorough mixing.
Animals, diets and experimental design
Twenty four multiparous Holstein dairy cows in early lactation period from Shaghayegh-Dam Company with average body weight and milk yield of 550 ± 24 kg and 38.5 ± 0.90 kg were used in this study. Animals were managed according to the guideline set by the Canadian Council on the Animal Care (NRC, 2001). Cows were housed in individual tie stalls except when being milked in the milking parlor. This experiment was conducted in 2 × 4 factorial arrangements by completely randomized design for 100 days. Fac-tors were particle size (15 and 25 mm) and two different sources of alfalfa supplemented with and without fat (alfalfa hay; alfalfa hay plus 3% fat; alfalfa silage and alfalfa silage plus 3% fat).
The diets were offered as a total mixed ration three times daily at 05:00, 13:00 and 21:00 h and residue from the previous feeding was collected and subtracted from the amount fed. The amount of feed offered was adjusted daily to obtain approximately 5-10% residue on as fed basis. Cows were milked three times a day at 04:00, 12:00 and 20:00 h and had free access to fresh water (Table, 1).
Data collection
The concentrate-to-forage ratio in the diets was 55:45 on dry matter basis. Feed samples were collected weekly during the experimental period, dried at 60 ˚C for 48 h, ground to pass through a one mm screen. The crude protein content was determining by micro-Kjeldahl analysis (AOAC, 2002).
Neutral detergent fiber was determined using α-amylase, acid detergent fiber, sodium sulphite and corrected for ash concentration according to Van Soest et al. (1991).
Fatty acids were determined after the procedure described by Schroeder et al. (2002) after extracting fat from the samples. Daily milk yield of each cow was recorded and then daily energy corrected milk (ECM) was calculated [ECM (kg/d)= (0.327×milk yield, kg)+(12.96×milk fat, kg) + (7.2×milk protein, kg)]. Milk samples were collected from three consecutive milking times each day and divided in two parts. The first part was preserve in bronopol and stored at 4 ˚C before analysing for milk fat (MF), milk protein, somatic cell count and milk urea nitrogen (MUN) concentrations by a Foss Milk-o-Scan FT 5000 (at the PAK dairy co., Iran). A second part was stored at -20 ˚C until analysis of conjugated linoleic acid (CLA). Before lipid extraction, milk samples were thawed in water at 36 ˚C for 30 min. Lipids extracted and methylated following the procedure described by Chouinard et al. (1997). CLA was determined using a gas chromatograph (Shimadzu Co., Japan) equipped with a 100-m CP-Sil-88 capillary column and a flame ionization detector.
The body weight of cows was recorded in 20-day intervals prior the morning feeding. Body condition scores (BCS) recorded at weighing on a scale of 1 (thin) to 5 (fat) in increments of 0.25 (Harvatine and Allen, 2005). Feeding behaviour, including the total time spent eating, ruminating and resting was monitored and recorded for each cow in 5-min intervals using a chronometer (Khadem et al. 2009). Feeding behaviour measurements was conducted for 24 h periods on days 19, 38, 57, 76 and 95 of the data collection period. The average daily total chewing time (min/day) was also calculated by adding the daily eating and ruminating times of each cow.
Rumen fluid was taken (about 200 mL) from each cow three hours after morning feedings at 20-days intervals, using a Geishauser oral probe. Samples were then strained with two layers of cheese cloth and the pH was measured immediately using a pH meter. Five mL of the strained rumen fluid samples was taken and stored at -20 ˚C after the addition of 1 mL 25% metaphosphoric acid solution for volatile fatty acids (VFA) analysis. 10 mL samples were also taken and stored at -20 ˚C for measuring ruminal NH3-N. For the VFA measurement of rumen fluid, frozen samples were thawed at room temperature and centrifuged at 6000 g for 10 min.
The VFA were determined by gas chromatograph (0.25×0.32, 0.3 μm i.d. fused silica capillary, model no. CP-9002 Vulcanusweg 259 a.m., Chrompack, Delft, the Netherlands) as outlined by Kowsar et al. (2008). NH3-N was measured by the colorimetric phenol-hypochlorite method of Broderick and Kang (1980).
Table 1 Ingredients and chemical composition of the treatments
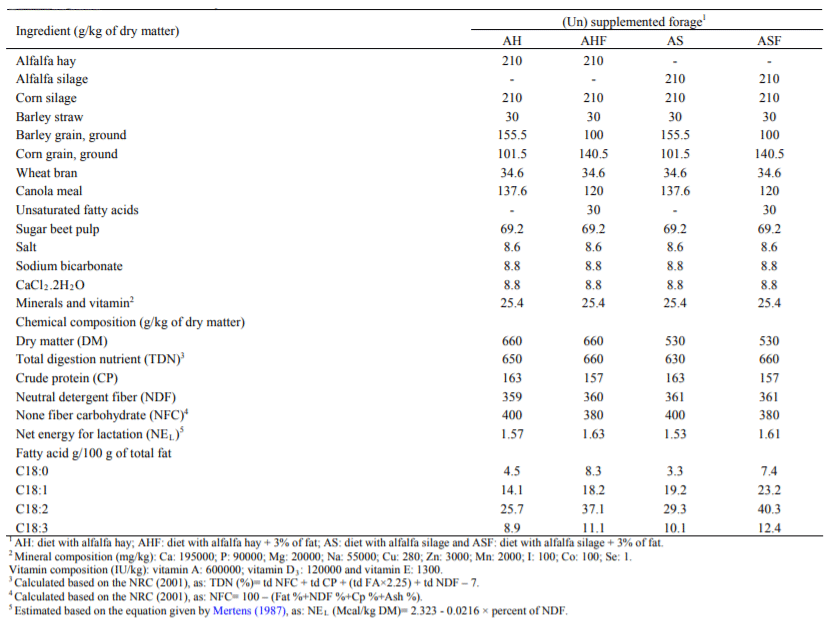

Click here to enlarge the image
In addition, for measuring of CLA in rumen fluid, lipids in culture solutions were extracted using Folch’s solution (Folch et al. 1957) and samples obtained were analyzed with a gas chromatograph.
For blood urea nitrogen (BUN), blood samples (20 mL) were collected from the tail vein of cows at 20-days intervals using evacuated tubes containing ethylenediamine-tetraacetic acid (EDTA). The samples were kept in ice until centrifuged at 1000 × g for 20 min. Plasma was separated and stored frozen in plastic tubes at -20 ˚C until further analysis. The plasma urea concentration was determined using the method described by Khadem et al. (2009). Moreover, non-esterified fatty acid was analyzing according to Wako NEFA C kit no. 990-75401. (Wako, USA) as modified by Johnson and Peters (1993).
Statistical analyses
Statistical analysis was performed as repeated measures data using the MIXED PROC model of SAS software (SAS, 2004). The model included the fixed effects of treatment, PS and their interaction. Cow and period were considering as random effects.
The covariance structure used to best fit the model was selecting based on the Akaike’s Information Criterion of the mixed models of SAS (Littell et al. 1998). The GLM PROC was used when it was necessary. Results have presented as least square means and statistical differences were considering significant at (P<0.05).
RESULTS AND DISCUSSION
Body weight change, body condition score, dry matter intake and milk yield
The body weight changes (BWC) and body condition score (BCS) of animal have shown in Table 2. The body weight of all the cows were lowered during the experimental period.
There were significant differences for BWC of cows among experimental diets. The daily rate of body weight loss was higher in AH and lower in diet with alfalfa silage + fat (ASF) group cows and the differences were significant (P<0.05). Similarly final BCS of the cows was affected by the diets. Silage form of the alfalfa resulted to higher BCS of animals compared with the hay (P<0.05).
Table 2 Body weight chang (BWC), dry matter intake (DMI), milk yield and composition of lactating Holstein cows fed from experimental diets with varyinginclusion rates of chopped alfalfa hay and alfalfa silage
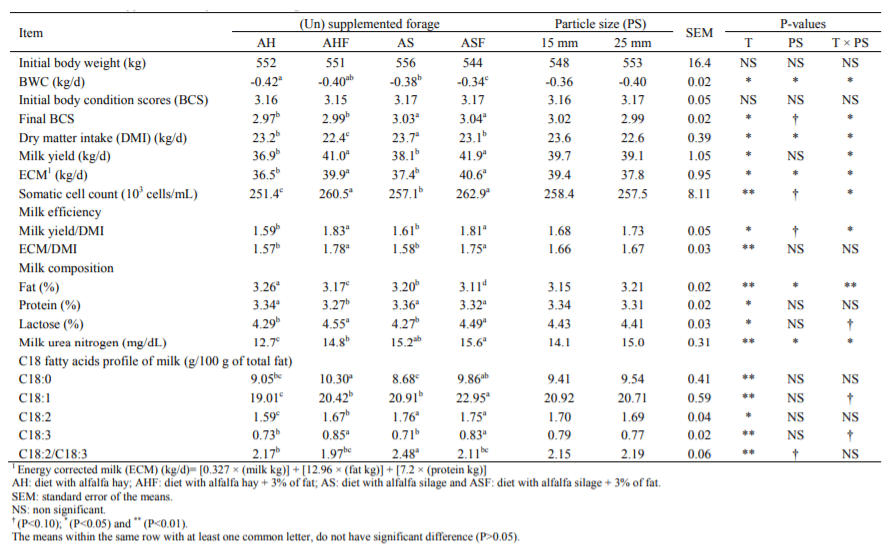

Click here to enlarge the image
Particle size also affected BWC and loss was higher (P<0.05) with 25 mm particle size diets as compared to 15 mm size diet. Significant differences (P<0.05) were observed in dry matter intake (DMI), milk yield and milk efficiency of cows in different treatments. DMI of cows fed alfalfa hay plus fat was lowest and that of alfalfa silage plus fat was highest (Table 2).
Fat supplementation in diet (i.e. ASF and AHF) resulted higher milk yield, ECM and milk efficiency (P<0.05). Using finer chopped alfalfa (15 mm vs. 25 mm) increased DMI and ECM (P<0.05), tended to decrease milk efficiency (MY/DMI) (P<0.10) and did not affect milk yield and milk efficiency (ECM/DMI).
Milk composition was affected by dietary treatments (P<0.05). Particle size reduction of alfalfa decreased milk fat percentage and MUN (P<0.05). Fat supplementation to the ration also decreased milk fat percentage in both hay (3.17 vs. 3.26) and silage (3.11 vs. 3.20) form of alfalfa (P<0.05). Somatic cell count significantly (P<0.05) de-creased when 3% fat was added to the diets (P<0.01). Con-tents of C18:1 and C18:2 fatty acids were changed when 3% fat was included in diets. Proportion of long chain fatty acids (C18:2 and 18:3) in milk fat was decreased (P<0.001), when fat was added to the rations. Chopping of forage had no effect on milk fat composition.
Blood metabolites and feeding behaviour
Feeding behaviours of experimental animals have presented in Table 3. Fat addition to the ration had no effect on eating time, but long particle size of roughage increased eating time (P<0.01).
Conversely, there were significant differences among treatments in the ruminating time (P<0.01). Cows fed ASF had the highest ruminating (488 min/d) and total chewing (939 min/d) time.
The highest resting time was observed in cows of AH and diet with alfalfa hay + fat (AHF) group. Higher BUN and blood glucose was observed in cows fed alfalfa silage and alfalfa silage plus fat and the differences were significant (P<0.05).
Fine chopped alfalfa (5.38 vs. 5.11 mmol/L) improved blood glucose levels (P<0.05). Fat addition to the diets, resulted to higher (P<0.05) non-esterified fatty acids and the differences were significant (P<0.05). Fine chopped alfalfa did not affect BUN and non-esterified fatty acids, but decreased eating, ruminating and total chewing time (P<0.01).
Cows spent less eating and ruminating time for finer chopped alfalfa (117.7 and 123.6 min/kg of alfalfa neutral detergent fiber (NDF) for short forage vs. 126 and 133.3 min/kg of alfalfa NDF for long forage) respectively.
Table 3 Blood metabolite and feeding behavior of lactating Holstein cows fed from experimental diets with varying inclusion rates of chopped alfalfahay and alfalfa silage
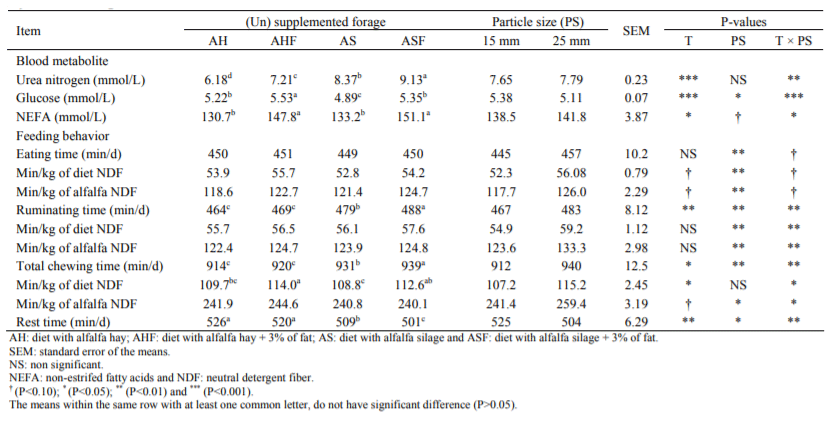

Click here to enlarge the image
Rumen fermentation
The average ruminal pH at 3 h after feeding ranged from 6.06 to 6.25 among the experimental treatments and the differences were significant (P<0.05) (Table 4). Ruminal VFA concentration was decreased by addition of fat to the diets (P<0.001). Alfalfa hay consuming cows had higher acetate, lower propionate and butyrate and higher acetate-to-propionate ratio in their rumen than silage consuming groups (P<0.05). Moreover, fat supplementation of the rations increased ruminal concentration of all C18 fatty acids (i.e. C18:0, C18:1, C18:2 and C18:3) (Table 2). Finer chopped alfalfa resulted to lower ruminal pH, acetate-to-propionate ratio and higher propionate and total VFA.
Similar to the findings of no effect of fat supplementation on BCS. Schroeder et al. (2002), Bell et al. (2006) and He et al. (2012)foundno significant differences in BCS ofcows fed fat supplemented diet. But, fat supplementation resulted to lower BWC in AHF and ASF because of higher energy density. Preservation method of forage (hay vs. silage) was effective on BWC and final BCS of animals. Moreover, finer chopped forage tended to decrease BWC and increase BCS. Higher DMI in both silage and finer chopped alfalfa consuming cows may be a reason for that. This finding is in accordance to the works of Kononoff and Heinrichs (2003) and Teimouri et al. (2004) who found that particle size reduction significantly increased BCS and im-proved BWC based on increase of feed intake. In contrast, Plaizier (2004) indicated that particle size of alfalfa has no effect on BW and BCS. Results for the DMI in this study are similar with those reported by Jerred et al. (1990), Vagnoni and Broderick (1997) and Calberry et al. (2003).
They found that the cows fed alfalfa silage (AS), had higher DMI than cows fed AH. Other researchers did not find relationship between forage preservation form (i.e. silage and haylage) and DMI or milk yield due to relatively low consumption of stored forage form (Onetti et al. 2004; Plaizier, 2004; Khadem et al. 2009). Addition of fat to the diets resulted in a decreased DMI and increased milk yield. These results were consistent with the reports of Schroeder et al. (2002),Onetti et al. (2004),Hollmann and Beede(2012) and Boerman and Lock (2014). While, Bell et al. (2006) and He et al. (2012) showed that fat supplementation has no effect on DMI and milk yield. It seems that sup-pressed DMI because of additional fat had no negative effect on milk yield, BCS or performance of cow in the early lactation period. Normally, DMI reduction can be a result of more energy intake in fat supplemented groups. In addition, DMI and ECM during early lactation were increased by alfalfa particle size reduction.
Several experiments have shown that feeding cows with high fat diets leads to a low milk fat. In contrary, He et al. (2012) demonstrated that fat supplementation of diet has no effect on milk fat. Milk fat depression is associated with changes in the pattern of ruminal biohydrogenation of unsaturated fatty acids that leads to accumulation of some fatty acids (trans-10 18:1 and trans-10 cis-12 18:2) in the rumen and inhibition of milk fat synthesis in the mammary gland (Griinari, 2003). The presence of unsaturated fatty acid as substrate, and an altered rumen environment appear to be necessary conditions for milk fat depression. In the present study, we didn’t find differences in the ruminal acetate concentration as the main precursor of milk fat among the fat supplemented and unsupplemented groups; but C18 fatty acids profile dramatically altered and C18:1, C18:2 and C18:3 concentration increased (P<0.05). It may be due to high amounts of these fatty acids in the supplemental fat and escape the rumen fermentation by ruminal passage rate. Reduction in forage particle size significantly decreased milk fat in the present study (P<0.05). It was similar with the reports of Onetti et al. (2004) and Teimouri et al. (2004). Lower rumination time and consequently lower rumen pH may alter rumen environment and biohydrogenation of C18 fatty acids that finally lead to decreased milk fat percentage. Experimental treatments showed significant differences in milk fat, protein and lactose contents (P<0.05). The data demonstrated that silage form of alfalfa in the ration decreased average milk fat (3.22 vs. 3.16% for hay and silage consuming cows, respectively). Higher rumen pH and more acetate as the main precursor of milk fat synthesis may be reasons for higher fat in these groups. There were a significant differences in the milk protein among treatments (P<0.05). We observed a significant reduction in the milk protein in AHF and an insignificant reduction in ASF consuming cows. It is suggested that additional dietary fat through suppressed microbial attachment to the fibre in AHF and decreased fermentation, limit microbial protein synthesis that could decrease milk protein (He et al. 2012; Hollmann and Beede 2012).
Finer particle size of roughage (hay or silage), resulted to a shorter eating time of the cows (445 vs. 457 min, P<0.01). Similarly, Beauchemin et al. (2008) and Khadem et al. (2009) found no differences in the eating time between AH and AS. Result for the ruminating behaviour in this study was similar with those reported by Khadem et al. (2009) who reported that ruminating time for cows fed AS was higher than AH. Higher ruminating time for AS compared to AH reflect higher DMI rather than a direct effect due to alfalfa preservation because this difference was not evident when ruminating time was expressed per unit of feed intake. Fat supplementation, was changed ruminating and chewing time especially when expressed as minute per kg of diet NDF (P<0.05). Higher rumination time in the fat supplemented groups may be a result of lower fiber digestion, probably due to limited microbial attachment to the fiber. However, Jerred et al. (1990) didn’t report any effect of supplemented fat on eating, ruminating and chewing time of dairy cows. Contrary our results Harvatine and Al-len (2005) demonstrated that fat supplementation of diet has negative effect on ruminating and total chewing time. Time spent chewing is primarily related to dietary intake and concentration of fiber and forage fiber and is poorly related to DMI. Total chewing time and time spent ruminating per kilogram of NDF intake wasn’t changing by fat supplementation (Table 3).
Therefore, differences in chewing behaviour observed in the current experiment could be attributed to differences in fiber intake. Treatment diets also contained the same base ration and are not expected to differ in effectiveness of stimulating rumination, although associative effects on ruminal fiber digestion and passage could have affected ruminal digesta pool size, leading to changes in rumination. Moreover, fat supplementation and chop length increased blood glucose concentration. Considering the fact that ruminal propionate concentration was insignificant in AH vs. AHF or AS vs. ASF (Table 4), then higher blood glucose concentration in AHF and ASF consuming cows (5.53 and 5.35 mmol/L for AHF and ASF vs. 5.22 and 4.89 mmol/L for AH and AS, respectively) cannot be due to propionate concentration for gluconeogenesis (P<0.05). This increase in the blood glucose level could be explained by shift of starch digestion from the rumen to the intestine (Larsen etal. 2009). Higher BUN in the silage consuming cows wasattributing to the higher rumen degradability of crude protein in AS fed cows (Table 4). It can lead to an increased ammonia production in the rumen and consequently increased urea production in the liver. However, Bhandari etal. (2008)showedthat preservation method of alfalfa hadno effect on BUN and blood glucose. In addition, Jerred etal. (1990)andSchroeder et al. (2002)illustratedthat fatsupplementation had no effect on BUN, non-esterified fatty acids and blood glucose.
Reducing alfalfa particle size decreased eating, ruminating and total chewing time. According to Beauchemin et al. (2008), these results were in the normal range. These results were similar to the reports of Kononoff and Heinrichs (2003), Teimouri et al. (2004), Onetti et al. (2004) and Bhandari et al. (2008), but were in contrast to Yang and Beauchemin (2007) who found no differences in eating time. Also, Bhandari et al. (2008) reported that BUN and blood glucose didn’t affected by chopping of alfalfa. So did Kononoff and Heinrichs (2003) found that forage particle size had no effect on non-esterified fatty acids concentration. Fat supplementation resulted to higher rumen pH. Maybe, the lower intact fat adversely affect fermentation and as a result pH is higher in these animals. Lower total VFA in these groups approves this suggestion. However, Jerred et al. (1990), Schroeder et al. (2002) and Onetti et al. (2004) reported that fat supplementation had no effect on ruminal pH, VFA, NH3-N, acetate and propionate in early lactation cows. Plaizier (2004), Beauchemin et al. (2008) and Khadem et al. (2009) reported that ruminal NH3-N was increased by AS vs. AH in dairy cow diets. We found similar results too. Higher ruminal NH3-N may be due to higher fermentation rate in the rumen, but probably lack of synchronization between carbohydrate and NH3-N resulted to high BUN and MUN.
Table 4 Rumen fermentation parameters of lactating Holstein cows fed from experimental diets with varying inclusion rates of chopped alfalfa hay andalfalfa silage
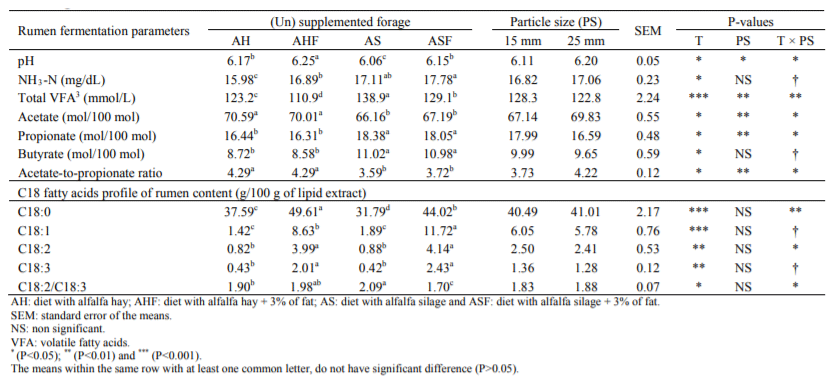

Click here to enlarge the image
Also similar to our results, Onetti et al. (2004) and Khadem et al. (2009) reported an increased concentration of VFA when AH was replacing with AS. This increase may be a result of higher feed consumption, especial fermentation of silage and increased amounts of propionate and butyrate due to higher passage rate. But, unlike AS and ASF, AH and AHF were increased rumen pH due to increased ruminating time. Based on the rumination time, it was expecting that ruminal pH be higher for AH and AHF consuming cows, because of higher saliva secretion and more buffering capacity in these groups. However, Plaizier (2004) and Yang and Beauchemin (2007) suggested that the concentrations of VFA, acetate and propionate were similar when AH and AS were using in diet.
Finer chopped forage resulted to lower ruminal pH (6.11 vs. 6.2, P<0.05). Shorter rumination and total chewing timeas a result of particle size reduction and consequent less buffering may be the main reason for lower pH in these groups. Onetti et al. (2004), Teimouri et al. (2004) and Yang and Beauchemin (2007) reported decreased rumen pH due to reduction in forage PS as well. In agreement with our result Onetti et al. (2004), Teimouri et al. (2004), Yang and Beauchemin (2007) and Bhandari et al. (2008) reported that VFA and propionate concentrations were greater in chopped alfalfa compared to long alfalfa. Reducing PS may increase the rate of rumen digestion because of increased attachment sites for microorganisms and higher DMI that may lead to more production of VFA.
ASF and AHF, respectively, increased polyunsaturated fatty acids concentrations in milk. These changes reflected changes in fatty acids composition of the diets and in DMI of cows.
Despite the reduction in DMI due to fat supplementation, intake of total C18 fatty acids tended to be higher for cows fed AHF and ASF. This result is consistent with those reported by several authors using fat supplementation (Bell etal. 2006; Onetti et al. 2004; Hollmann and Beede, 2012; Heet al. 2012; Boerman and Lock, 2014). Milk fatty acidsprofile, especial C18, depends on the balance between the increase in exogenous fatty acids (i.e. fat supplementation) transfer to the mammary gland by increased delivery and uptake of long-chain fatty acids in blood and the decrease in de novo synthesis. A reduction of fatty acids synthesis within the mammary gland is often observed when supplemental fat is added to the diet of lactating dairy cows (Jenkins et al. 2008; He et al. 2012). Ruminal biohydrogenation of dietary unsaturated fatty acids is relatively constant (86 and 82%, respectively, for C18:2 and C18:3), whereas secretion of these in milk is more variable (Jenkins et al. 2008). High supply of these fatty acids and escapefrom the rumen was the main reason for higher amount of these fats in milk, nevertheless obvious changes were happening in the profile of C18 fatty acids in the rumen and milk and it was very different from the dietary fatty acid profile (Table 2). In agreement with our result Onetti et al. (2004) and He et al. (2012) reported that fat supplementation had significantly effective on C18:0 and C18:1. But, Onetti et al. (2004) stated that fat supplementation wasn’t affected on C18:2 and C18:3.
CONCLUSION
It is concluded that fat supplementation significantly increased the proportion of C18:0, C18:1, C18:2 and C18:3 fatty acids in milk. Although, milk fat of cows decreased when fat was fed at 3% of diet dry matter. Replacement of AH with AS in diets containing 3% fat increased somatic cell count and MUN in milk, ruminating and total chewing time, ruminal NH3-N, VFA, propionate and butyrate in rumen, CLA in rumen and milk. The results suggest that ASF and AHF are more effective in sustaining DMI, milk yield, C18:1 and C18:2, VFA, propionate, chewing activity and BUN than AS and AH that was fed, respectively. Thus, C18-enriched milk produced as a result of feeding fat could increase the range of milk and milk products available to consumers. Also, reduction of chop length increased final BCS, DMI, ECM, blood glucose, total VFA and propionate in rumen, but decreased acetate, acetate-to-propionate ratio, rumen pH, total chewing activity, rumination time, and milk fat.
ACKNOWLEDGEMENT
We gratefully thank the team at the experimental dairy farm at the Cooperative Company Shaghayegh-Dam of Tehran for financial support and University of Tabriz and Razi Serum and Vaccine Research Institutes for their technical assistance.
This article was originally published in Iranian Journal of Applied Animal Science (2016) 6(2), 293-301.