As a result of consumer concern about food safety, the association between animal and human health has become a prominent issue. A recent study published by Ramchandani and coworkers (2005) found that many of the Escherichia coli strains responsible for urinary tract infections in women can be traced back to E. coli strains from the intestinal tract of cattle. Even more significantly many of these organisms were found to be resistant to a variety of antibiotics, which often results in increased treatment cost and rehabilitation time. Until recently, researchers were only interested in the development of antibiotic resistance one antibiotic at a time. Today we know that it is much more common for antibiotic resistant bacteria to be resistant to two or more compounds. The discovery of gene cassettes or intregons that contain the genetic information for resistance to several different antibiotics or toxic compounds in both Gram(-) and Gram(+) bacteria has emphasized the need to re-evaluate our current understanding of the persistence of antibiotic resistance in a bacterial population.
Bacteria with high levels of resistance have been isolated from environments contaminated with antimicrobial agents such as hospitals, aquaculture, sewage effluents and wastewater (Fontain and Hoadley, 1976; Gauthier and Breittmayer, 1990; Mach and Grimes, 1982; Sandaa et al., 1992). However, resistant bacteria have also been isolated from theoretically nonselective (water and soil) environments (Levy, 1992; Memish et al., 2003). Resistant genes reside not only in disease-causing organisms (Salmonella spp., Campylobacter spp., Pasteurella and Actinobacillus spp.), but also in commensal organisms (E. coli, enterococci and Bacteroides spp.). The incidence of resistance varies depending on the environment (Levy, 1997; McEwen and Fedorka-Cray, 2002). The most frequently proposed hypothesis is that genes involved in antibiotic resistance originated in antibiotic-producing organisms and acted to prevent self-destruction in these organisms (Davies, 1992). Table 1 illustrates the mode of action of the antibiotic against the bacterial cell and the potential response of a resistant organism.
Streptomyces species produce aminoglycoside antibiotics. In 1973, a study demonstrated that Streptomyces species contain aminoglycoside-modified enzymes that allow this species to overcome the effects of the antibiotic (Benveniste and Davies, 1973). During the process of purification from these antibiotic-producing organisms, remnants of the DNA containing antibiotic-resistant genes are also co-extracted and can be recovered in the antibiotic preparation. During antibiotic administration, antibiotic resistance genes could then be co-administered and, therefore, reach bacteria in the environment where they become incorporated into the bacterial population (Webb and Davies, 1993; Woo et al., 2003).
Table 1.Mode of action of several different antibiotic types and the bacterial resistance mechanism associated with each class of antibiotic.
Human health and animal productionIt is generally accepted that there is a link between the use of antibiotics and the selection for resistance (Baquero et al., 1998; Woo et al., 2003). Treatment with a single drug usually leads to resistance to that drug; however cross-resistance to other antibiotics (multidrug-resistance) that are structurally and functionally unrelated may also occur (Lewis, 1994; Poole, 1994). Soon after the use of penicillin, S. aureus was found to produce penicillinase. To overcome this situation, the antibiotic methicillin was used to replace penicillin and a S. aureus resistant to methicillin emerged very quickly. This pattern was also seen following the use of vancomycin (Woo et al., 2003). However, the abundance of antibiotic-resistant bacteria in the natural environment where the bacteria have not been exposed to antibiotics may suggest that antibiotic-resistant genes can be kept stable in the absence of antibiotic pressure (Salyers and Amábile-Cuevas, 1997).
A zoonosis is an infectious disease transmissible from vertebrate animals to man (Acha and Szyfres, 1994). In industrialized countries, the zoonoses that are most frequently seen are food-borne infections caused by species of Campylobacter, enterohemorragic E. coli, Listeria, Salmonella and Yersinia. The gastrointestinal tract of livestock can be a primary reservoir of zoonotic agents. Many food-borne infections result from fecal contamination during slaughter or cross-contamination with fecal material during further processing (Aarestrup and Wegener, 1999).
Development of antibiotic resistance in zoonotic bacteria constitutes a public health risk due to the possibility of treatment failures and the propagation of antimicrobial resistance in humans (Aarestrup and Wegener, 1999). The potential human-health consequences have been demonstrated by outbreaks of quinolone-resistant S. typhimurium DT104 and fluoroquinolone-resistant C. jejuni that resulted in treatment failures in patients (Endtz et al., 1991; Molbak et al., 1999). Another outbreak involved tetracycline resistant S. newport in which 18 cases of salmonellosis were reported in the US midwest and directly linked to a beef herd that had received tetracycline in the feed (Holmberg et al., 1984).
The gastrointestinal tract of an animal is an excellent environment for the exchange of genetic information (Van den Bogaard, 1997). E. coli and Salmonella human isolates, resistant to Apramycin, provided additional evidence that resistant organisms can be transferred from animal to human since this particular antibiotic is not used for human treatment (Hunter et al., 1993; Wray et al., 1986). In Europe, fluoroquinolone-resistant strains of C. jejuni were isolated in humans following the use of enrofloxacin in poultry (Jacobs-Reitsma et al., 1994; Velazquez et al., 1995). A similar situation was observed with nourseothricin resistant E. coli, when this antibiotic was used as a growth promoter for swine but was not used in human medicine. Nourseothricin resistance was detected in the pigs, farm employees and their relatives, as well as in residents from the surrounding communities (Hummel et al., 1986). An epidemic of resistant S. heidelberg in Connecticut was traced to calves on a dairy farm (Lyons et al., 1980). Eighteen people in the US midwest became ill when infected with multiple-drugresistant S. newport traced to a beef herd in South Dakota (Holmberg et al., 1984). Separate outbreaks of S. typhimurium in Arizona and Canada were traced to multiple-drug-resistant bacteria in raw milk (Tacket et al., 1985; Bezanson et al., 1983). Finally, an outbreak of S. newport in 45 patients in California was linked to beef from dairy farms (Spika et al., 1987).
Medicated milk replacers have been implicated as a potential risk factor when describing the presence of multiple antibiotic resistant bacteria in dairy calves. Martel and Coudert (1993) suggested that the emergence of antibiotic resistant organisms was greater in young calves compared with older cattle, reasoning that drug use is greater with young calves because of greater susceptibility to bacterial diseases. In addition, the transfer of antibiotic resistance from cows receiving antibiotics to nursing calves has also been examined.
Development of antibiotic resistant microorganisms was investigated in calves fed milk from cows treated for mastitis (Wray et al., 1990). Calves that received milk from cows treated with antibiotics for mastitis developed bacteria that were resistant to streptomycin whereas calves in the group fed milk replacer without antibiotics did not shed antibiotic resistant organisms into the environment. Other studies have reported the existence of antibiotic resistant organisms in calves. Hariharan et al. (1989) isolated E. coli that was resistant to potentiated sulfonamide (a combination of trimethoprim and sulfamethoxazole) from fecal samples of calves with diarrhea. Also, all the E. coli isolates were resistant to tetracycline, and most were resistant to neomycin and ampicillin as well. A summary of studies from several countries indicates that multiple-drug resistant E. coli is widespread in calves with enteritis (NRC, 1999).
When assessing resistance risks from the use of antimicrobials in animals, it is important to consider other sources of resistance (McEwen and Fedorka-Cray, 2002). Fecal waste from animals is usually spread as fertilizer, composted or stored in lagoons. Antibiotic
Selection for antibiotic resistanceThe widespread development of resistance to several different antibiotics is generally a result of lateral or horizontal gene transfer. This is a process where DNA can be transferred between individual bacteria. Three mechanisms of horizontal gene transfer have been identified: transduction, transformation and conjugation. Transduction involves the ability of bacteriophage to transfer DNA between two closely related bacteria (e.g. between two different Pseudomonas spp.).
Transformation is the process where free DNA is taken into the bacteria cell from the environment. This process is dependent on the close association of the bacteria with the free DNA and their ability to first take the DNA into the cell and then incorporate the genetic information in their own DNA.
The final and arguably most important mechanism for horizontal gene transfer is conjugation. Conjugation is known to be the major mechanism by which Gram(-) bacteria exchange DNA (Woo et al., 2003). Conjugation requires cell-to-cell contact for transfer of mobilizable elements from donor to recipient cell (Dröge et al., 1998). Conjugation between Gram(+) and Gram(-) bacteria has been described, for example the intergeneric conjugation from E. coli to Streptomyces species and to coryneform bacteria (Mazodier et al., 1989; Schäfer et al., 1990). The primary mobile elements are plasmids and conjugative transposons, which are broad-host range conjugal elements. Plasmids are extrachromosomal DNA elements that replicate independently of the chromosome of the bacterial cell. Plasmids are generally small, circular, double stranded molecules that can be identified in a variety of bacterial types. The number and varieties of plasmids in a bacterial cell can vary. The genes contained on plasmids are considered to be non-essential but often offer some competitive advantage to the bacterium. Plasmids range in size from 1 Kb to 2000 Kb. Resistance plasmids or ‘R plasmids’ code for enzymes that can inactivate antibiotics, prevent the uptake of an antibiotic or pump out the particular antibiotic (Madigan et al., 2003). Antibiotic resistanceconferring plasmids have been described in virtually all bacteria and are widely dispersed in nature. In Gram (-) bacteria, this resistance frequently occurs with multiple antibiotics (Neu, 1989). Conjugation of plasmid transfer is common among Enterobacteriaceae, Pseudomonas and many anaerobic bacteria (Neu, 1992).
Gram(-) bacilli causing nosocomial infections are usually multiple-antibiotic resistant, resulting in significant problems for treatment. The mortality rate in these patients tends to be very high. The antibiotics most commonly associated with resistance are penicillins, first to third generation cephalosporins and aminoglycosides, particularly gentamicin. Recently a new class of mobile genetic elements called intregons has been described in many Gram(-) bacteria throughout the world. These intregons are horizontal gene transfer systems usually linked to transposons or conjugative plasmids, which allow them to be spread easily in the presence of antibiotic use.
Hugues and Datta (1983) determined that most of the plasmids studied today are similar to those found prior to the beginning of the antibiotic era, except these plasmids carried no resistance genes at that point. The F ‘fertility factor’ plasmid in E. coli was the first bacterial host system to be described (Willetts and Skurray, 1987). The F plasmids exist frequently as a single-copy plasmid in Enterobacteriaceae, which proliferate in the animal digestive tract (Waters, 1999). This ecosystem constitutes a stable environment and allows F plasmids to develop a transfer apparatus with higher genetic complexity, while promiscuous plasmids (IncN, P, W and Q) in more unstable environments may have to develop simpler, less specialized and less efficient but more versatile conjugative apparatus (Sastre et al., 1998).
CONJUGATION PROCESSConjugation is a process requiring cell-to-cell contact that involves the transmission of genetic material, including antibiotic-resistance genes (R plasmids), from one cell to another (Figure 1). Conjugation occurs at significantly higher frequency and efficiency than transformation, and several resistant genes can be obtained simultaneously at a rate of 10
-1 per organism (Waters, 1999). For the process, basic elements must be present: the donor bacteria containing the F factor (pili) and the recipient bacteria that will obtain the genetic material (R plasmid). Essential functions for conjugation include DNA preparation, mating-bridge formation and conjugative DNA processing (Daugelavicius et al., 1997; Haase et al., 1995).
Figure 1.Electron micrograph of two bacteria in the process of conjugation.
Among the earliest events in the conjugation process, contact between donor and recipient is an essential step. This process is usually mediated by adhesins on the Figure 1. Electron micrograph of two bacteria in the process of conjugation. surface of the microbe. Some adhesins are assembled into hair-like appendages called pili or fimbriae that extend from the bacterial surface (Figure 2). In other cases, non-pilus adhesins are also associated with the microbial cell surface. Based on their assembly and system pathway, adhesins can occur in a variety of ways (Soto and Hultgren, 1999). A prominent example of an adhesin associated with antibiotic resistance-carrying plasmids is called a Type I pili. These appendages are prototypic class I fimbriae and are important virulence determinants in E. coli and in most members of Enterobacteriaceae.
Type I pili mediate binding to the mannose-containing oligosaccharide receptors of the host cell (Sokurenko et al., 1992). The second step of conjugation includes the formation of a relaxosome, which is the conjugative DNA plasmid/ protein complex made by the binding of certain transfer proteins. The relaxosome is located at the base of the bridge. Relaxases, or nicking enzymes, will cleave specific strands of the DNA at the origin of transfer (oriT), resulting in a single strand of relaxed DNA (Waters, 1999). As a result of nicking, the 5′ end of the cleaved strand will be bound covalently to relaxase, forming the transferred intermediate (relaxosome) (Lawley et al., 2002). The relaxosome then moves towards the cytoplasmic side of the cell envelope to the transport pore (Gomis-Rüth et al., 2002). The Mating Pair Bridge is then formed (at the donor cell) and is responsible for the transfer of DNA from the donor to the recipient. The mating bridge is a multiprotein complex apparatus that spans the cytoplasmic membrane (Gram(+)) and outer membrane (Gram(-)), forming a pore or channel. Finally the transfer signal will open the bridge pore, which will allow the rapid transfer of a nicked and protein-bound single strand of DNA (Waters, 1999). In vivo signals, such as sugars, phenolic compounds and peptides (sex pheromones), released within the host milieu play an important role in bacterial gene transfer (Detmers et al., 2001; Maqueda et al., 1997; Mel and Mekalanos, 1996). The DNA transport apparatus, a type IV secretion system, enables conjugative plasmids to control and finally transfer DNA from a donor to a recipient cell, which then becomes a potential donor. ‘Two-way conduction’, or retrotransfer, occurs with low frequency but has been described (Heinemann and Ankebauer, 1993; Mergeay et al., 1987). The plasmid-containing donor can act as an active recipient directing the formation of a second conjugation bridge for the transfer of DNA from the transconjugant to the donor (Sia et al., 1996).
KINETICS OF PLASMID TRANSFERThe kinetics of horizontal DNA transfer can be used to describe the ecology and evolution of bacteria with the spread of antibiotic resistance (Andrup and Andersen, 1999). In order to quantitatively express the spread of plasmids, Andrup and coworkers (1998) used a mathematical model based on the similarity of bacterial conjugation to Michaelis and Menten enzyme kinetics. The process of conjugative transfer could be explained as a catalytic change of genetic information from recipients to transconjugants, mediated by the donors. In this case, donor and recipients are analogous to the enzyme and the substrate, respectively. The mating pair is comparable to the activated complex; and the transconjugant is equivalent to the product. Therefore, the conjugation system will be characterized by two constants. First, ‘Vmax’, which is related to the maximal conjugation rate or the number of transconjugants produced per donor per minute. The second constant will be ‘Km’, which relates to the recipient concentration where the conjugation rate is half its maximal value (Andrup and Andersen, 1999). The epidemic spread of plasmids through a bacterial population depends on the chief transfer from donor cells and the ability of recipients to become donors (Cullum et al., 1978).
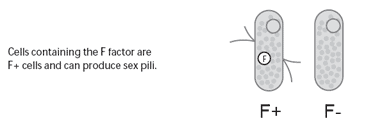
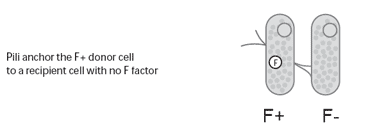
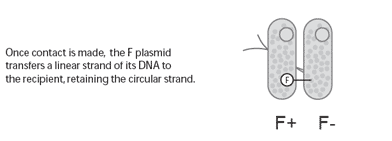
Figure 2.The conjugation process.Antibiotic resistance in the environmentMany studies have demonstrated that plasmid transfer between bacteria occurs in diverse environments. Most of the transfer is described using bacteria from the same group or ecological niche. It seems that there is no distinction between plasmids derived from bacteria of human, animal or fish origin (Kruse and Sørum, 1994). In vitro studies have demonstrated that R plasmids can be transferred from bacteria of animal or fish origin to humans and from human pathogen to fish pathogen (Hinton et al., 1986; Sandaa et al., 1992). Kruse and Sørum (1994) obtained frequencies of plasmid transfer for bacteria of different species and origins (human, bovine and marine) mimicking natural environments (untreated seawater, minced meat on a cutting board and feces from pigs) similar to frequencies measured under standard laboratory cultural conditions. Pukall and coworkers (1996) demonstrated that in a soil microcosm there were high frequencies of plasmid transfer from sewage and manure origin (including broad- and narrow-host range), mainly IncP plasmid between E. coli species. The presence of antibiotic resistant bacteria in different environments represents a real threat, not only as a source of possible diseases, but also for the potential spread of R plasmids to other pathogenic or non-pathogenic microorganisms of diverse origins (Kruse and Sørum, 1994).
‘CURING’ ANTIBIOTIC RESISTANCECuring is the process of removing plasmids from a bacterial cell. This may be observed with high copy number or relaxed plasmids when the bacterial cell is grown for successive generations in the absence of a selective agent (e.g., antibiotic). The resulting bacteria then become sensitive to the selective agent. Initially it was thought that this phenomenon would be an appropriate solution to control the development of antibiotic resistance in formerly antibiotic susceptible bacteria. Simply remove the offending antibiotic from the bacterium’s environment and it would ultimately revert to its previously antibiotic receptive form. Unfortunately we now know that many plasmids are conserved even in the absence of the selective agent. Many possible reasons for this conservation exist, including the presence of DNA cassettes that encode for stability (Zielenkiewicz and Ceglowsk, 2001). In the quest to find a solution to the problem of antibiotic resistance some researchers have searched for compounds that might retard the spread of these determinants or eliminate them from the bacteria. The objective would be to identify a safe substance that could be fed to livestock and poultry to eliminate resistance plasmids from intestinal bacterial or prevent the transfer of these plasmids among the bacteria. As a result, the prevalence or degree of resistance of the intestinal flora may be reduced.
Antibiotics such as mitomycin, rifampin, and flavophospholipol and DNA intercalating dyes such as acridine orange and ethidium bromide have been shown to cure many plasmids. As a result of this plasmid loss the bacterium will no longer have the genetic advantages contained on the plasmid. A natural DNA intercalating compound is plumbagin (Fuji et al., 1992). This compound is found in the Indian medicinal plant Plumbago zeylanica, which has been associated with antimicrobial, antimutagenic, antitumor and radiomodifying properties (Krishnaswami and Purushothoman, 1980). In a study evaluating the effect of this plant extract on multi-antibiotic resistant bacteria of clinical origin, 14% of the plasmid containing bacteria exhibited curing. This percentage of curing is equivalent to the more widely accepted curing agents acridine orange and ethidium bromide (36%), but pefloxacin was the most efficient agent (88%) (Beg and Ahmad, 2000). Many phenolic compounds can interfere with DNA replication and consequently result in the elimination of plasmids (Lakshmi et al., 1989). Pefloxacin is an antibacterial compound with similar structure to norfloxacin and ofloxacin (Ahmad et al., 1993). Other phenolic compounds, such as bharangin and gossypetin at concentrations > 200 ppm, showed similar curing results as the previous studies (Lakshmi et al., 1989).
Not all plasmids encode for antibiotic resistance. Some may contain the genetic determinants for resistance to other environmental stressors such as heavy metals and toxins or the plasmids might provide other survival advantages to the bacterium such as virulence, toxin production or the formation of compounds like pediocin. Pediocin is a bacteriocin produced by pediococci that inhibits the growth of many Gram(+) pathogenic and food spoilage bacteria (Bhunia et al., 1988). Problems occur when the pediococci produce this potent antimicrobial during fermentation. The resulting food product is often unacceptable because proper fermentation is dependent on the growth of healthy starter cultures. In the presence of pediocin the starter cultures are generally killed or inhibited. As a result, the food industry has investigated several ways to cure the pediocin encoded plasmids from these organisms. Among these agents novobiocin, ethidium bromide, acriflavin orange, acridine orange, ascorbic acid and elevated growth temperatures have been effective (Gonzalez and Kunka, 1987; Ramesh et al., 2000). Ascorbic acid (vitamin C) is a non-hazardous curing agent that is readily available resulting in approximately 35% curing rate in many strains of pediococci (Ramesh et al., 2000).
OPTIONS FOR THE FUTUREIt is generally accepted that the use of antibiotics not only leads to the emergence of antibiotic resistance but also perpetuates the dissemination of antibiotic resistance among pathogenic and commensal bacteria (potentially pathogenic bacteria) (Van den Bogaard and Stobberingh, 2000). The problem behind the increase in resistant populations are the ‘easy to get’ and ‘hard to lose’ resistance genes (Salyers and Amábile-Cuevas, 1997). Some plasmids are very stable and can be maintained through consecutive generations (Trevors, 1986). It has been reported that certain physical treatments and chemical compounds as well as growth conditions may increase the frequency of elimination of drug-resistant R plasmids, resulting in sensitive cells that were previously resistant to antibiotics (Lakshmi et al., 1989). DNA intercalating dyes, sodium dodecyl sulfate (SDS), antibiotics, thymine starvation and elevated temperatures have been used as curing systems, having a very successful effect on sensitization of previously resistant bacteria (Chakrabartty et al., 1984; Gupta et al., 1980; Obaseiki-Ebor, 1984; Reddy et al., 1986). Curing refers to the ability of bacteria to spontaneously lose their resistance plasmid under the effect of certain compounds, thus reverting to antibiotic sensitive bacteria (Trevors, 1986). It has been suggested that the variety of curing agents may have several different modes of action; they may affect membrane potential, membrane permeability, protein synthesis and the processing of DNA, eliminating certain types of plasmids (Stanisich, 1984; Viljanen and Boratynski, 1991).
The most effectiveconcentrations of a particular curing agent depend upon the species being treated, efficiency of the curing agent and the mode of action of that particular compound (Carlton and Brown, 1981). Acridine orange has shown to cure F plasmids from E. coli, and it is suggested that this dye interferes with plasmid replication, stimulating the entire plasmid loss (Hohn and Korn, 1969; Salisbury et al., 1972). Using combinations like ethidium bromide and novobiocin or imipramine and methylene blue resulted in curing six plasmids from Salmonella of avian origin of the 17 strains evaluated (Poppe and Gyles, 1988). Contrary to these results, studies with Lactobacillus plantarum showed that intercalating agents, such as acriflavine and ethidium bromide, were not as effective as novobiocin in curing plasmids (Ruiz- Barba et al., 1991). Elimination of plasmids with chemical agents is not always a success. In fact, intercalating dyes do not cure large plasmids (> 250 mDa) (Stanisich, 1984).
The mode of action of many of these curing agents is not fully understood. In general the agents tend to be toxic to the host bacteria. The concentrations at which curing occurs is just slightly lower than the concentration that might inhibit or kill the host organism. Comermycin, novobiocin or rifampicin act as curing agents by inhibition of DNA gyrase or RNA polymerease. Their action inhibits DNA plasmid replication and consequently promotes the very effective degradation or elimination of antibiotic resistant plasmids (Danilevskaya, 1980; Trevors, 1986). Sodium dodecyl sulfate is an ionic detergent found in household products (shampoos) and is widely used as a protein-denaturing compound in electrophoresis systems. Also, it is capable of curing R and F plasmids in E. coli (Tomoeda et al., 1968). Some curing compounds block plasmid transfer. Epigallocatechin gallate (EGCg) is a tea catechin that is suggested to act by blocking or significantly reducing the transfer of conjugative R plasmids between E. coli species. The inhibition rates of transfer were dose dependent, signifying a 42–67% inhibition at 50–200 μg/ml and up to 99% at 800 μg/ml (Zhao et al., 2001).
Promethazine is a neuroleptic, antibacterial and antiplasmid agent and has a heterocyclic planar ring that binds DNA between bases, resulting in inhibition of plasmid replication and the DNA partitioning process. It was shown that this compound had a better curing effect in a multi-species community or in densely populated normal flora, such as GI tract, skin, etc (Molnár et al., 2003). A natural alternative to many of the potentially toxic curing compounds described in the literature might be extracted yeast cell wall. Studies recently performed at the University of Kentucky have shown that the prevalence in a growing population of streptomycin- and ampicillin-resistant strains of Salmonella is reduced in the presence of some yeast cell wall preparations (Figures 3 and 4). Strategies using these types of materials for decreasing the prevalence of antibiotic resistant bacteria are attractive since the use materials that can be safely introduced into an animals diet without the concerns associated with most of the chemical curing agents.
Even elevated temperatures (43–44°C) have been successfully used not only to cure tetracycline-resistant and penicillinase-positive strains of S. aureus but also for curing F plasmids from E. coli (May et al., 1964; Stadler and Adelberg, 1972).
Changes in bacteria characteristics and structure have been reported as a result of plasmid acquisition or plasmid elimination (curing). Acquisition of drug resistant plasmids by S. enteritidis resulted in a phage type change (Frost et al., 1989). A change in lipopolysaccharide composition and shorter O-specific side chains were seen in S. dublin cured of its virulence plasmid. Cured Salmonella isolates (S. enteritidis and S. havana) demonstrating missing plasmids also exhibit a loss of long chain lipopolysaccharides (becoming rough), as well as variations in outer membrane proteins (Icgen et al., 2001). Curing plasmid-containing Streptomyces that can impart off-flavors to drinking water resulted in the loss of a linear plasmid DNA and leaving a bald mutant (no aerial mycelia) (Saadoun et al., 1998).
ConclusionsAarestrup, F.M. and H.C. Wegener. 1999. The effect of antibiotic usage in food animals on the development of antimicrobial resistance of importance for human in Campylobacter and Escherichia coli. Microbes Inf. 1:639-644.
Acha, P.N. and B. Szyfres. 1994. Zoonoses and Communicable Diseases Common to Man and Animals. Pan American Health Organization, Pan American Sanitary Bureau, Washington, DC, USA.
Ahmad, I.J., N.S. Yadava and S. Ahmad. 1993. Elimination of R factors among Escherichia coli strains with special reference to norfloxacin. Indian Vet. Med. J. 7:115-122.
Andrup, L., L. Smidt, L. Andersen and L. Boe. 1998. Kinetics of conjugative transfer: a study of the plasmid pXO16 from Bacillus thuringiensis subsp. israelensis. Plasmid 40:30-43.
Andrup, L. and K. Andersen. 1999. A comparison of kinetics of plasmid transfer in the conjugation systems encoded by the F plasmid from Escherichia coli and plasmid pCF10 from Enterococcus faecalis. Microbiol. 145:2001-2009.
Baquero, F., M.C. Negri, M.I. Morosini and J. Blazquez. 1998. Antibiotic-selective environments. Clin. Dis. 1998; 27(Suppl. 1): S5-S11
Beg, A.Z. and I. Ahmad. 2000. Effect of Plumbago zeylanica extract and certain curing agents on multidrug resistant bacteria of clinical origin. World J. Microbiol. Biotech. 16:841-844.
Benveniste, R. and J. Davies. 1973. Aminoglycosides: antibiotic-inactivating enzymes in actinomycetes similar to those present in clinical isolates and antibiotic-resistant bacteria. Proc. Nat. Acad. Science USA 70:2276-2280.
Bezanson, G.S., R. Khakhria and E. Bollegraaf. 1983. Nosocomial outbreak caused by antibiotic-resistant strain of Salmonella typhimurium acquired from dairy cattle. Can. Med. Assoc. J. 128:426-427.
Bhunia, A.K., M.C. Johnson and B. Ray. 1988. Purification, characterization and antimicrobial spectrum of a bacteriocin produced by Pediococcus acidilacti. J. Appl. Bacteriol. 65:261-268.
Carlton, B.C. and B.J. Brown. 1981. Gene mutation. In: Manual Methods of General Bacteriology (P. Gerhardt, R.G.E. Murray, R.N. Costilow, E.W. Nester, W.A. Wood, N.R. Kreig. and G.B. Philip, eds). American Society of Microbiology, Washington, DC, USA, pp. 222-242.
Chakrabartty, P.K., A.K. Mishra and S.K. Chakrabarti. 1984. Loss of plasmid-linked drug resistance after treatment with iodo deoxy uridine. Indian J. Expt. Biol. 22:333-334.
Chee-Sanford, J.C., R.I. Aminov, I.J. Krapac, N. Garrigues Jeanjean and R.I. Mackie. 2001. Occurrence and diversity of tetracycline resistance genes in lagoons and groundwater underlying two swine production facilities. App. Environ. Microbio. 67(4):1494-1502.
Cullum, J.J., F. Collins and P. Broda. 1978. Factors affecting the kinetics of progeny formation with F’lac in Escherichia coli K12. Plasmid. 1:536-544.
Danilevskaya, O.N. and A.I. Gragerov. 1980. Curing of Escherichia coli K12 plasmids by coumermycin. Mol. General Genetic 178:133-235.
Daugelavicius, R., J.K.A. Bamford, A.M. Grahn, E. Lamnka and D.H. Bamford. 1997. The IncP plasmidencoded cell envelope-associated DNA transfer complex increases cell permeability. J. Bacteriol. 179(16):5195-5202.
Davies, J. 1992. Another look at antibiotic resistance. J. Gen. Microbiol. 138:1553-1559.
Detmers, F.J.M., F.C. Lanfermeijer and B. Poolman. 2001. Peptides and ATP binding cassette peptide transporters. Res. Microbiol. 152:245-258.
Dröge, M., A. Pühler and W. Selbitschka. 1998. Horizontal gene transfer as a biosafety issue: A natural phenomenon of public concern. J. Biotechnol. 64:75- 90.
Endtz, H.P., J. Rujisg, B. Van Klingenen, W.H. Jansen, T. Vander Reyden and R.P. Mouton. 1991. Quinolone resistance in campylobacter isolated from man and poultry following the introduction of fluoroquinolones in veterinary medicine. J. Anti. Chemo. 27:199-208.
Fontaine, T.D.III and A.W. Hoadley. 1976. Transferable drug resistance associated with coliforms isolated from hospital and domestic sewage. Health Lab. Sci. 13:238-245.
Frost, J.A., L.R. Ward and B. Rowe. 1989. Acquisition of drug resistance plasmid converts Salmonella enteritidis phage type 4 to phage type 24. Epid. Infect. 103:243-248.
Fuji, N., Y. Yamashita, M. Nagashima and H. Nakano. 1992. Induction of topoisomerase II mediated DNA cleavage by the plant naphthoquinones plumbagin and shikonin. Anti. Agents Chemo. 36:2589-2594.
Gauthier, M.J. and V.A. Breittmayer. 1990. Gene transfer in marine environment. In: Bacterial Genetics in Natural Environments (J.C. Fry and M.J. Day, eds). Chapman and Hall, London, pp. 100-110.
German, B., E.J. Schiffrin, R. Reniero, B. Mollet, A. Pfeifer and J.R. Neeser. 1999. The development of functional food: lessons from the gut. Trends Biotech. 17:492-499.
Gomis-Rüth, F.X. and M. Coll. 2001. Structure of TrwB, a gatekeeper in bacterial conjugation. Intl. J. Biochem. Cell Bio. 33:839-843.
Gonzalez, C.F. and B.S. Kunka. 1987. Plasmid associated bacteriocin production and sucrose fermentation in Pediococcus acidilactici. Appl. Environ. Microbiol. 53:337-341.
Gupta, T.D., T. Bandyopathyay, S.G. Dastidar, M. Bandopadhyay, A. Mitra and A.N. Chakrabarty. 1980. R plasmids of Staphylococcus and their elimination by different agents. Indian J. Exp. Bio. 18:478-481.
Haase, J., R. Lurz, A. M. Grahn, D.H. Bamford and E. Lanka. 1995. Bacterial conjugation mediated by plasmid RP4:RSF1010 mobilization, donor-specific phage propagation and pilus production require the same Tra2 core components of proposed DNA transport complex. J. Bacteriol. 177(16):4779-4791.
Hariharan, H., J.W. Bryenton, J. St-Onge, J.R. Long and M.O. Ojo. 1989. Resistance to trimethoprimsulfamethoxazole of Escherichia coli isolated from pigs and calves with diarrhea. Can. Vet. J. 30 (4):348- 349.
Heinemann, J.A. and R.G. Ankebauer. 1993. Retrotransfer in Escherichia coli conjugation: bidirectional exchange or the novo mating? J. Bacteriol. 175:583-588.
Hinton, M., A. Kaukas and H. Linton. 1986. The ecology of drug resistance in enteric bacteria. J. Appl. Bacteriol. 61:77-92.
Hohn, B. and D. Korn. 1969. Cosegregation of a sex factor with the Escherichia coli chromosome during curing by acridine orange. J. Mol. Bio. 45:385-395.
Holmberg, S.D., J.R. Wells and M.L. Cohen. 1984. Animal-to-man transmission of antimicrobial resistant Salmonella: investigation of U.S. outbreaks 1971- 1983. Science 225:833.
Hughes, V. M. and N. Datta. 1983. Conjugative plasmids in bacteria in the “pre-antibiotic” era. Nature 302:725- 726.
Hummel, R., H. Tschape and W. Witte. 1986. Spread of plasmid-mediated nourseothricin resistance due to antibiotic use in animal husbandry. J. Basic Microbiol. 26:461-466.
Hunter, J.E., C.A. Hart, J.C. Shelley, J.R. Walton and M. Bennett. 1993. Human isolates of apramysin resistant Escherichia coli which contain the genes for the AAC (3) IV enzyme. Epi. Inf. 110:253-259
Içgen, B., G.C. Gürakan and G. Özcengiz. 2001. Effect of plasmid curing on antibiotic susceptibility, phage type, lipopolysaccharide and outer membrane protein profile in local Salmonella isolates. Food Microbiol. 18:631-635.
Jacobs-Reistma, W.F., C.A. Can and N.M. Bolder. 1994. The induction of quinolone resistant Campylobacter bacteria in broilers by quinolone treatment. Let. Appl. Microbiol. 19:228-231.
Krishnaswamy, M. and K.K. Purushothaman. 1980. Plumbagin: A study of its anticancer, antibacterial and antifungal properties. Indian J. Expt. Bio. 18:876- 877.
Kruse, H. and H. Sørum. 1994. Transfer of multiple drug resistance plasmids between bacteria of diverse origins in natural environments. Appl. Environ. Microbiol. 60(11):4015-4021.
Lakshmi, V.V., S. Padma and H. Polasa. 1989. Loss of plasmid linked antibiotic resistance in Escherichia coli on treatment with some phenolic compounds. FEMS Microbiol. Lets. 57:275-278.
Lawley, T.D., G.S. Gordon, A. Wright and D.E. Taylor. 2002. Bacterial conjugative transfer: visualization of successful mating pair and plasmid establishment in live Escherichia coli. Mol. Microbiol. 44(4):947-956. Levy, S.B. 1992. The Antibiotic Paradox. Plenum Press, New York, NY, USA.
Levy, S.B. 1997. Antibiotic resistance: an ecological imbalance. In: Antibiotic Resistance: Origin, Evolution, Selection and Spread. Willey J. & sons Ltds, England, (Ciba Foundation Symposium 207), pp. 1-14.
Lewis, K. 1994. Multidrug resistance pump in bacteria: variation on a theme. Trends Biochem. Sci. 19:119- 123.
Lyons, R.W., C.L. Samples, H.N. DeSilva, K.A. Ross, E.M. Julian and P.J. Checko. 1980. An epidemic of resistant salmonella in a nursery. Animal-to-human spread. J. Am. Med. Assoc. 243:546.
Mach, P.A. and D.J. Grimes. 1982. R-plasmid transfer in a wastewater treatment plant. Appl. Environ. Microbiol. 44:1395-1403.
Madigan, M., J. Martinko and J. Parker. 2003. Brock Biology of Microorganisms (10th Ed). Prentice Hall, Upper Saddle River, NJ, USA.
Maqueda, M., R. Quirantes, I. Martín, A. Goncálvez, M. Martínez-Bueno and E. Valdivia. 1997. Chemical signals in gram-positive bacteria: sex-pheromone system in Enterococcus faecalis. Microbiología Sem. 13:23-36.
Martel, J.L. and M. Coudert. 1993. Bacterial resistance monitoring in animals: The French national experiences of surveillance schemes. Vet. Microbiol. 35:321-338.
May, J.W., R.H. Houghton and C.J. Perret. 1964. The effect of growth at elevated temperatures on some heritable properties of Staphylococcus aureus. J. Gen. Microbiol. 37:157-169.
Mazodier, P., R. Petter and C. Thompson. 1989. Intergeneric conjugation between Escherichia coli and Streptomyces species. J. Bacterio. 171:3583-3585.
McEwen, S.A. and P.J. Fedorka-Cray. 2002. Antimicrobial use and resistance in animals. Clin. Infect. Dis. 34(Suppl. 3):S93-106.
Mel, S.F. and J.J. Mekalanos. 1996. Modulation of horizontal gene transfer in pathogenic bacteria by in vivo signals. Cell 87:795-798.
Memish, Z.A., S. Venkatesh and A.M. Shibl. 2003. Impact of travel on international spread of antimicrobial resistance. Intl. J. Anti. Agents. 21:135- 142.
Mergeay, M., P. Lejeune, A. Sadouk, J. Gerits and L. Fabry. 1987. Shuttle transfer (or retrotransfer) of chromosomal markers mediated by plasmid pULB113. Mol. Gen. Genet. 209:61-70.
Molbak, K., D.L. Baggesen, F.M. Aarestrup, J.M. Ebbesen and J. Engberg. 1999. An outbreak of multidrug-resistant, quinolon-resistant Salmonella enterica serotype typhimurium DT104. N. Eng. J. Med. 341:1420-1425.
Molnár, A., L. Amaral and J. Molnár. 2003. Antiplasmid effect of promethazine in mixed bacterial culture. Intl. J. Anti. Agents. 22:217-222.
National Research Council (NRC). 1999. Nutrient Requirements of Swine (10th Ed). National Academy Press, Washington, DC, USA, p. 97.
Neu, H.C. 1989. Overview of mechanisms of bacterial resistance. Diag. Microbiol. Inf. Dis. 12:109S-116S. Neu, H.C. 1992. The crisis in antibiotic resistance. Science 257:1064-1073.
Obaseiki-Ebor, E.E. 1984. Rifampicin curing of plasmids in Escherichia coli K-12 rifampicin resistant host. J. Pharm. Pharmacol. 36:467-470.
Poole, K. 1994. Bacterial multidrug resistance - emphasis on efflux mechanisms and Pseudomonas aeruginosa. J. Anti. Chem. 34:453-456.
Poppe, C. and C.L. Gyles. 1988. Tagging and elimination of plasmids in Salmonella of avian origin. Vet. Microbiol. 18:73-87.
Pukall, R., Tschäpe and K. Smalla. 1996. Monitoring the spread of broad host and narrow host range plasmids in soil microcosms. FEMS Microbiol. Ecol. 20: 53-66.
Ramchandani, M., A.R. Manges, C. Debroy, S.P. Smith, J.R. Johnson and L.W. Riley. 2005. Possible animal origin of human-associated multidrug-resistant, uropathogenic Escherichia coli. Clin. Inf. Dis. 40:251-257.
Ramesh, A., P.M. Halami and A. Chandrashekar. 2000. Ascorbic acid-induced loss of a pediocin-encoding plasmid in Pediococcus acidilactici. CFR K7. World J. Microbiol. Bio. 16:695-697.
Reddy, G., P. Shridhar and H. Polasa. 1986. Elimination of Col E1 (pBR322 and pBR329) plasmids in Escherichia coli on treatment with hexamine ruthenium (III) chloride. Curr. Microbiol. 13:243- 246.
Ruiz-Barba, J.L., J.C. Piard and R. Jiménez-Díaz. 1991. Plasmid profiles and curing of plasmids in Lactococcus plantarum strains isolated from green olive fermentations. J. Appl. Bacteriol. 71:417-421.
Saadoun, I., A. Elbetieha and W.T. Blevins. 1998. Mutagenesis by ethidium bromide and N-methyl-N’- nitro-N-nitrosoguanidine in off-flavor compound producing strains of Streptomyces. J. Bio. 23(5):595- 600.
Salisbury, V., R.W. Hedges and N. Datta. 1972. Two modes of “curing” transmissible plasmids. J. Gen. Microbiol. 70:443-452.
Salyers, A.A. and C.F. Amábile-Cuevas. 1997. Why are antibiotic resistance genes so resistant to elimination? Ant. Ag. Chemo. 41(11):2321-2325.
Sandaa, R.A., V.L. Torsvik and J Goksøyr. 1992. Transferable drug resistant bacteria from fish-farm sediments. Can. J. Microbiol. 38:1061-1065.
Sastre, J.I., E. Cabezón and F. de la Cruz. 1998. The carboxyl terminus of protein TraD adds specificity and efficiency to F-plasmid conjugative transfer. J. Bacteriol. 180 (22):6039-6042.
Schäfer, A., J. Kalinowski, R. Simon, A. H. Seep- Feldhaus and A. Pühler. 1990. High-frequency conjugal plasmid transfer from gram-negative Escherichia coli to various gram-negative coryneform bacteria. J. Bacteriol. 172:1663-1666.
Sia, E.A., D.M. Kuehner and D.H. Figurski. 1996. Mechanism of retrotransfer in conjugation: prior transfer of the conjugative plasmid is required. J. Bacteriol. 178(5):1457-1464.
Sokurenko, E.V., H.S. Courtney, S.N. Abraham, P. Klemm and D.L. Hasty. 1992. Functional heterogeneity of type 1 fimbriae of Escherichia coli. Infect. Immun. 60(11):4709-4719.
Soto, G.E. and S.J. Hultgren. 1999. Bacterial adhesins: Common themes and variations in architecture and assembly. J. Bacteriol. 181(4):1059-1071.
Spika, J.S., S.H. Waterman, G.W. Hoo, M.E. St.Louis, R.E. Pacer, S.M. James, M.L. Bissett, L.W. Mayer, J.Y. Chiu, B. Hall, K. Greene, M.E. Potter, M.L. Cohen and P.A. Blake. 1987. Chloramphenicolresistant Salmonella newport traced through hamburger to dairy farms. A major persisting source of human salmonellosis in California. N. Eng. J. Med. 316(10)565-570.
Stadler, J., and E.A. Adelberg.1972. Temperature dependence of sex- factor maintenance in Escherichia coli K-12. J. Bacteriol. 109: 447-449.
Stanisich, V.A. 1984. Identification and analysis of plasmids at genetic level. Met. Microbiol. 17: 6-32.
Tacket, C.O., L.B. Dominguez, H.J. Fisher and M.L. Cohen. 1985. An outbreak of multiple-drug-resistant salmonella enteritis from raw milk. J. Am. Med. Assoc. 253:2058.
Tomoeda, M., M. Inuzuka, N. Kubo and S. Nakamura. 1968. Effective elimination of drug resistance and sex factor in Escherichia coli by sodium dodecyl sulfate. J. Bacteriol. 95:1078-1089.
Trevors, J.T. 1986. Plasmid curing in bacteria. FEMS Microbiol. Rev. 32(3-4):149-157.
Van den Bogaard, A.E. 1997. Antimicrobial resistance - relation to human and animal exposure to antibiotics. J. Anti. Chemo. 40:453-454.
Van den Bogaard, A.E. and E.E. Stobberingh. 2000. Epidemiology of resistance to antibiotics. Links between animals and humans. Intl. J. Anti. Agents. 14:327-335.
Velazquez, J.B., A. Jimenez, B. Chomon and T.G. Willa. 1995. Incidence and transmission of antibiotic resistance in Campylobacter jejuni and Campylobacter coli. J. Anti. Chemo. 35:173-178.
Viljanen, P. and J. Boratynski. 1991. The susceptibility of conjugative resistance transfer in Gram-negative bacteria to physicochemical and biochemical agents. FEMA Microbiol. Rev. 88:43-54.
Waters, V.L. 1999. Conjugative transfer in the dissemination of beta-lactam and aminoglycoside resistance. Fr. Bio. 4:416-439.
Webb, V. and J. Davies. 1993. Antibiotic preparation contains DNA: a source of drug resistance genes? Anti. Ag. Chemo. 37:2379-2384.
Willetts, N. and R. Skurray. 1987. Structure and function of the F factor and mechanism of conjugation. In: Escherichia coli and Salmonella typhimurium, Cellular and Molecular Biology (Vol. 2) (F.C. Neighardt, J.L. Ingraham, K.B. Low, B. Magasanik, M. Schaechter and H.E. Umbarger, eds). American Society for Microbiology, Washington, DC, USA, pp. 1110-1133.
Woo, P.C.Y., A.P.C. To, S.K.P. Lau and K.Y. Yuen. 2003. Facilitation of horizontal transfer of antimicrobial resistance by transformation of antibiotic-induced cell-wall-deficient bacteria. Med. Hypot. 61(4):503-508.
Wray, C., R. Hedges, K.P. Shannon and D.E. Bradley. 1986. Apramysin and gentamycin resistance in Escherichia coli and salmonellas isolated from farm animals. J. Hygiene 97:445-456.
Wray, C., S. Furniss and C.L. Benham. 1990. Feeding antibiotic-contaminated waste milk to calves-effects on physical performance and antibiotic sensitivity of gut flora. Br. Vet. J. 146(1):80-87.
Zhao W.H., Z.Q. Hu, Y. Hara and T. Shimamura. 2001. Inhibition by epigallocatechin gallate (ECGg) of conjugative R plasmid transfer in Escherichia coli. J. Infect. Chemo. 7:195-197.
Zielenkiewicz, U. and P. Ceglowski. 2001. Mechanisms of plasmid stable maintenance with special focus on plasmid addiction systems. Acta Bio. Polo. 48(4):1003-1023.