Introduction
Ergot alkaloids (EA) are a group of compounds produced primarily by fungi of the Claviceps and Epichloë spp. Ergot infection has traditionally been associated with grasses and rye, however when conditions are favourable, other important cereals such as wheat, barley, triticale and oats can become infected. The fungus prefers cool, moist conditions during the flowering phase of the host plant and there is evidence that the incidence is increasing. For example, in a survey of samples submitted to the Canadian Grain Commission harvest sample program from 2002 to 2013 it was shown that both the percent of samples down-graded due to ergot and the content of ergot in the samples had increased over the decade (Tittlemier et al. 2015).
Due to the pharmacological effects of the EAs they have been extensively studied and used in human medicine (Komarova and Tolkachev 2001) to treat a wide variety of clinical conditions such as post-partum hemorrhage, migraines, hyperprolactinemia, cerebral insufficiency and others (Beede 1980). Humans can be exposed to EA via ingestion of contaminated grains and there is historical evidence of outbreaks of ergotism with gangrene, vasoconstriction and neurotoxic symptoms reported (Mulac and Humpf 2011). However, strict biosecurity means that outbreaks are now relatively rare and ergotism is primarily a problem for livestock.
Unlike other mycotoxins prevalent in Western Canada such as deoxynivalenol or zearalenone which are a concern primarily to swine and poultry, ergot affects all classes of livestock, including ruminants. Consumption of the toxic alkaloids by livestock produces neurological and/or vasoconstrictive symptoms including gangrene resulting in losses of ear, tails and sloughing of hooves, especially in cattle (Klotz 2015). Reduced feed intake, growth, reproductive problems and agalactia are observed in both cattle and swine following EA ingestion. The negative effects on feed intake are observed at low levels and probably due to direct pharmacological effects of the EA on receptors in the brain (Mulac et al. 2012). Negative effects of the EA on growth have been observed prior to decreased feed intake in swine, indicative of mechanisms independent of decreased feed intake (Oresanya et al. 2003) potentially intestinal and liver damage (Maurpo et al. 2017). Agalactia is due to the suppressive effect of the EA on prolactin, a hormone required for milk production. When nursery pigs were fed diets containing 0 to 1.0% ergot by weight, significant reductions in feed intake (13%) and growth (18%) were observed at dietary ergot concentrations of 0.10% and 0.25%, respectively. Prolactin, however, was reduced by 47% at the lowest level of ergot inclusion, 0.05% (Oresanya et al. 2003). This effect of EA on prolactin secretion has also been observed in lambs (Stanford et al. 2018) and cattle (Blaney et al. 2000) with dramatic effects on milk production and growth of offspring.
Decreased feed intake is the most sensitive indicator of the presence of EA in poultry diets, however reduced growth, diarrhea and increased mortality are also observed (Danicke 2017). There is some evidence that poultry may be less sensitive to EA than other classes of livestock (Mainka 2005) however results are variable. Danicke (2017) observed effects on feed intake with 2.5 mg/kg EA in the diet of young chicks while in a recent project conducted at the University of Saskatchewan we saw no effect on feed intake in chickens with 12 to 15 mg/kg EA in their diet (Beaulieu and Newkirk unpublished) and in work with laying hens, Danicke (2016) established a LOAEL (lowest observed adverse effect level) of 14.56 mg EA/kg diet and a NOAEL (no observed adverse effect level) of 3.72 mg/kg. Discrepancies in results may be partly due to differences in bird genetics, but are more likely a result of differences in the alkaloid composition of the ergot used in the studies, how the EA were quantified and the end-points used.
Chemistry of the Ergot Alkaloids
Although more than 50 different alkaloids are produced by Claviceps purpurea (Schummer et al. 2020) only 6 are consistently included in analytical methods. These can be divided into three related classes; clavine EA, lysergic acid derivatives and peptide EA with the latter being the most physiologically active (Hafner et al. 2008). The main EA consist of ergometrine (a simple lysergic derivative) and the ergopeptides; ergotamine, ergosine, ergocristine, α-ergocryptine and ergocornine. The amount and profile of the EA depend on fungal strain, host plant and climate (Hefner 2008). All EA have in common a tetracyclic ring system, ergoline (Figure 1), but vary in the structure of ring D, and the substitution around C8 (Komarova and Tolkachev 2001).
The physiological activity of the EA is related to the similarity of the ergoline ring to the noradrenaline transmitters, dopamine and serotonin (Figure 1; Komarova and Tolkachev 2001, Hafner 2008, Klotz 2015). The receptors associated with these amines are transmembrane Gproteins that consist of numerous families and sub-types (Klotz 2015). Ergot alkaloids can act at more than one receptor site, and the structural differences among the EAs means that binding may elicit a different response (Klotz 2015).
Figure 1. (A) Ergoline ring system common to all ergot alkaloids. Epimerization occurs at C8 and substitutions on R1 and R2 to create the various ergopeptine alkaloids. (B) Structural similarity to the catecholamines, norepinephrine, dopamine, and serotonin explains most of the physiological actions of the ergot alkaloids (adapted from Klotz, 2015).
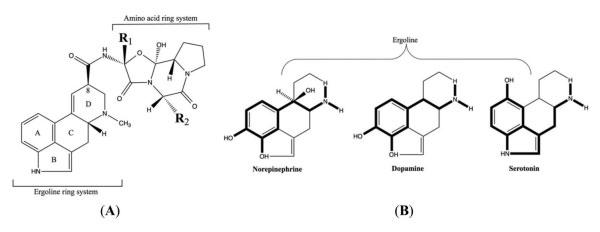
The alkaloids have a stereocenter on C8 that exists in an “R” or “S” configuration. These are also distinguished by the final syllable, “ine” for the R-form and “inine” for the S form. Both epimers coexist in nature and epimerization is rapid, spontaneous and bidirectional (Komarova and Tolkachev 2001; Schummer et al. 2020). Extraction with buffers, especially alkaline buffers favours epimerization as does UV light (Komarova and Tolkachev 2001). Hefner et al. (2008) showed that epimerization depended on the solvent used for the extraction with methanol promoting the quickest epimerization and relative stability when the EA were stored in chloroform. Except for ergotametrine which was stable and did not epimerize to ergotametrinine, regardless of the solvent used, the ergopeptides, ergosine, ergotamine, ergocornine, α-ergocryptine and ergocristine behaved similarly with rapid epimerization (Hefner 2008). Following incubation of EA contaminated cookies in simulated salivary, gastric and duodenal juices, the percent of the “R” epimer of ergotamine and ergosine increased while ergocornine, α-ergocornine, ergocryptine and ergocristine shifted towards the “S” epimer (Merkel et al. 2012). Further studies indicated that the epimerization occurred primarily in the duodenal juice (pH 7.5) with little occurring in the saliva (pH 6.4) or gastric juices (pH 2.0; Merkel et al. 2012)
Despite their structural similarity, there is some evidence that EA vary in toxicity. For example, in a review of EA levels associated with gangrenous ergotism, intakes of 0.009 to 0.016 mg/kg BW ergovaline produced symptoms in cattle and sheep while 1.0 mg/kg ergotamine was required to produce symptoms in sheep (Klotz et al. 2010). In contrast, pelleting diets had no effect on total ergot content, but resulted in increased proportions of ergocristine and decreased ergotamine. Lambs fed the ergot contaminated, but pelleted diets had improved growth relative to those fed the unpelleted, contaminated diets indicating that the reduction in ergotamine may be responsible (Coufal-Majewski et al. 2017). Similarly, in an in vitro model of contractile response, ergovaline acted as an agonist in smooth muscle with maximal stimulation while ergocristine and ergocornine produced only 40 to 50% maximal stimulation (Klotz et al. 2010). Based on several parameters, ergocristine was shown to be the most toxic to cultured human renal cells, while ergometrine had no effect (Mulac and Humpf 2010). Similarly, in a model system of porcine brain endothelial cells it was demonstrated that the peptide EA could cross the blood brain barrier in high quantities within a few hours, while ergometrine (lysergic acid) required active transport (Mulac et al. 2011). The authors (Mulac et al 2011) noted that the peptide EAs are highly lipophilic compounds while the lysergic acid amides are slightly more soluble.
There are also consistent statements found in the literature such as “:..the alkaloids exist as different epimers, and there is evidence that one class of epimer (‘in’ or ‘R’ epimer) is more potent than the less active ‘inine’ (S) epimer” (Wolff et al. 1998) or “inine form is assumed to be inactive” (EFSA 2012). Although this observation is commonly stated (eg; Komarova and Tolkachev 2001; Merkel 2012; Danicke 2016; Schummer et al. 2020) it is difficult to find the experimental data supporting this claim. Several references lead back to a short article (less than 1 page in German) by Stadler and Sturmer (1970) and to Weber (1980). Weber (1980) describe a series of experiments examining the physical differences among the epimers and the potential for differences in biological activity but cites Sturmer (personal communication) as a reference for the data supporting his hypothesis of differential biological activity of the epimers.
Recent work has challenged this hypothesis. Ergocryptinine, ergocornine, ergocristinine and ergotaminine (“S” epimers) were all found to be vasoactive when tested in an in vitro system with bovine metatarsal arteries (Cherewyk et al. 2020) and had similar activity to that reported by others (ie. Klotz et al. 2010) for the “R” epimers. As mentioned above, using a model of the blood-brain barrier, while only the “R” epimers were able to cross the blood-brain barrier, the “S” epimer, ergocristinine was unable to cross; but this resulted in significant levels of cellular accumulation and disruption of the barrier integrity (Mulac et al. 2011).
Strategies to Mitigate Ergot Content of Feeds and Toxicity
Physical separation
Agronomists have several “tools” to limit contamination of crops with Claviceps spp and thus maintain grain quality (www.saskatchewan.ca). However, unpredictable weather and environmental conditions, means that periodic outbreaks still occur. The sclerotia or ergot bodies are black or purple and usually larger than the grain and thus easily separated from the grain by gravity separation or optical sorting. However, while this can reduce sclerotia content, these methods can be expensive and not always practical (Miedaner and Geiger 2015). Moreover, byproducts from the milling process, which are often used in animal feeds, are a concentrated source of EAs (Tittlemier et al. 2019).
Hydrothermal processing
Hydrothermal processing refers to the use of heat, moisture and often pressure to change the physical and chemical characteristics of a feed, resulting in improved handling characteristics and nutrient digestibility. Steam explosion is a form of extreme hydrothermal processing. It consists of a steam explosion phase with temperatures of 170 to 210 ⁰C and an explosion phase to convert the thermal energy into mechanical energy. It is commonly used in the bioprocessing industries to disrupt lignocellulosic materials to make the cellulose available to further enzymatic treatment (Ziegler-Devin et al. 2021). A similar process is “expansion”, which is primarily used as a pre-treatment prior to pelleting. It is characterized by high temperature, and short time where steam-conditioned feed is forced through a barrel into an outlet when rapid expansion of the feed occurs (Danicke 2016). Expansion of diets contaminated with ergoty rye reduced total EA content by 11%. When the diets, containing up to 14 mg/kg EA, were fed to laying hens it was observed that while most performance parameters were compromised by the EA, this negative effect was mitigated when the diets were treated by expansion (Danicke 2016).
Steam explosion of heavily contaminated wheat and rye screenings reduced total EA content by 40 to 50% (Figure 2. Beaulieu and Newkirk, unpublished). Moreover, the response of the individual EA was variable with increases in proportions of ergocristine and ergotamine and decreases in ergocryptine and ergometrine following the SE (Figure 2). The processed or unprocessed wheat screenings, incorporated into diets to obtain final EA content of 0, 0.5, 1, 2 or 4 mg/kg were fed to newly weaned piglets for a 28 day trial. Overall, growth and feed intake were not significantly affected by EAs in the diet. The exception was during the first week, when growth rate was severely depressed by EA, but only when unprocessed (Figure 3; EA by processing interaction, P < 0.01). A similar response was observed with serum prolactin which was depressed at all levels of EA in the diet, but more so when the screenings were unprocessed (data not shown).
Figure 2. The change in proportions of wheat EA following steam explosion (SE). The total content of the EA was decreased by 40% with SE.
Figure 3. The response of weanling piglets (day 3 to 10 post weaning, initial BW 6.7 kg) to ergot alkaloids in the diet from contaminated wheat screenings processed by steam explosion.
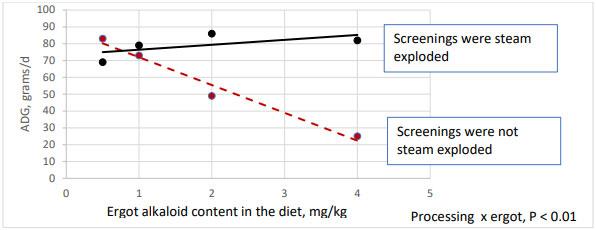
The potential importance of the altered EA profile from hydrothermal treatment of the feed is also highlighted by the work of Coufal-Majewski et al. (2017). Pelleting diets for lambs had no effect on total EA content, but ergotamine and ergosine were 2-3 times greater in mash feeds, while ergocornine, ergocristine and ergometrine were 2-3 times greater in pelleted diets. Lambs fed the pelleted diets had 60 g/d greater ADG than those fed the mash diets. Moreover, the ergot induced depression in serum prolactin was less when the diets were pelleted (Coufal-Majewski et al. 2017.) As mentioned previously, there are numerous suggestions in the literature of a differential role of the “S” and “R” EA epimers, specifically referred to as the “biological inactivity of the ‘S’ epimer”. Moreover, it has been demonstrated that processing affects this ratio. For example, baking cookies with ergot contaminated rye flour resulted in a 2-30% degradation of the EAs, plus a shift to the “S” epimer for all EAs (Merkel et al. 2012) and Tittlemier et al. (2019) reported a higher proportion of the “S” epimers resulting from the milling and cooking during the production of spaghetti.
We (Beaulieu and Newkirk) have tested out the hypothesis that “processing reduces EA toxicity by promoting a shift from the ‘R’ to the ‘S’ epimer” with experiments in swine and poultry. Diets formulated to contain 4 mg/kg EA using heavily contaminated wheat screenings, processed or not by extrusion (90 ⁰C; 80–100psi), or pelleting (90 ⁰C) were fed to growing pigs from 65 kg to 120 kg BW. Extrusion had no effect on total EA content or profile of the individual alkaloids (data not shown). However, there was a marked shift in the epimer ratio, resulting from both a decrease in the “R epimer” and an increase in the “S” epimer (Figure 4). Pigs fed the ergot contaminated diets were apparently healthy but had reduced growth and feed intake (Table 2). The lack of an effect on feed efficiency is evidence that reduced feed intake was the primary effect of the EAs. Extrusion, and the resulting shift in the epimer ratio, had no effect on the response of pigs to the EA contaminated diets (EA by extrusion interaction, P > 0.10).
Figure 4. The percent change of the R and S epimers from the unprocessed control following extrusion of ergot contaminated wheat screenings.
Table 1. The response of growing pigs to diets contaminated with 4 ppm ergot alkaloids, unprocessed or extruded.
In a similar experiment, diets were formulated for poultry to contain 0, 6, 12, 18 or 24 mg/kg EA (R + S epimer). The EA were obtained from heavily contaminated wheat screenings which were processed by either extrusion or pelleting prior to incorporation into the diet. Thus, there were 15 experimental treatments (5 EA levels x 3 processing treatments). These diets were fed to 480 Ross 308 chicks, housed 4 per cage. Temperature of a toe was taken individually using a thermocouple and a plastic clip attached to the middle toe of the right foot. Ergot alkaloid of the diets indicated they contained approximately 2.5 times the expected, formulated levels of EA. Birds were removed from the high EA treatments when toxicity symptoms were observed.
Neither pelleting nor extrusion resulted in the changes in the EA profile that has been reported by others (ie. Coufal-Majewski et al. 2017). However, processing consistently decreased the proportions of the “R” (ine) epimer and increased proportions of the “S” (inine) epimer, and this response was greater with extrusion than pelleting (Table 2). Overall, this resulted in a decreased R:S ratio with increased processing intensity.
Table 2. Changes in the ergot alkaloid epimer content and percentage when heavily contaminated wheat screenings were processed by pelleting or extrusion1.
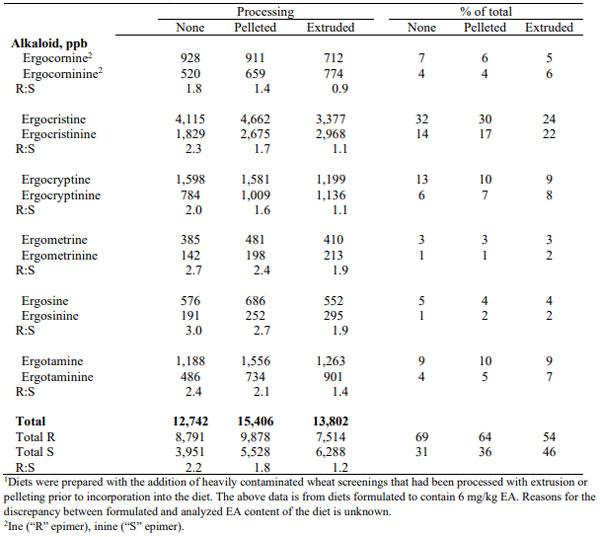
Birds responded to increasing ergot content of the diet with reduced BW (Table 3a), feed intake (Table 3b) and external body temperature (Table 3c; P < 0.001). Feed intake and BW were comparable between birds consuming the 0 mg/kg diet and those consuming the diet formulated to contain 6 mg/kg. The analysis of this diet indicated 13 to 15 mg/kg EA, confirming our earlier observations that, compared to other livestock, poultry are relatively insensitive to EAs. The main effect of processing was significant for BW and feed intake. This is difficult to explain as only the screenings (ergot contaminated or not) were subject to processing prior to incorporation into the diet. Importantly, there were no EA level by processing interactions, indicating that even though processing changed the epimer ratio, this had no effect on EA toxicity. Further work is required to determine what hydrothermal processing conditions might cause a shift in EA profile and if this is indeed responsible for the observed effects.
Table 3a. Effect of ergot inclusion level and processing method on the body weight (g) of Ross 708 broilers1,2.
Table 3b. Effect of ergot inclusion level, processing method, on the average daily feed intake of Ross 708 broilers (g/bird/day).
Table 3C. Effect of ergot inclusion level, processing method, and sex on the external temperature of Ross 708 broilers at 29 days of age (°C)1.
Summary and Conclusions
Severe hydrothermal processing (SE) of contaminated screenings reduced total EA content and the negative effects of the EA’S in a piglet model. However moderate processing; pelleting or extrusion, had no effect on total EA content or profile, but reduced the “R” epimer content and the overall R:S ratio. In contrast to our original hypothesis, this had no effect on toxicity of EA in a poultry or growing pig model. This implies that the “S” epimers of EAs have some or equivalent biological activity to the “R” epimers and need to be included in analysis and the consideration of amounts in livestock diets.
Presented at the 2022 Animal Nutrition Conference of Canada. For information on the next edition, click here.