In the feed manufacturing industry, particle size reduction (grinding) is second only to extrusion processes (pelleting, expanders, extruders) in terms of total energy consumption. To achieve a finer grind (smaller finished particle size), energy and maintenance costs increase and, everything else being equal, the capacity of the grinding system will be reduced.
The Cost(s) of Grinding
The cost of grinding can be broken down into three general categories; equipment cost, energy cost, and maintenance cost. While the initial equipment cost is the most obvious, it certainly should not be the only consideration when designing or installing a grinding system. In many modern, high production plants where the equipment runs two or more shifts per day, the cost of energy during one year can easily exceed the cost of a new hammermill. In other words, the energy to operate a hammermill or roller mill during its normal expected life will be 10 to 20 times more expensive that the machine cost alone. It is therefore very important to make sure the equipment selected is carefully matched to the specific grinding task to insure most efficient operation. For hammermills, the diameter (tip speed) and screen area must be adequate to effectively utilize the connected HP. While other parameters such as hammer pattern and screen size can be changed, the tip speed and available screen area are determined with the machine purchase. In the case of roller mills, the rolls must have sufficient surface are to be able to utilize the connected HP. As HP goes up, the roll diameter and length must increase to insure the machine can operate with maximum efficiency, and that an acceptable roll life will be achieved.
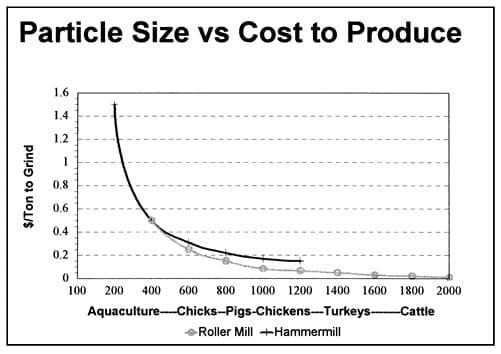
Maintenance costs for hammermills include the screens and hammers, as well as a variety of other wearing items as detailed later. For many customers, maintenance parts are an obvious cost, and one that is quite easy to track through purchasing records, etc. For this reason, many customers are tempted to try and save some cost by using lower cost parts, or by using the screens and hammers beyond a reasonable useful life. By comparison, the maintenance parts cost for operating a hammermill are generally only 10 to 20% of the total grinding cost. In nearly every instance a strong case can be made for the use of high quality (i.e. high cost) wear parts in order to maintain the maximum efficiency, lowering the energy cost per ton, and thus insuring the lowest operating cost per ton.
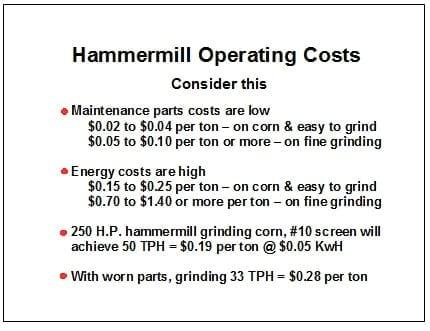
Maintenance costs for roller mills are generally higher on a per ton basis, typically ranging from $0.05 to $0.09 per ton depending on the size of the machine and the finished particle size required. The cost of recorrugation is the most significant factor accounting for 60 to 70% of the total maintenance cost. Other regular maintenance parts include v-belts, cheek plates (used to prevent material from passing the ends of the rolls unprocessed), and dust seals. In most cases, the bearings used on roller mills are designed to last the life of the rolls so routine replacement of bearings is not required. Keeping the rolls properly adjusted and maintaining belt tension will contribute significantly to keeping the maintenance cost of a roller mill as low as possible.
Why process at all?
Why process at all? Of course, the answer is ultimately feed efficiency, producing the most milk, eggs, meat or fiber at the lowest possible cost. Particle size reduction as the first step in the feed manufacturing process works toward the goal of improved feed efficiency by increasing the surface area of the materials being processed. This increases the amount of materials exposed to the animal’s digestive system and ultimately leads to more complete digestion, thus better feed efficiency. Particle size of ground feed ingredients also has a direct influence on subsequent processing and handling. To produce pellets or extruded feeds of acceptable quality the particle size of the ground materials must be correct. Generally speaking, finer grinding will result in a better quality pellet or extruded feed, increases the capacity of the pellet mill or extruder, and reduces wear of the pellet mill or extruder working parts such as dies, rollers, and worms.
Because animal needs vary considerably, the degree of processing for various diets also must vary. Ruminant animals such as cattle and sheep have rather long, complex digestive tracts and so require a less processed feed material. On the other hand, many of the ingredients used in ruminant feed pellets consist of low protein, high fiber material so fine grinding may be required in order to achieve a reasonable pellet quality. Swine have a fairly short, simple digestive system (much like humans) and therefore benefit from a more highly processed feed. Poultry have a short but rather complex digestive system and, depending on the make up of the diet, can efficiently utilize feedstuffs less highly processed than swine. The size and the age of the animals also affect the dietary requirements so far as particle size is concerned. Generally speaking, younger animals require a finer, more highly processed feed than do older, more developed livestock.
How fine do you grind?
Determining and expressing fineness of grind has been the subject of study as long as feed ingredients have been prepared. While appearances or feel may allow an operator to effectively control a process, subjective evaluation is inaccurate at best and makes objective measurement and control virtually impossible. Descriptive terms such as coarse, medium and fine are simply not adequate. What is “fine” in one mill may well be “coarse” in another. Describing the process or equipment is also subject to wide differences in terms of finished particle size(s) produced. Factors such as moisture content of the grain, condition of the hammers and/or screens (hammermill) or the condition of the corrugations (roller mills) can produce widely varying results. In addition, the quality of the grain or other materials being processed can have a dramatic impact on the fineness and quality of the finished ground products.
The best measurement of finished particle sizing will be some form of sieve analysis, expressed in terms of mean particle size or percentage (ranges) on or passing various test sieves. A complete sieve analysis will not only describe the average particle size but will also indicate peculiarities in the distribution, such as excessive levels of fine or coarse particles, etc. Typical descriptions that lend themselves to objective measurement and control might be “corn ground to 750 microns” or “75% < 14 mesh”.
Particle Size and Distribution
The most common way to analyze ground feed materials for particle size and distribution (uniformity) is to perform a complete a sieve analysis. The particle size distribution of common ground feed materials is skewed when plotted on normal – normal graph paper; when plotted on log-normal graph paper, the curve becomes more like the typical bell shaped curve. In order to make reasonable comparisons between samples, the American Society of Agricultural Engineers (ASAE) has defined a procedure, ASAE S319.1 based on a log-normal distribution of the ground particles.
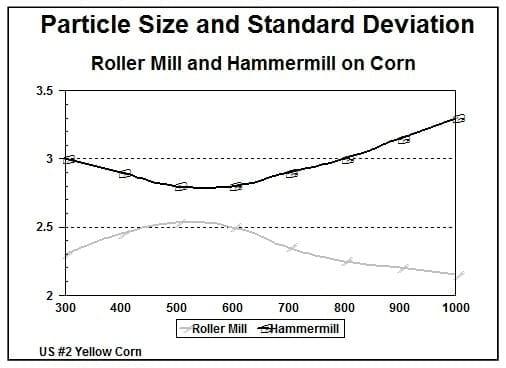
This method involves sifting a sample of ground material through a set of 14 test sieves, weighing the fraction on each sieve, and computing the “geometric mean particle size”. This figure represents the mid point (mean) of the distribution, where 50% of the material by weight is coarser, and 50% of the material by weight is finer. Although technically it is not correct, the mean particle size (in microns or µ) is commonly referred to as the “average” or the “micron size”. Another common calculation performed in the size analysis procedure is to determine the “log-normal standard deviation”. For most feed materials ground through a roller mill, the log-normal standard deviation will be in the range of 2 to 2.5. For most feed materials ground through a hammermill the log-normal standard deviation will be from 2.5 to 3.5.
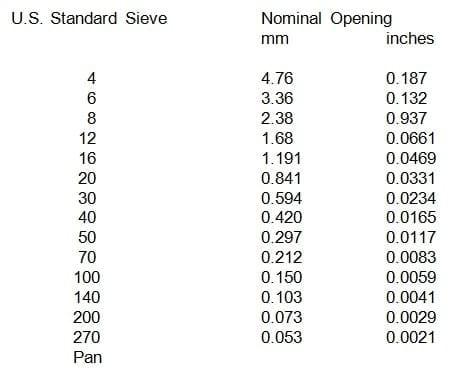
In order to obtain an accurate description of the ground material, the sieve analysis must thoroughly separate the fine particles. In order to achieve this separation the sample size must be correct (100g as prescribed by the ASAE procedure) and normally a sieving agent will be added to insure fine particles are completely separated and sifted. The sieving agent serves to keep smaller particles from sticking together and insures a complete separation of the sample into the various fractions.
Differences in particle size analysis can come from a number of causes including incorrect sampling or sample division, inadequate separation in the sifting (sieving), screens blinding due to oil, moisture, or electrostatic charges, and errors in math or procedures. One common mistake is failing to use all of the sieves as described in the procedure; the missing fine sieves artificially shift the distribution coarser and more uniform. As grain quality changes, the characteristics of the ground materials will vary as well. Even the variety (hybrid or type) of grain, especially with corn, can affect the quality and consistency of the finished ground material. Grain with more hard, horny endosperm will tend to product samples that are coarser, and contain fewer fines and a lower log-normal standard deviation; grain with more soft, floury endosperm will produce finer finished products with more fines and a higher log-normal standard deviation.
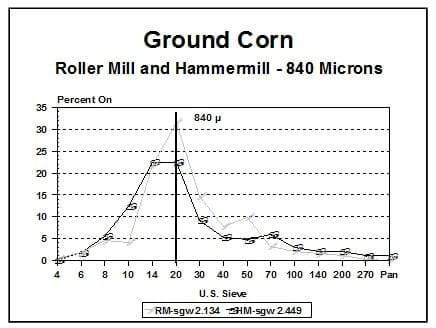
To illustrate how the mean particle size and log-normal standard deviation numbers really work, consider these examples of hammermill and roller mill ground corn.
Hammermill, 841µ mean particle size, log-normal standard deviation 2.449
Log 841 = 2.925, log 2.449 = .389
One standard deviation would be 2.925 plus / minus .389 or
2.925 + .389 = 3.314 10^3.314 = 2060µ
2.925 - .389 = 1.906 10^1.906 = 80µ
67% of the material would be between 2060 and 80 microns
Roller mill, 840µ mean particle size, log-normal standard deviation 2.134
Log 840 = 2.924, log 2.134 = .329
One standard deviation would be 2.924 plus / minus .329
2.924 + .329 = 3.253 10^3.253 = 1790µ
2.924 - .329 = 2.595 10^2.595 = 393µ
67% of the material would be between 1790 and 393 microns
Grinding equipment
Both roller mills and hammermills have been applied to the task of particle size reduction or grinding in feed milling applications. Hammermills have traditionally been used to produce the finer grinds commonly used for pelleting and for many mash (meal or non-pelleted) feed applications as well. The hammermill is a relatively simple machine and requires a fairly low degree of skill in regards to both the operation and maintenance.
However, recent significant changes in the industry have caused many to reassess their approach to particle size reduction. Increasing energy costs, increasing customer awareness of feed quality and environmental concerns all challenge the validity of the hammermill as the only choice for particle size reduction (grinding) applications. In the following discussions, both roller mills and hammermills will be looked at in terms of equipment selection, operating conditions and parameters, and relative costs to acquire and to operate.
Roller Mill Grinding
Roller mills have been used in the processing of common feed materials for years. The earliest roller mills used in the feed milling were abandoned flour milling roll stands, used primarily to produce coarse granulations of friable materials. Over time, roller mills have been used to perform a wide variety of tasks related to the production of animal feeds.
Roller Mill Equipment Description
Roller mills are commonly referred to by the type of service they perform. A mill used to crack grain or other types of friable materials may be called a cracking mill. Mills used to flake grains or other products may be called flaking mills or flakers. Roller mills used to grind in a feed mill are commonly referred to as a roller mill or roller mill grinder
Double pair (two pair high) roller mills may be utilized in feed milling operations when two distinctly different grains are processed through one mill. A machine processing both corn and oats, for example, requires one set of coarse grooved rolls to crack corn and one set of fine grooved rolls to be able to effectively process the oats. A double pair mill equipped with differential roll speeds (one turning faster than the other) can be utilized as a grinder to reduce all kinds of friable materials, including grains, pelletized products, oilseed and by-product meals, and many other common feed ingredients. Double pair mills are usually referred to as roller mills or roller mill grinders.
Triple pair (three pair high) mills are used for special applications requiring a finer finished product or when a wide range of materials will be processed through the same machine. A triple pair mill may be employed to achieve a variety of finished products from different feed stocks such as whole grain, mixed meals, or other combinations. Occasionally, three pair high roller mills will be used to permit one machine to serve as both a two pair high grinder and a single pair cracking/crimping mill.
Basic Machine Characteristics
Roller mills used in various feed processing applications will have some common characteristics as well as certain features peculiar to specific tasks such as machines used to flatten (crimp) small grains or crumble pellets. All roller mills will have some kind of framework to house the rolls and contain the roll separating forces experienced in operation. This basic frame must be robust enough to hold the rolls securely in position during operation, yet allow easy access to the rolls for normal service. In any roller mill, the rolls will need to be removed periodically for recorrugation. This very important detail must be carefully studied when roller mill selection is made and the installation is laid out.
Generally, one roll is fixed in the frame and the opposing roll can be adjusted to set the clearance or gap between the rolls. This roll gap adjustment needs to be quick and easy and must accommodate the requirement of maintaining the rolls in parallel. Common systems employ screws, cams, or fluid-operated (hydraulic or pneumatic) cylinders to achieve this roll adjustment. Adjustment can be manual or remote operated and may feature some means to display the roll gap setting at a remote location. Roll corrugations (also described as roll cut or fluting) will vary depending on the material to be processed, initial and finished product sizes and the product quality (amount of fines) desired. Coarse grooving will produce a coarse finished product at high capacities while finer grooving produces a finer finished product at lower capacities.
While flour milling may require many different corrugation styles to produce the desired finished products, feed processing can usually be accomplished with less sophisticated roll corrugations. The most commonly employed corrugation styles for roller mill grinding will be Round Bottom Vee (RBV). For certain special applications such as high moisture grain, some form of a raked tooth with different leading and trailing angles, commonly known as Sawtooth may be beneficial. Occasionally, crumbler rolls (roller mills dedicated to the reduction of pellets) will feature a classical LePage cut, with one roll corrugated longitudinally and one corrugated circumferentially. The circumferential roll will often be equipped with a groove known as the LePage ring cut.
Rolls may operate at differential speeds depending on the task the mill is called to perform. Cracking, crimping and flaking use lower roll (peripheral) speeds - 1,000 feet/minute (fpm) up to 2,200 fpm and no roll speed differentials. Mills used to grind will operate with higher roll speeds - 1,500 fpm up to 3,000 fpm with roll speed differentials. Roll speed differentials simply means one roll turning faster than the other and is usually described in the form of a ratio, slow roll speed expressed as 1. For example, rolls operating at 1.5:1 differential with a fast roll speed of 1,000 RPM would have the slow roll turning 667 RPM.
Grinding with a Roller Mill
In recent years, more attention has been given to the roller mill set up to function as a grinder. Several important factors have contributed to this including energy costs, product quality concerns, and environmental issues.
Energy costs have escalated dramatically in the last 20 years and, at the same time, margins in feed manufacturing have decreased. As a result, cost savings of $0.10-$0.40 per ton for grinding can mean a significant difference in the bottom line of a feed manufacturing operation. Because of an efficient reduction action, roller mill grinders will product 15-40% more tons/hour at a given horsepower than traditional “full-circle” hammermills when producing the same finished particle size. Roller mill energy savings advantages will be even greater when compared to older half screen hammermills with direct connected fans. In many instances, the energy savings potential of a roller mill grinder will justify the capital expenditure.
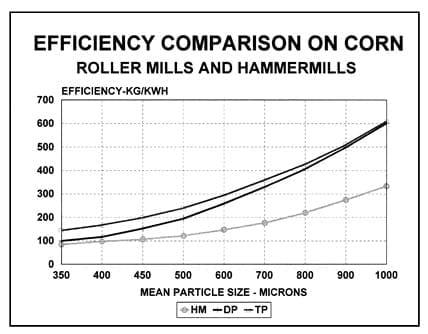
Product quality concerns have always been a part of feed manufacturing and there are many quantitative methods for measuring feed quality. Nonetheless, the physical traits (appearance, feel, handling characteristics) will always influence the feed buying customer. Because the grind produced by a roller mill is very uniform, the finished product(s) have an excellent physical appearance. The low level of fines and lack of oversize particles make a feedstuff with excellent flow and mixing characteristics. This is especially important for mash or meal type feeds where the flow from the bins and feeders can be difficult to regulate and where segregation and separating may occur in shipping and handling. Because the product is not heated significantly in the grinding process, less moisture is driven off and the finished product is not prone to hanging up in the bins, spoiling in storage, and other maladies related to heat and moisture. Figure 5 illustrates the difference in particle size distribution on corn ground to similar finished mean particle sizes through both roller mill grinders and hammermill grinders.
Environmental issues of concern to the feed manufacturer today include particulate emission, employee exposure to noise, and the risk of fire and explosion. Because roller mill grinders create fewer fines, less material is likely to be lost to the atmosphere. Additionally, high efficiency hammermill installations require air assist to achieve the rated performance. Cyclones and bag filters are not 100% effective in removing the particulates from the air streams and so some emissions occur. Whether or not these emissions are a problem will depend on widely varying local conditions and regulations. Because roller mill grinders operate at lower speeds and with a different kind of reduction action, less noise is generated in the grinding process. In many cases, this reduction in noise means a roller mill grinder will not require a separate enclosure to limit employee exposure to high noise levels. Lower operating speeds in roller mill grinders mean less frictional heating and less inertial energy (such as thrown hammers) in a hammermill. This reduction in ignition source, combined with less dust in the product steam, greatly reduces the risk of fire in the grinding operation.
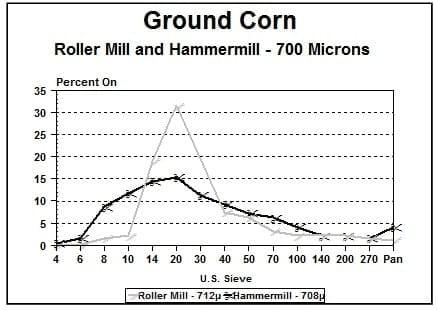
The Roller Mill Grinder
Not every make or style of roller mill can be applied to the task of grinding. Essentially, a roller mill grinder will utilize rolls from 9-16 inches in diameter operating at differential speeds. Roll speeds will be higher for roller mill grinders than for cracking/crimping and flaking mills. Typical peripheral speeds range from 1,500 fpm to more than 3,000 fpm for 16” diameter rolls. Due to higher speeds and greater loads, the bearings and shafts of a roller mill grinder experience far more demanding conditions than cracking/crimping mills.
Differential ratios vary from about 1.2:1 up to 2:1 for typical feed milling operations. Lower differential ratios do not permit adequate reduction, while higher ratios can lead to excessive roll wear. It is essential that the roll speed differential be maintained when operating at full motor loads in order to achieve the desired grinding results.
Because the roll clearances need to be maintained under demanding conditions, the mill housing and roll adjustment mechanism of the roller mill grinder must be more robust than for cracking and crimping mills. More precise roll position adjustments must be made and better control over the feeding is necessary in order to achieve the full benefits of the roller mill grinder through its range of capabilities. Rolls must be operated in parallel and tram to reliably produce quality finished products. For these reasons, many of the existing cracking and crimping mills cannot be made to function effectively as a roller mill grinder. The illustrations here show roll conditions of tram and parallel.
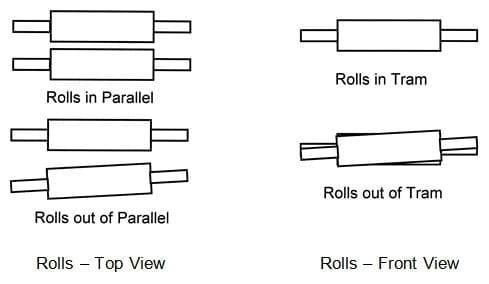
Roll feeders or pocket feeders are generally preferred for a roller mill grinder to insure a uniform feed across the full length of the rolls. Pocket feeder have the inherent advantage of utilizing conventional inverter (Variable Frequency Drive) technology to control the feed rate, and simplify automation where required.
Cleaning grain ahead of a roller mill can improve the roll life and the quality of the finished product(s). Normally all that is required is some form of scalper to remove gross oversize pieces - stalks, cobs, clods, stones and the like. Magnetic protection ahead of the mill will insure a minimum amount of tramp metal enters the rolls. While grain for a roller mill grinder does not require any more cleaning than grain going to a hammermill, some objectionable fibrous materials may be passed unprocessed through a roller mill grinder. Rolls tend to be self-limiting in so far as the size of materials that will be pulled into the nip. Rolls cannot get a purchase on large stones, etc. and, though roll wear may be accelerated by the presence of such objects, the mill is not likely to suffer acute failures. Grain sized bits of stone, iron and such that escape the cleaning system will generally pass through the machine without any significant impact on the processing as the rolls can open (with spring protection) and close again.
The primary claims against the roller mill grinder are high initial cost, maintenance hours to change rolls, and the need to carry spare rolls in stock. Roller mills are generally more expensive than hammermills of equal capacity, but total installed costs for the two systems are not so different when all factors are considered. Items such as larger motors, starters, and wiring, air assist systems (including fans and bag filter units), and additional labor to install the more complex material handling systems of hammermills tend to offset the differences in the basic equipment costs. Because roller mill maintenance (roll change) occurs in a concentrated block, the actual time required appears to be significant. In fact, when compared on a “maintenance hours per ton” basis, roller mill grinders are quite competitive with hammermill grinders. Finally, spare rolls may amount to a fair capital investment but, again comparing the actual cost on a “perton” basis, the maintenance costs of recorrugation and roll replacement are within $0.01-$0.03 per ton of hammermill maintenance costs. Due to the significantly lower energy cost per ton, the roller mill offers an overall lower cost per to grind corn and similar feed materials.
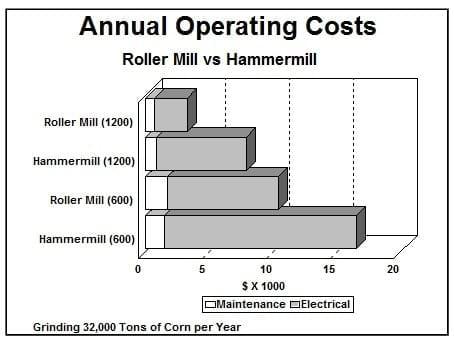
Hammermill Processing
Hammermills have long been used for particle size reduction of materials used in the manufacture of animal feeds. At the same time, it is not far from the truth to say that the hammermill has been the most studied and least understood piece of equipment in the feed manufacturing plant. Much of this confusion has come about over the years as a result of hit or miss problem solving, changing several variables at once when testing or problem solving, and by treating symptoms rather than addressing the root causes when treating operational problems.
On the other hand, a well-designed hammermill grinding system will offer good long term performance and require a minimum amount of attention if a few basis considerations are made at the time the equipment is selected. The following discussion will explore both the theory of hammermill operation as well as supply the good, hard engineering principles on which systems may be successfully designed.
While hammermills are primarily applied to the task of grinding (significant particle size reduction), they are also used at times to produce coarse granulations, crack grain and even, in some cases, to homogenize mixtures of materials. Every effort will be made to explore these alternative applications and to offer the best information available dealing with each peculiar task.
Equipment Description
A hammermill consists of a rotor assembly (two or more rotor plates fixed to a main shaft) enclosed in some form of grinding chamber. The actual working mechanisms are the hammers, which may be fixed or swinging and the screen or grinding plates that encircle the rotor. The rotor may be supported from one end only (overhung) or supported on both ends by the shaft and bearings. For modern, high capacity machines in widths of 12” up to 48”, the rotor is normally supported on both ends. This provides a more stable running mill and reduces the tendency for a rotor shaft to “wind up” or run out of true under load. The hammers are simply flat metal bars with a hole at one or both ends and usually have some form of hardface treatment on the working end(s). The hammers may be fixed, fastened rigidly to the rotor assembly, but much more common is the swinging hammers, where the hammers float on pins or rods. This swinging hammer design greatly facilitates changing hammers when the working edges are worn.
Reduction in a hammermill is primarily the result of impact between the rapidly moving hammer and the incoming material. There is some attrition (gradual reduction by particles rubbing) between the particles and between the hammers and the screen.
The efficiency of the grinding operation will depend on a number of variables including, but not limited to, screen area/horsepower ration, screen (hole) size and open area, tip speed, hammer pattern (number of hammers), hammer position (coarse or fine), uniform feed distribution, and air assist. In addition, the nature and quality of the material(s) being processed will affect the performance of the hammermill.
Basic Machine Characteristics
Hammermills used in feed processing have some common characteristics but equipment manufacturers differ significantly in how they achieve those same characteristics. For the purpose of this discussion, here a number of basic design principles will be reviewed as they apply to maximizing the performance and minimizing the cost of operating a hammermill system.
Full Width Top Feed
The modern hammermill design must include a full-width top feed in order to achieve maximum efficiency and minimize the cost of operation. A full width top feed insures the entire screen area can be utilized and that the work being accomplished will be evenly distributed across the full hammer pattern. The full width top feed also permits the direction of rotation to be changed, allowing two corners of the hammer to be utilized before a physical change of the hammer is required.
Tear-Shaped Grinding Chamber
A tear-shaped grinding chamber is necessary to prevent material from merely circulating within the grinding chamber. Most well designed modern hammermills have some sort of flow director or diverter in the top of the hammermill to properly feed the hammermill (right relationship of incoming grain to the direction of the hammers) and to positively stop any materials that are circulating within the grinding chamber. Hammermills with circular screens lack this important action and so are more prone to near size material traveling around with the hammers, increasing product heating and reducing capacity.
Split Screen/Regrind Chamber
The tear-shaped screen should be split in two pieces, with some device at the bottom of the mill to disrupt the flow of materials within the grinding chamber. This device must be large enough to take products out of rotation and redirect them back into the path of the hammers, but should not be so large as to subtract from the screen area available for grinding. The application of a split screen design will permit the user to adjust the screen sizing on the down side and up side to maximize productivity and product quality.
Outboard Supported Rotor
As noted earlier, the rotor should be supported at each end, preferably with standard bearings and bearing housings. This will provide a degree of rigidity not available with an “overhung” rotor design and reduce any problems with rotor shaft “wind up”, even if the mill operates with an out of balance rotor. Adequate support for the rotor is particularly important with today’s increased capacity demands, requiring wider machines. The use of standard bearings and housings is an added benefit to the customer by increasing the availability of replacement parts should the need arise.
Rigid Rotor Support
In order to maintain the relative position of the rotor to the grinding chamber (screens and supporting mechanisms) the foundation of the mill must be extremely rigid since, even under normal circumstances, a hammermill will be subject to vibration and shock. A rigid structure positively maintains the clearances between the hammer tips and the screen through the full rotation for consistent, efficient processing. This must be accomplished without sacrificing the accessibility to the grinding chamber, as routine maintenance of the hammers and screens will be required.
Replaceable Wear Items
One final rule for a good hammermill design is “if it can wear, it should be replaceable”. Beyond the hammers, screens and pins, every component within the hammermill will be subject to wear. Accordingly, these components should be fabricated from wear resistant materials, heavy enough to provide good service life and ultimately should be reasonably simple to replace.
Basic Operational Concepts
What is intended to take place inside a hammermill is the uniform, efficient reduction of the material introduced into the grinding chamber. This particle reduction occurs as a result of the impact between a rapidly moving hammer and a relatively slow moving particle. If sufficient energy is transferred during the collision, the particle breaks and is accelerated towards the screen. Depending on the particle size and the angle of approach, it either passes through the screen or rebounds from the screen into the rapidly moving hammers again. As materials move through the grinding chamber they tend to approach hammer tip speed. Since reduction only occurs when a significant energy is transferred from the hammer to the particle (large difference in velocities), less grinding takes place when the particles approach hammer tip speed. Many manufacturers incorporate devices within their mills to interrupt this product flow, allowing impact and reduction to continue. Tear circle hammermills have a more positive, natural redirection of product at the inlet than “full circle” design machines.
While the basic operational concepts are the same for all hammermills, the actual unit operating conditions change rather dramatically depending on the materials being processed. Grains such as corn, wheat, sorghum and various soft stocks, like soybean meal, tend to be friable and easy to grind. Fibrous, oily, or high moisture products, like screenings, animal proteins, and grains like oats and barley, on the other hand, are very tough and require much more energy to reduce. Consequently, the hammermill setup that works well for one will not necessarily work for the other. The following discussion covers such factors as tip speeds, hammer patters and position, horsepower ratios (to hammer and screen area), and air assist systems. Little space is devoted to screen sizes (perforation or hole size) since processing variables would make any hard and fast statements nearly impossible.
Tip Speed
Tip speed, in addition to the screen size, has a significant influence on finished particle sizing. High tip speeds (>18,000 fpm) will always grind finer than lower tip speeds. Low tip speeds (<13,000 fpm), on the other hand, produce a coarser granulation with fewer fines, all other factors being equal. As a rule, smaller holed screens should only be used with higher tip speeds and large holed screens with lower tip speeds. Refer to the figures here for general guidelines for screen sizing in relation to tip speeds.
Tip speed is simply a factor of mill diameter and motor RPM and is not easily changed on direct coupled machines. There are a few v-belt drive hammermills on the market today but the time and expense involved in maintaining those machines makes them impractical for normal applications in feed manufacturing and oilseed process plants.
Tip Speed - Friable Products
For producing a uniform granulation with few fines on friable products like corn, wheat, grain sorghum, pelleted ingredients, and solvent extracted meals, an intermediate tip speed is normally desired. Hammermills with a tip speed of 13,000-18,000 fpm will produce a high quality finished product with excellent capacity and efficiency. 38” diameter mills with 1800 RPM motors (17,800 fpm) and 44” mills with 1200 or 1500 RPM motors (13,500 or 17,250 fpm) are both used extensively in the processing of all kinds of feed ingredients.
Tip Speed - Fine Grinding and Tough-to-Grind Materials
For fine grinding friable products and tough-to-grind materials, like soybean hulls, mill feed, and mixtures with animal protein products, a higher tip speed is indicated. Because more energy is required to grind these kinds of materials. more tip speed is needed to impart sufficient energy when the hammer to particle impact takes place. Normal tip speeds for fine grinding and fibrous materials are obtained on 42” and 44” mills operating at 1800 RPM (19,500 and 20,000 fpm) or 28” mills operating at 3000 RPM and 54” mills operating at 1500 RPM (21,000 fpm). Recent developments in hammermill grinding have included the use of 54” diameter mills operating at 1800 RPM. This very high tip speed (>25,000 fpm) is particularly well suited to fine grinding at high capacities and high efficiency. Because a larger screen (holes) size can be used while maintaining the fineness of grind, operating costs are reduced as well.
It should be noted while discussing tip speeds that, even though two different hammermills with different sized screens can make the same finished particle size, they will achieve those results with different efficiencies. Conversely, hammermills with different tip speeds will produce different finished products (lower speeds = coarser products) even though they are fit with the same sized screen. This is one reason it is important to include particle sizing specifications (mean particle size or % passing a test sieve) when identifying hammermill performance requirements.
Hammers
There are many hammer styles available from suppliers around the world. At the same time, there are distinctly different types of hammers used in different regions of the world. Europeans feed processors tend to favor a plain two-holed hammer with no hardfacing or edge treatment. North and South American feedmillers tend to favor a hammer with a flared hardfaced end (or ends). Each market finds a hammer type that best suits their particular needs.
As a rule, most of the variety of hammer styles that have been developed have been modified to meet a specific operational problem. In many cases, a better design of the hammermill grinding system would have eliminated the need for the “special” hammer style.
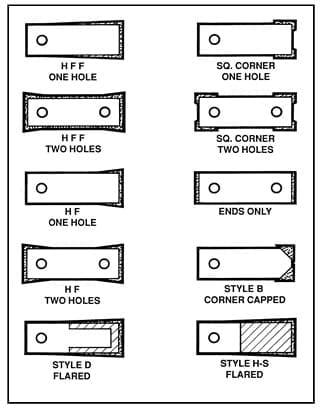
Hammer patterns and positions have a profound effect on the performance of any hammermill. Because different materials grind differently, the ideal number of hammers (pattern) and clearance to the screen (position) will need to be adjusted according to each application. At the same time, it is important to make sure the hammer pattern completely covers the working screen without having hammers trailing, that is hammers on adjacent pins in line with the preceding hammer. Complete screen coverage insures maximum process efficiency as well as controlling operating costs by getting the most out of each screen set. Trailing hammers will tend to cause accelerated wear in one area of the screen and may actually cut grooves in the screen material.
In most cases, the hammer pattern should include double hammers on the outside rows of at least two opposing pins. Because the material in the grinding chamber near the sides of the mill moves more slowly (dragging on the sides), the outside rows of hammers must do more work and are subject to more wear. Other means of dealing with this problem are also implemented by some manufacturers, including thicker, longer or even shorter hammers on the outside rows.
The hammer pattern described below depicts a typical hammer arrangement with good coverage of the screen area, no trailing hammers and double hammers on the outside rows of two opposing pins. Note that good coverage does not necessarily mean completely covering the screen with hammers but does mean distributing the hammers as uniformly as possible across the available screen area.
The hammer pattern (number of hammers used) and the position (coarse or fine) will affect the capacity of the hammermill and the quality (fineness) of the ground products. For friable products more hammers (heavier pattern) will reduce capacity and make the grind finer. Fewer hammers (lighter pattern) will increase capacity and make the grind slightly coarser and more uniform.
Many types of fibrous or tough-to- grind products will require heavier hammer patterns just to process at all. Indeed, for some very difficult to grind products the hammermill will be fit with hammers on all eight pins, with some coarse and some fine.
The graph below shows the relative affect of the hammer pattern and position on the quality (coarse and fine material produced) of corn when tested with different patterns and settings.
Hammer Patterns and Positions for Friable Products
When a relatively coarse, uniform finished product is desired, a “light” hammer pattern is selected. This means that there are fewer hammers per pin so fewer collisions will occur with particles in the grinding chamber. Light hammer patterns will demonstrate higher efficiencies than heavier patterns because less work is done. In many cases, hammermill efficiency can be improved from 5-10% simply by reducing the number of hammers used in the mill. While the grind will be slightly coarser, the difference is not noticeable without the benefit of a full sieve analysis. For maximum capacity and minimum fines, the hammers should be in the coarse position with maximum clearance between the hammers and the screen.
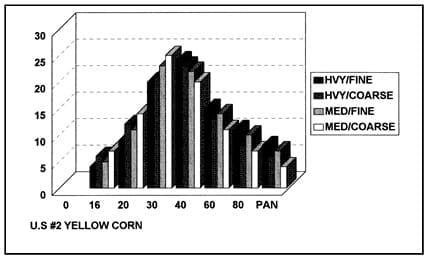
When lighter hammer patterns are employed, the horsepower per hammer ratio is also affected. For grinding friable materials in large diameter hammermills (over 36”) with 1/4” thick hammers, the ratio should be in the range of 2.5-3.5 HP/hammer, ideally about 3. For small diameter mills (up to 28”) with 1/4” thick hammers, the range is roughly 1-2 HP/hammer, with 1.5 HP/hammer ideal for mills up to 22” and 2 HP/hammer for 28” mills. Hammers will typically be mounted on four pins only when processing friable materials to a coarse, uniform finished product. This allows maximum product into the mill with minimum number of contacts being made.
Normally, hardface flared hammers will be used for the efficient reduction of friable products. Either one-hole or two-hole hammers will provide satisfactory results though good maintenance is required to be sure the two-hole hammers are turned in time to effectively use the hardfacing on both ends. It is also important to note that the second hole on two-hole hammers is exposed to the grinding operation and so is subject to some wear before it is ever used to mount the hammer to the pin.
Hammer Patterns and Position for Fibrous and Tough-to-Grind Products
As materials become tougher to grind, an increasing hammer load is employed to maximize contact between hammers and particles. Where increasing the number of hammers used to grind friable products may decrease mill capacity, increasing the number of hammers for tough-to-grind products will often improve mill capacity. In some cases, it is desirable to add hammers to all eight pins for maximum grinding efficiency and to improve screen coverage and utilization.
Because more work is done by the hammers and screens on tough-to-grind products, reducing the clearance between the hammer and screen improves grinding results. This is more true as the screen opening and grind size become smaller. The “fine position” puts the end of the hammer 3/16”-1/4” from the screen and maximizes the work done to the product. While wear to the screen and hammer is increased, the work done increases as well, making a more efficient process.
With heavier hammer patterns, the HP/hammer ratio naturally declines. For tough-to-grind materials in large diameter hammermills (over 36”) with 1/4” thick hammers, the ratio should be in the range of 1.5-2.5 HP/hammer under normal circumstances, going as low as 1:1 for particularly difficult-to-grind materials or when grinding to very fine particle sizes as in aquaculture feeds. For small diameter mills (up to 28”) with 1/4” thick hammers the ration will be roughly 1:1 (1 HP/hammer) for normal applications, going as low as 1:2 (1 HP/2 hammers) for very fine or difficult grinding. Placing hammers on all eight pins tends to reduce surging in the mill and improves screen coverage without overloading either hammer pins or rotor plates.

There is also a relationship between the HP/hammer and the wear on the hammer. Too much HP/hammer will tend to “rock” the hammer each time the hammer swings through a bed of material on the screen, leading to rapid wear of the hammer hole and hammer mounting pin. In extreme cases, the bed may be so deep that the hammer wears above the hardfacing. If this happens, the correct solution is not to use a hammer with more hardfacing extending up the side of the hammer, but to reduce the HP, increase the number of hammers, or reducing the feed rate to the mill. Too little HP/hammer dramatically reduces hammermill efficiency by consuming motor horsepower simply to turn the rotor with its load of hammers. Too little HP/hammer also tends to wear the hammers right on the corner and does not effectively use all the working surface of the hammer. In extreme cases, the rotor may actually run slow, allowing the hammers to rock, causing hammer hole and pin wear.
Screens
Hammermill screens are the highest wearing item on the hammermill, and in many cases the most obvious and seemingly expensive maintenance item. However, considering the cost of energy, hammermill screen cost per ton is quite low, and the best way to minimize the cost of hammermill operation is by frequent changing of the hammermill screens to maintain capacity, efficiency, and product quality. Depending on the material being ground and the screen hole size, one set of high quality hardfaced hammermill will normally wear out 2-4 sets of screen before the hammers require replacement. For small diameter screen holes even more frequent replacement may be required. For certain aquaculture and pet food applications it is not uncommon to replace screens with very small holes (3/64” or 1 mm and smaller) as frequently as every 8-24 hours of operation.
It is easy to see how new screens allow more product to escape, improving capacity and grinding efficiency. While thicker screens may last longer, they significantly reduce the tons/hour that a mill can process. When maintenance costs are typically $0.02-$0.04/ton and electrical costs range from about $0.25 to more than $1.00 per ton, saving money by not changing screens is not cost effective. Normally, screen material thickness will be dictated by the hole size, as it is not possible to punch a hole in material that is thicker than the diameter of the hole being punched.
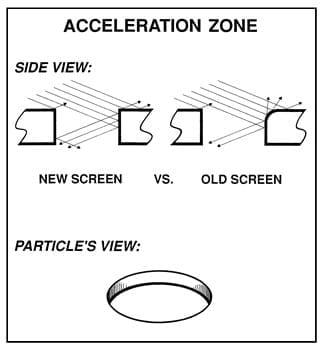
Another screen configuration problem is the amount of open area that a particular screen offers. Factors affecting open area include hole size, stagger, angle of stagger, and land dimension. Screens with fewer holes have less open area, are easier to produce and generally cost less. Screens with inline perforations as opposed to staggered hole patterns are also easier to produce and so cost less. Neither can provide good grinding efficiently and both lead to poor finished quality products because of over grinding. Screen wear is accelerated with inline perforations and screen may actually be cut by wearing the land between the holes in a very short time. Screens with little open area may wear a long time but the actual grinding cost per ton is greatly exaggerated because of the increased energy cost.
Two rules of thumb apply to hammermill screens in relation to applied horsepower:
1. Never have less than 14 In² of screen area per horsepower (more is always better).
2. Never have less than 4 In² of open area per horsepower.
Consider a typical 44” diameter by 30” wide hammermill grinding corn. A tear circle machine will have approximately 3600 In² of raw screen area. 3600 In² divided by 14 In²/HP = 250 HP maximum.
If a screen with 10/64” (4 mm) round hole perforation is used, the actual open area is roughly 36% or 3600 In² x 36% = 1296 In² of actual open area. 1296 divided by 250 HP = 5 In² open area per horsepower. This machine would grind very efficiently and produce a high quality, uniform finished meal.
If the same machine were equipped with a 4/64” (1.5 mm) round hole screen and 3/4” (20 mm) back up screen (to prevent the light gauge sizing screen from “blowing out”) for fine grinding in preparation for pelleting, or extrusion, the open area would be 3600 In² x 30% x 51% = 551 In². If the same 250 HP motor were applied, the open area per horsepower would be 551 In² / 250 HP = 2.1 In² open area per horsepower. This mill would not grind as efficiently, capacity would be reduced, and the product would be heated considerably and moisture driven off in the process.
One very simple way of increasing hammermill capacity without significantly affecting the finished grind or adding expense to the grinding system would be to replace the “up” side screen with perforations that are 2/64” to 6/64” larger than the “down” side screen. This may add 10-15% to the hammermill capacity and produce no noticeable difference in the finished products.
Feeders
Proper feeding of a hammermill is absolutely essential if the system is to operate at maximum grinding efficiency and with the lowest possible cost per ton. Uneven or inconsistent feeding can lead to surges in the motor load. This reduces capacity by causing the feed rate to be set lower than optimal in order to insure the surging load does not overload the motor. Because the load is constantly changing, the motor cannot operate at peak efficiency and so increases the grinding costs. An additional liability of surging feed that is often overlooked is the fact that surges in the feed tend to accelerate wear on the hammers and pins by causing the hammers to “rock” on the pin hammer pins.
Rotary Pocket Feeders
As the name indicates, rotary pocket feeders utilize a rotor mechanism much like a rotary airlock to evenly distribute the feed to the hammermill. In most cases, the rotor is segmented and the pockets are staggered to improve the distribution of the feed and to reduce surges in the feed rate. Because the rotary pocket type feeders rely on a free-flowing material to fill the pockets they are best suited to granular materials with a density of 35#/Ft³. or more, such as whole grains and coarsely ground meals.
Air Assist
The final application topic to be considered is the use of aspiration air to improve mill efficiency and performance. A properly designed air assist system will increase hammermill capacity by as much as 15 to 40%. The air assist system controls the environment of the grinding chamber in the hammermill and aids in moving product from the grinding chamber through the screen perforations. A properly designed air assist allows a hammermill to grind more efficiently, producing a more uniform finished product with less heating and controls dusting around the mill. Although hammermill capacity will vary with the type of machine and operational parameters, air assisted grinding systems will out produce non-assisted systems by 15-40%.
Any hammermill acts rather like a large fan, with the rotor and hammers moving air as the blades on the hub would do. Normally this “inherent air” is about 1/2 CFM per square inch of raw screen area for a modern tear circle hammermill. In order to assist the mill, an induced air flow from the inlet of the grinding chamber through the screen is required. Simply venting the discharge of the hammermill may not be adequate to relieve the pressure inside the mill since the air is being forced out in all directions, including the inlet.
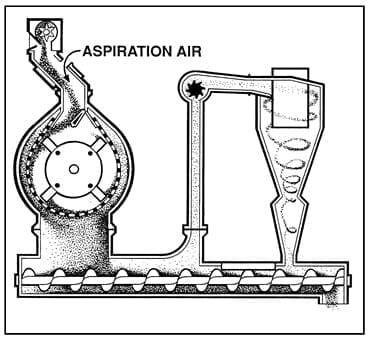
A good rule of thumb for the amount of air required to assist produce and control dusting is 1.25-1.5 CFM per square inch of screen area. Pressure drops across the mill may range from 2-5” WC, depending on system operating conditions. In order to make an air assist system work, several items must be factored, including the air flow into the mill, paths for the air and product out of the mill, separating the product from the air stream, and controlling the path of the air in the system.
To aid the product in moving through the grinding chamber and screen, the air must enter with the products being ground. If a sufficient opening for this air is not provided, the hammermill system may suffer from symptoms not unlike asthma. The velocity of the inlet air should normally not exceed 2000-2500 feet per minute (fpm).
To permit the air assist to convey product through the grinding chamber and screen there must be some place for the air to go when it discharges from the mill. Ideally, the air/product conveyor will be large enough that even when operating at full capacity, the velocity of the air will not exceed the same 2000-2500 fpm as at the inlet. If this critical path does not exist there will be a high static pressure outside the grinding chamber and the desired pressure drop across the screen may not exist.
Once the air is through the mill, it is necessary to allow the entrained fines to settle out before sending it along to the cyclone or filter system. To accomplish this, a plenum or settling chamber should be provided between the air/product conveyor and the pickup point. While in the past, such figures as “3-5 times the duct diameter” have been suggested, the bottom line is to reduce the velocity as much as possible to permit the fine material to settle out. If the plenum is designed so the air velocity drops below 15 times the bulk density (15 x 40, or 600 fpm for most feed ingredients) the separation will usually be adequate. Larger plenums will reduce the velocity further and improve the air/fines separation. For practical purposes, the plenum cannot be too large.
To make the air assist system work, it is necessary to control the path the air takes through the hammermill. Normally, the discharge end of the take away conveyor must include some kind of airlock to insure the air is pulled through the hammermill instead of back through the discharge system. This may be as simple as a shroud over the take away screw or as complex as a powered rotary airlock at the discharge of the drag conveyor.
Other Considerations
Not specifically addressed so far in this discussion is the need to provide a relatively clean feed stream to the hammermill grinder no matter what is being processed. Foreign material such as dirt, stones, and other mineral impurities greatly accelerate wear of the working components.
Large stones and pieces of non-magnetic metals can not only damage hammermill screens but can cause a set of hammers to go out of balance or even fail catastrophically. This is not only expensive but can also expose personnel in the area to danger.
Magnetic protection is necessary in order to realize the best life of the working components of the mill. Errant tramp iron that enters a hammermill can knock holes in the screen, break hammers, and create undesirable sources of ignition. Always buy the best possible magnetic protection that is reasonable for a specific system and make sure the magnets are routinely cleaned. Nothing in the process plant is less productive than magnets that are covered with tramp iron.
Hammermills should all have a vibration monitor switch to shut down the hammermill in the event excessive vibration is encountered.
Perhaps the most important factor to keep in mind regarding hammermill operating costs, is that the energy required is typically 5 to 10 times more expensive than the cost of maintenance parts (screens and hammers). Trying to economize on hammermill operation by using screens and hammers beyond a reasonable
The Economics of Grinding
As noted previously, both roller mills and hammermills are used to grind common feed materials in preparation for pelleting and other extrusion processes. Whether to use a hammermill or a roller mill will depend on a variety of factors including but not limited to: the material(s) to be ground, energy costs, type of feed(s) produced, and level of automation required.
Materials Roller mills work best on relatively easy to grind materials like corn, grain sorghum, wheat, and soybean meal. A roller mill can effectively be used to produce a coarse, uniform cracked corn and grind corn down to a finished particle size around 600-700µ mean particle size. Roller mills are not particularly effective on rations that are high protein, high fat, or contain a lot of fibrous materials.
Hammermills can process a wide range of products but need to be properly equipped for the specific task at hand. If a hammermill will be used to process a wide range of materials and finished particle size, it may be necessary to equip the main driving motor with an inverter (Variable Frequency Drive) to allow the tip speed to be adjusted according to the materials being processed and finished particle size required. Hammermill can easily be set up to grind corn to 500, 400, or even 300µ mean particle size or smaller if needed.
Energy Cost In a typical feed milling application grinding corn, a roller mill will produce anywhere from 15 to 85% more tons per hour than a hammermill depending on the finished particle size. As energy costs ($/kWh) increase, the difference between the roller mill and hammermill are even more considerable. For the following examples, an energy cost of $0.05/ kWh has been used to compare the cost difference between grinding with a roller mill or grinding with a hammermill. As energy prices move higher or lower, the actual grinding costs will change as well.
Type of Feed(s) It has been well documented that swine diets utilizing roller mill ground corn provide a positive effect in terms of feed conversion, even when the diets are pelleted. In a case such as this the roller mill could easily be applied to the task of grinding for pelleted feeds. On the other hand, certain poultry rations such as ducks or turkeys require a very high quality pellet in order to allow the animal to consume the feed effectively. Hammermill grinding may be indicated, particularly if the diet contains ingredients that are not well suited to roller mill grinding.
Automation Both roller mills and hammermills can be equipped with rotary pocket feeders, making automation of feed rate control very simple using conventional inverter (Variable Frequency Drive) technology. By constantly monitoring and adjusting the feed rate to maintain a predetermined motor load, both the throughput of the grinding equipment, as well as the efficiency are maximized. Since most hammermills operate with a fixed rotor speed (tip speed) the only variable in processing is the feed rate and so automation is really quite simple and straight forward. Roller mills do require some adjustment of the roll gap to take into account processing variables such as grain moisture or physical conditions, and to accommodate the normal wear of the roll corrugations. For this reason, automation of roller mills is more complicated than hammermills, and final roll adjustment may still require examining the finished ground product and additional roll adjustment to insure the finished product is within process specifications.
Equipment and Operating Costs
It is commonly assumed that roller mills are more expensive than hammermills, and in the most basic comparison this would be true. Considering that a roller mill typically contains significantly more material (roller mills may weigh 2 to 5 time more than hammermills on a “per capacity” basis ) and contain 4 times as many bearings plus v-belt drives and roll adjusting mechanisms, it would be unreasonable to expect any else. However, when the full grinding packages are considered, including feeders, motors, starters, and air assist systems required for hammermills to attain their peak efficiency, there may be no difference in the initial cost of the machine. In many cases, a roller mill system complete will be less expensive than a hammermill system to achieve the same capacity.
Maintenance cost for hammermills will generally be quite low, often $0.02 to $0.03 per ton for the screens and hammers when grinding corn between 500 and 1000µ mean particle size. In most cases, wear parts for hammermills are available from a variety of sources, keeping the costs very competitive. Routine hammermill maintenance does not require highly skilled technicians, and even a full hammer change on the largest machines can be completed in 1-2 hours with a little planning and organization. Screen changes may require 10-15 minutes, and often it takes longer for the hammermill to coast to a stop than the time actually required to change out the screens. Other maintenance items on the hammermill are generally more long term, perhaps 2-5 years before the regrind chamber or inlet flow directors must be replaced, and often 5-7 years or more for the wear liners in the hammermill. Overall, the total cost of all wear parts used on the hammermill will normally be less than $0.05/ton for hammermills used in typical feed milling applications.
Roller mill maintenance is more expensive (per ton) and will require certain specialized resources for roll recorrugation. Normal down time for a roll change will be 4-8 hours, and the frequency depends entirely on the number of tons processed, as well as the quality and cleanliness of materials being ground. Since the frequency of recorrugation is less than that of hammermill maintenance the actual maintenance hours per ton are quite comparable to hammermill operations. Most roller mills producing corn ground to around 700µ meal particle size will operate between 1,500 and 2,000 hours before the rolls require recorrugation. Changing rolls will require a reasonably well trained technician to insure the rolls are properly aligned and that the drives are correctly adjusted. For most operations, maintenance costs for roller mill grinding will be in the range of $0.05 to $0.07 per ton. Even so, due to the lower energy cost per ton, the overall operating cost for a roller mill will be significantly lower than hammermills, making them an attractive alternative for many feed milling applications.
Consider the equipment and operating cost comparisons for the three corn grinding scenarios identified below:
In this first example corn is being ground to around 1200µ mean particle size. While this is rather coarse for many rations, it would represent the particle size deemed best for feeds such as laying hens or sow rations. In this case, the equipment cost of the roller mill system is slightly less than the hammermill system. The higher installed cost of the hammermill is due primarily due to the added cost of the air assist package required to insure the hammermill operates at peak capacity and efficiency. The lower installed cost combined with the lower operating cost of the roller mill is makes the roller mill very attractive in terms of long term or “life cycle” cost.
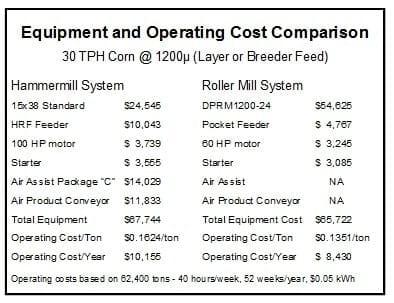
The equipment cost is rather straightforward and easy to determine in the case of a new machine installation. Energy costs can also be calculated relatively easily, since there is a distinct relationship between HP and kW, 1 HP = .746 kW, and electrical rates are normally described in terms of cost per kWh (kilowatt hour). Keep in mind that with the base charge for electrical energy, many industrial customers are charged an additional “demand charge” for even relatively short periods of relatively high usage. In some cases, starting a large motor will cause a surge in usage that can trigger a demand charge. On the other hand, some industrial customers will be offered attractive “off peak” rates where the cost per kWh is reduced for example during overnight operations when electrical consumption is generally lower.
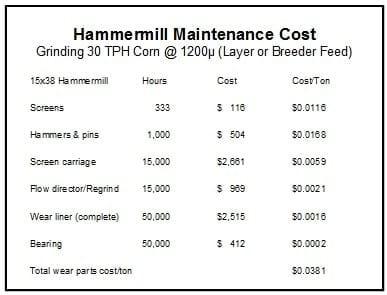
In the examples used here, flat rate of $0.05/kWh was used with no attempt to take into account demand charges, off peak rates, or other electrical rate adjustments. For the hammermill, 100 HP = 74.6 kW x $0.05 kWh = $3.730 /hour to run the motor; $3.730 / 30 TPH = $0.1243/ton for electrical energy.
For the roller mill, 60 HP = 44.76 kW x $0.05 kWh = $2.238 / hour to run the motor; $2.238 / 30 TPH = $$0.0746 / ton for electrical energy.
Maintenance costs for the hammermill include both the “routine” maintenance items including the screens, hammers, and pins as well as longer term maintenance items that will, eventually have to be replaced. As noted previously, one characteristic of a well designed modern hammermill is that everything that is subject to wear is replaceable. As a results, many hammermills can remain in service for 10, 15, or even 20 years and still perform with the same capacity and efficiency as new machines, since in fact all of the working parts will be maintained in like new condition.
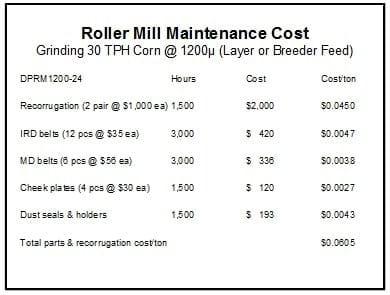
For the roller mill, normal maintenance items include roll recorrugation and regular maintenance parts such as belts, dust seals, and cheek plates (roll end seals). As might be expected, the maintenance cost, per ton, for the roller mill is higher than maintenance parts costs for the hammermill. However, when the energy cost is factored in, the roller mill in this case is 18 to 20% lower cost per ton when processing corn to 1200µ.
In the second example shown here, corn is being ground to about 700µ mean particle size. This would be quite typical for pelleted swine feeds, as well as for many broiler feed applications.
As in the first example, the cost of the roller mill system used to grind corn to 700µ is less than the cost of a hammermill system of equal capacity though in this example the difference is remarkably greater. With the suitable air assist system included, the hammermill system cost is roughly 30% higher than the roller mill system of equal capacity. The grinding cost per ton for the hammermill is more than 25% higher than the roller mill. Again when considering the long term cost of both grinding systems, the roller mill offers a potential cost savings of more than $100,000 if the system is operated for only 10 years.
Maintenance costs for the hammermill and roller mills used are detailed here and as in the first example include all of the normal wearing parts that would be required for long term maintenance of both the hammermill and roller mill.
It is interesting to note that even though the roller mill maintenance costs in this instance are relatively high ($0.0935/ton) the big difference in energy cost per ton still favors the roller mill for cost per ton of ground corn.
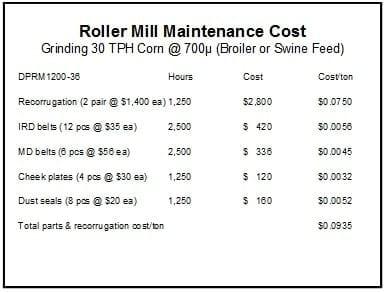
For many customers today, the desire is to produce a finer finished grind, either for improved pellet quality or for improved feed conversion. In swine diets especially, there seems to be a positive response between reducing particle size and increasing animal performance. The in final example described here, both roller mills and hammermill are used to reduce the size of corn to a mean particle size of 500µ. To achieve this relatively fine finished product while maintaining a high throughput (30 TPH in this case) both the roller mill and hammermill increase in size and connected HP. Since the roller mill in this example is significantly larger than the two previous scenarios, the cost of the machine increase quite dramatically. In fact, the installed cost of the roller mill will be greater than a hammermill of equal capacity, even taking into account the higher cost of larger motors, starters, and the air assist system needed to maintain peak operating efficiency.
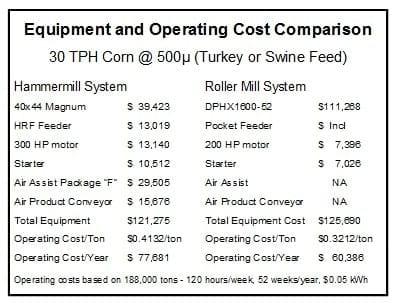
As in the other examples, the cost per ton of operating the roller mill system is less than a hammermill of comparable capacity considering energy and maintenance costs. In fact, the roller mill operating cost of $0.3212 / ton is 23% less than the hammermill cost per ton of $0.4132. The lower operating cost of the roller mill would pay back the difference in initial cost in about 50,000 tons or about 1,600 hours. In this example of grinding 188,000 tons per year the roller mill system payback is reached in just over three months of operation.
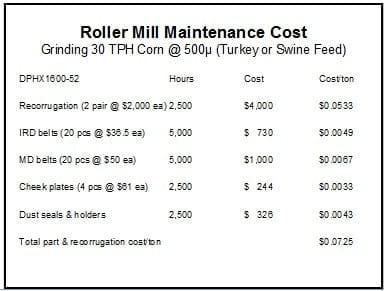
In many plants today, there is a need or desire to increase the grinding capacity of existing hammermills, or to maintain the current capacity while reducing the particle size of the finished materials being ground. One relatively simple way to achieve this would be to install a single pair roller mill ahead of the hammermill to reduce the particle size being introduced into the hammermill grinder. This technique has been applied to pet food plants, feed mills, and ethanol plants all of which require a fine ground product for their subsequent processing. It has been noted in some instances that the roller mill “pre-grind” of corn, rations containing a high percentage of coarse materials (including corn, oilseed meals, or pelleted ingredients) can increase the hammermill capacity from 25% to as much as 50%. At the same time, the wearing parts of the hammermill including the screens and hammers benefit from a significant increase in useful life. Reports of double or even triple the useful life are not uncommon.
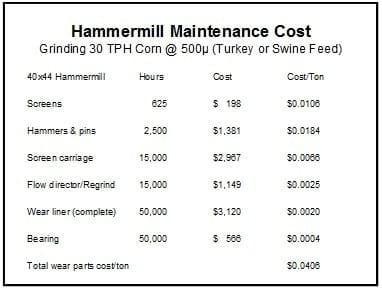
As seen in this example, to achieve the same capacity and particle size, a combined “step grinding” system offers both a lower installed cost and lower operating cost than either a hammermill system or roller mill system alone. While it is true that the combined system does require more sophisticated controls to obtain the maximum capacity and efficiency, the low operating cost means this approach to grinding cannot be ignored.