Introduction
Poultry meat being a high quality animal protein source plays significant role in maintaining the health and nutrition of the people (Shahzad et al., 2011). Along with broilers, 35.4 million spent birds (27.4 million layers and 8.0 million breeders) are also being used at the end of egg production cycle contributing more than 46,000 metric tones of poultry meat per annum in Pakistan (Anonymous, 2009; Mahmud et al., 2011). However, with the introduction and adoption of induced molting technique by most of the poultry farmers (Akram and Rehman, 1998), spent bird’s meat is available after two production cycles. Different methods have been used to induce molting but feed deprivation with or without water and light restriction remains the most widely utilized method because it is simple, practicable and economical (Yousaf and Ahmed, 2006).
During post fast stage of molt, various feeding regimens like 12.4, 13.5, 14.8 or 17.0% crude protein (CP) molt diet (Hoyle and Garlich, 1987), corn supplemented with corn gluten feed, and spent hen meal (Koelkebeck et al., 2001), corn, 14.0, 16.0 or 18.0 CP with 2700 and 2900 kcal/kg metabolizable energy (ME) molt diets (Zia et al., 2000), use of probiotics with restricted feeding (Khajali et al., 2008), use of calcium in low energy diet (Dickey et al., 2010) and use of distillers dried grains with soluble (Mejia et al., 2011) have been recommended to induce molt. However, the optimal levels of CP and ME in molt diet, during post fast phase of molt, are still controversial.
In most of the experiments, the effect of different molt diets on egg quality parameters have been studied extensively (Koelkebeck et al., 2001; Yousaf and Ahmed, 2006; Khajali et al., 2008; Mejia et al., 2011), whereas its effect on meat quality of the bird has been neglected despite realizing the contribution of spent birds in total poultry meat production. In some of the studies conducted so far, changes in meat composition have been observed during molt induction process and at different stages of post molt production cycle (Akhtar, 1996; Akram, 1998; Zia et al., 2000). During molting period, birds mobilized body fat which reduced to minimum at post fast phase (Zia et al., 2000). Dry matter (DM), EE and ash contents of breast and thigh meat reduced to minimum at early post molt stage. However, these contents were increased at peak production stage and were found maximum at pre molt stage (Akhtar, 1996). But protein content of the meat increased to maximum at post fast stage and then decreased gradually (Akram, 1998). Tang et al. (2007) noticed significant interaction of dietary ME and lysine on weight and yield of breast muscle and were of the opinion that different concentrations of dietary ME and lysine might be considered to improve meat quality.
Although various nutritional regimens have been adopted during induced molt but the information regarding effects of varying CP and ME levels in molt diet on meat composition of the hens was scanty. Therefore, present study was undertaken to determine the impact of different CP and ME levels of molt diets on proximate composition of breast and thigh meat of molted layers.
Materials and methods
One hundred and fifty four White Leghorn Single Comb (WLHSC) hens of a commercial strain (Euribrid), 70 weeks old having uniform body weight (1.48±0.03 kg), were housed in individual cages at Poultry Research Farm, University of Agriculture, Faisalabad. The study was conducted during the winter and spring seasons (from October to April) when the stress of heat was minimum. These hens were given 7 days for adjustment period. During this period hens were provided ad libitum layer ration and water with 24 hours light and were dewormed, vaccinated against New Castle Disease and given antibiotics to prevent them from secondary infection. At the end of adjustment period 5 birds were randomly selected, slaughtered and dressed by defeathering through warm water scalding. The meat samples were then drawn from both sides of breast and thigh regions and were pooled separately. The samples were saved in plastic bags, labeled and stored in freezer for chemical analysis. The remaining hens were induced to molt by feed deprivation along with reduction of photoperiod from 24 hours light to 6 hours light (Akram and Rehman, 1998). After 10 days of fasting, 5 hens were randomly slaughtered and meat samples were stored as previously described. The remaining 144 hens were allocated at random in 18 experimental units of 8 hens each. Six experimental molt diets, mentioned below, having 3 levels of protein (14, 16 and 18%) and 2 levels of energy (2700 and 2900 kcal/kg) were formulated.
1. Low Protein-Low Energy (LPLE) diet having 14% CP and 2700 kcal/kg ME
2. Low Protein-High Energy (LPHE) diet having 14% CP and 2900 kcal/kg ME
3. Medium Protein-Low Energy (MPLE) diet having 16% CP and 2700 kcal/kg ME
4. Medium Protein-High Energy (MPHE) diet having 16% CP and 2900 kcal/kg ME
5. High Protein-Low Energy (HPLE) diet having 18% CP and 2700 kcal/kg ME
6. High Protein-High Energy (HPHE) diet having 18% CP and 2900 kcal/kg ME
The ingredients and chemical composition of these diets is presented in Table 1. These diets were randomly allotted to the hens in such a way that each diet received 3 experimental units of 8 hens each. These diets were offered at the rate of 45g/bird for 25 days with same lighting pattern as was in fasting. After the completion of molting period hens were offered ad libitum layer ration containing CP 17% and ME 2900 kcal/kg and were provided natural day light which was then increased half an hour weekly till 16 hours during the remaining period (24 weeks of egg production). Two hens per replicate at post-molt, at 50% egg production of total hens and at the end of experiment were randomly selected, slaughtered and meat samples were stored.
Table 1: Composition of experimental molt diets and layer ration
The frozen breast and thigh meat samples were thawed, chopped into small pieces and then ground in a meat grinder using a die with 0.5 cm diameter holes. A part of each sample was dried by mixing with anhydrous sodium sulfate in 1:4 ratios, ground in pestle mortar and then used for proximate analysis. The remaining of each sample was used for determination of moisture content. Proximate composition (moisture, protein, ether extract and ash) of meat samples was determined by methods of AOAC (1990). The data were subjected to analysis of variance technique with a 3 (protein levels) × 2 (Energy levels) factorial arrangement of the treatments using the General Linear Model (GLM) procedure of SAS (2000). Significance of differences among means was determined by applying LSD test. Statements of statistical significance are based on P<0.05.
Results and discussion
Results regarding proximate composition of breast and thigh meat as affected by various protein and energy levels of molt diets are presented in Table 2. There were significant (P<0.05) differences in moisture, protein and EE contents of breast meat, and moisture and EE contents of thigh meat with respect to various dietary protein levels. Whereas, the effect of different energy levels was found to be significant (P<0.05) only on ash content of thigh meat. Unfortunately, the linear and quadratic effects of protein levels on the response variables could not be observed in the study.
Table 2: Proximate composition (%) of breast and thigh meat of White Leghorn layers as affected by different levels of protein and energy in molt Diets
Moisture content:
Maximum moisture content of breast (72.8%) and thigh (72.0%) meat at post molt stage were recorded in birds fed 18% protein diet and minimum moisture content of breast (71.7%) and thigh (71.5%) meat were noticed in birds fed 14% protein diet reflecting proportionate increase in meat moisture content with increase in dietary protein. Similar trend were also observed at 50% egg production and at the end of trial. Results of our study are in line with the findings of Mark and Pesti (1984) who observed an increase in water intake in the birds fed on high protein diet. Our results are also in agreement with Rosebrough et al. (1999) who reported an increase in moisture percentage of carcass of birds fed on high protein diet with the argument that high protein diet reduces lipogenesis in the liver and leads to the reduction of fat deposition in muscles and therefore, decrease the overall dry matter percentage of meat. Higher moisture content in carcasses of birds fed high protein diet compared to the birds fed low protein diet has also been observed in other studies (Ferket and Sell, 1990). However, non-significant differences were reported by Results regarding effect of dietary protein and energy interactions on moisture content of meat at post molt stage showed significantly higher moisture content (73.0 and 72.1%) with HPLE diet whereas, significantly lower values of moisture (71.6 and 71.4%) were noticed with LPHE diet in breast and thigh meat, respectively. Similar trend was also seen at 50% egg production and at the end of trial. However, the difference between LPHE and MPHE diets was non-significant. Our results also co-relate with the findings of Ferket and Sell (1990) who reported significantly higher moisture content of meat in birds fed high protein-low energy diet and lower moisture content with low protein-high energy diet combinations.
The proximate composition of breast and thigh meat at various stages of molt and production indicates significant (P<0.05) differences in moisture content (Fig. 1a) at different stages. Moisture percentages of both breast and thigh meat were higher at post fast stage compared to pre molt stage however, it decreased with increase in age and was found lowest at the end of experiment (24 weeks of production). Fasting during molting resulted in depletion of body reserves like fat, protein and ash contents reflecting decrease in dry matter, hence, moisture percentage of meat increased. Significant increase in moisture percentage of hens as a result of fasting during moulting and then decreasing trend has also been reported by Hoyle and Garlich (1987) and Akram (1998).
Protein contents:
Protein content of breast meat was significantly (P<0.05) affected by different dietary CP levels during molt and production stages. However, effect of dietary energy levels on protein content of both breast and thigh meat was non-significant. Significant increase in protein contents of breast meat limited to 16% CP diet as compared to 14% CP diet reflected increase in muscle protein with increase in dietary protein due to more availability of the nutrients. However, the difference in protein content of meat on 16 and 18% CP diets was nonsignificant. This was probably due to the fact that the optimum amount of protein consumed by the birds was deposited in the breast muscles more efficiently than the extra amount consumed by the birds fed on higher level of protein. Auckland and Morris (1971) observed the same response in birds fed 19.8 vs 28.9% protein diets and hypothesized that this response was a consequence of diminishing efficiency of protein deposition as protein level increased. Naulia and Singh (2002) in their study recommended that dietary level of 16% CP may be adequate for laying hens and increase over this level (up to 18%) leads to wastage of protein. Dietary protein and energy interaction (Fig. 1b) showed significantly (P<0.05) higher protein content in breast meat of birds fed MPHE and HPHE diets. Results regarding main effects of dietary protein, discussed earlier, indicate higher protein contents of breast meat with 16 and 18% CP diet, whereas, different dietary energy level could not show any influence upon protein content hence, protein content of breast meat was higher with MPHE and HPHE diets compared to other diets. Breast muscle occupies the largest proportion of body mass relative to all other individual body parts, and its growth accounts for a large proportion of the body protein gains. Hence breast meat contains more protein content than thigh (Ferket and Sell, 1990). In the present study, protein content of breast meat was also found to be higher as compared to thigh meat.
Non-significant changes in protein content of thigh meat with respect to various dietary treatments were observed probably due to lesser protein content of thigh meat.
Among stages, maximum protein content of breast and thigh meat was recorded at post fast stage and minimum protein content at post molt stage (Fig. 1b). However, after post molt stage there was significant increase in protein content of breast and thigh meat at 50% egg production stage as well as at the end of trial. This highest protein content in both breast and thigh meat at post fast stage was due to depletion of fat and ash contents of meat as a result of fasting. With the resumption of restricted feeding after fasting there was increase in mostly the fat content of the meat that resulted in decreased percent protein of the meat at post molt stage. Then with the provision of ad libitum layer ration after molting there was increase in protein percentage of breast and thigh meat due to more protein availability from the feed. Our results are also supported by the findings of Hoyle and Garlich (1987) and Akram (1998) who also observed significant increase of protein percentage of breast and thigh meat at post fast stage compared to post molt stage.
Ether Extract content:
The differences among ether extract (EE) content of breast and thigh meat as affected by different dietary protein levels and at various molt and production stages were statistically significant (P<0.05). However, the effect of dietary energy levels on EE content was non-significant. Diet containing 14% CP resulted in higher EE percentages of breast and thigh meat whereas, lower values of EE content were observed with 18% CP diet indicating an inverse trend between EE content of the meat and dietary protein levels. Rama Rao et al. (2004) also found an inverse relationship between the levels of dietary protein and EE content of the meat and speculated that an increase in dietary protein level decreased the flow of substrate (acetic acid) through glycolysis and increase the production of glucose from substrates that were formally in the pathways leading to fat synthesis. Effect of dietary protein and energy interaction on EE content showed significantly (P<0.05) higher EE content of thigh meat in birds fed on LPHE diet and significantly (P<0.05) lower EE content with HPLE and MPHE diets. It reflected that diet having low protein with higher calorie: protein ratio resulted in more EE content in meat as compared to high protein diet with lower calorie: protein ratio. These results are also supported by the findings of Edwards and Denman (1975) who reported that low protein diet with wider calorie: protein ratio produced birds with more carcass fat than those of high protein with narrow calorie: protein ratio.
Among stages, maximum EE content (2.34 and 3.93%) was observed at pre molt stage whereas minimum EE content (1.90 and 3.21%) was noticed at post fast stage in breast and thigh meat, respectively (Fig. 1c). This drastic decrease in EE content at post fast stage was due to the utilization of body fat to provide energy to the bird during fasting. However after fasting, with the provision of restricted dietary treatments, there was increase in EE content at post molt stage. And thereafter, due to resumption of ad libitum feeding during production period there was increasing trend in EE content of breast and thigh meat at 50% egg production stage and even at the end of trail due to more availability of energy. Significant decrease in EE content of breast and thigh meat after fasting and then increasing trend after moulting and at the end of post molt production cycle was also reported in other studies (Akhtar, 1996; Akram, 1998).
Ash content:
Neither the protein nor the energy levels of molt diets individually or in combination with each other resulted in any significant change in ash content of breast meat. However, in case of thigh meat, 2700 kcal/kg ME diet produced significantly higher ash content (1.47%) at post molt stage, as compared to the ash content (1.35%) produced by 2900 kcal/kg ME diet. Our results are supported by the findings of Simpson and Ferket (1979) who observed that feeding a high energy ration resulted in decrease in ash content of thigh meat but not in breast meat probably due to more ash content of thigh meat vs. breast meat. However, the difference became non significant at later stages of production. Among stages, ash content followed the pattern similar to EE i.e. significantly (P<0.05) lower values (1.28 and 1.33%) in breast and thigh meat, respectively after fasting as a result of depletion of ash along with other meat components (Fig. 1d). Then with the resumption of feeding, after fasting, ash content again increased. These results are in line with the findings of Akram (1998) who also reported a decrease in ash content after fasting and then increasing trend thereafter.
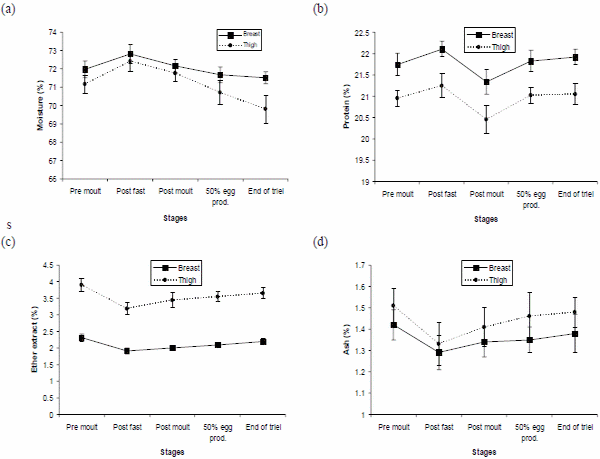
Fig. 1: Proximate composition (%) of breast and thigh meat (a, b, c & d) of Leghorn layers at various molt and post molt production stages.
In this study, the difference of 1 or even <1% among some of the values of variables has been observed significant. Percentage wise these differences look small. But in the developing countries like Pakistan where huge quantity (46,000 metric tons) of spent birds meat is consumed annually, the significant difference of <1% in moisture or CP values of the meat becomes very important in nutritional point of view.
Conclusions:
Dietary level of 16% CP may be adequate for hens during molt process and increase over this level (up to 18%) leads to wastage of protein. Molt diet (MPHE) having 16% CP and 2900 kcal/kg ME can be used more efficiently as this diet produced more DM and protein content with less EE in meat.
This article was originally published in Pakistan Veterinary Journal, ISSN: 0253-8318 (PRINT), 2074-7764 (ONLINE).
References
Akhtar RS, 1996. Effect of second molt on productive performance, carcass characteristics and meat composition during third production cycle of commercial layers. MSc Thesis, Univ Agric, Faisalabad, Pakistan.
Akram M, 1998. Effect of induced molt on the subsequent second production cycle performance of commercial layers reared under various lighting and feeding regimes. PhD Thesis, Univ Agric, Faisalabad, Pakistan.
Akram M and Zia-ur-Rehman, 1998. Reconditioning of 50,000 spent commercial layers. Proc 8th World Conference on Animal Production. Seoul, South Korea, June 28-30, 1998, pp: 716-717.
Anonymous, 2009. Economic Survey 2008-2009. Government of Pakistan, Economic Advisor’s Wing, Finance Division, Islamabad.
AOAC, 1990. Official Methods of Analysis. 15th Ed, Association of Official Analytical Chemists, Arlington, Virginia, USA.
Auckland JN and TR Morris, 1971. Compensatory growth after under nutrition in market turkeys: effect of low protein feeding and realimentation on body composition. Br Poult Sci, 12: 137-150
Dickey ER, K Bregendahl, K Stalder, R Fitzgerald and AK Johnson, 2010. Effects of a pre molt calcium and low-energy program on laying hen behavior and heterophil to lymphocyte ratio. Poult Sci, 89: 2317-2325.
Edwards HM Jr and F Denman, 1975. Carcass composition studies. 2. Influences of breed, sex and diet on gross composition of the carcass and fatty acid composition of adipose tissues. Poult Sci, 54: 1230-1238.
Ferket PR and JL Sell, 1990. Effect of early protein and energy restriction of large turkey tomes fed high or low fat realimentation diets. 2. Carcass characteristics. Poult Sci, 69: 1982-1990.
Hoyle CM and JD Garlich, 1987. Post fasting dietary protein requirements of induced molt hens. Poult Sci, 66: 1973-1979.
Khajali F, S Karimi and D Qujeq, 2008. Probiotics in drinking water alleviate stress of induced molting in feed-deprived laying hens. Asian-Aust J Anim Sci, 21: 1196-1200.
Koelkebeck KW, CM Parsons, MW Douglas, RW Leeper, S Jin, X Wang, Y Zhang and S Fernandez, 2001. Early post molt performance of laying hens fed a low-protein corn molt diet supplemented with spent hen meal. Poult Sci, 80: 353-357.
Mahmud A, Saima, Rafiullah and I Ali, 2011. Effect of different light regimens on performance of broilers. J Anim Plant Sci, 21: 104- 106.
Mark HL and M Pesti, 1984. The role of protein level and diet form in water consumption and abdominal fat pad deposition of broiler. Poult Sci, 63: 1617-1623.
Mejia L, ET Meyer, DL Studer, PL Utterback, CW Utterback, CM Parsons and KW Koelkebeck, 2011. Evaluation of limit feeding varying levels of distillers dried grains with solubles in non-feedwithdrawal molt programs for laying hens. Poult Sci, 90: 321-327.
Naulia U and KS Singh, 2002. Effect of substitution of groundnut meal with soybean meal at varying fish meal and protein levels on performance and egg quality of layer chickens. Asian-Aust J Anim Sci, 15: 1617-1621.
Rama Rao SV, MVLN Raju, MR Reddy and AK Panda, 2004. Replacement of yellow maize with pearl millet (Pennisetum typhoides), foxtail millet (Setaria Italia) or finger millet (Eleusine coracana) in broiler chicken diets containing supplemented enzymes. Asian-Aust J Anim Sci, 17: 836-842.
Rosebrough RW, JP McMurtry and R Vasilatos-Younken, 1999. Dietary fat and protein interactions in the broiler. Poult Sci, 78: 992-998.
SAS, 2000. SAS User’s Guide: Statistics. Version 8th Ed SAS institute Inc, Cary, NC, USA.
Shahzad M, F Rizvi, A Khan, M Siddique, MZ Khan and SM Bukhari, 2011. Diagnosis of avain paramyxovirus type-1 infection in chicken by immunoflourescence technique. Int J Agric Biol, 13: 266–270.
Simpson MD and PR Ferket, 1979. Chemical composition and yield of Cornish game hens. Poult Sci, 58: 1400-1402.
Tang MY, OG Ma, XD Chen and C Ji, 2007. Effects of dietary metabolizable energy and lysine on carcass characteristics and meat quality in Arbor Acres broilers. Asian-Aust J Anim Sci, 20: 1865-1873.
Yousaf M and N Ahmed, 2006. Effects of housing systems on productive performance of commercial layers following induced molting by aluminum oxide supplementation. Pak Vet J, 26: 101-104.
Zia-ul-Hasan, JI Sultan and M Akram, 2000. Nutritional manipulations during induced molt in white leghorn layers: Effects on carcass characteristics and visceral organs. Int J Agric Biol, 2: 214-218.