Raw Materials
Traditionally the most popular raw material for production of textured vegetable proteins in an extrusion system has been slightly toasted defatted soy flour. This defatted soy flour usually meets the following characteristics: 50% protein minimum, 3.5% fiber maximum, 1.5% fat maximum and PDI of 60 to 70.
Soy flour with these specifications allows controllable production of textured proteins in chunk and extended form on single screw extrusion cookers. Other vegetable protein sources also have been used as raw materials for texturizing, and these include: glandless cottonseed flour, rape seed or canola concentrates, defatted peanut flour, defatted sesame flour, as well as soybean grits, flakes, meal, concentrates and isolates.
The use and development of twin screw extrusion cookers in the field of texturized proteins has increased the raw material specification range to include raw materials that include: PDI ranges from 20 to 70, fat levels from 0.5 to 6.5%, fiber levels up to 6% and particle sizes up to 8 mesh.
Protein dispersibility index. The Protein Dispersibility Index (PDI) is the percentage of total protein that is dispersible in water under controlled conditions of extraction. The PDI is now the preferred measurement with regard to specification of raw ingredients, as it is a more reliable figure when compared with NSI. Textured soy products have been produced with raw materials ranging from 20 to 70 PDI.
Fat level. Raw materials have been texturized containing 0.5 to 6.5% fat levels. This higher range of fat (5.5%) allows mechanically extracted soybean meal to be texturized into meat extenders and meat analogs. When extruding material with higher fat levels, generally it can be said that increased shear energy input and higher temperatures are required to maintain a desired product integrity.
Fiber level. The presence of fiber in extruded soy proteins inhibits or blocks the interaction or cross-linking of protein molecules necessary for good textural integrity. Changing the extruder configuration to impart more shearing action into soy proteins containing higher levels of fiber may achieve a final product with textural properties similar to soy protein with lower levels of fiber.
Particle size. With regard to successful production using single screw extrusion cookers, the exact limitations of particle size requirements of defatted soy flour have never been determined and the range is very wide. The only limitations encountered are as follows: Very fine flour, below 400 mesh (38 micron), should be limited because of problems in wetting this very fine powder without lumping; also, very coarse product, over 80 mesh (180 micron), requires complicated pre- moistening, and sometimes, whole granules are seen in the finished product. We, therefore, recommend a product grind of 95% through 100 mesh (150 micron), with a maximum of 50% through 325 mesh (45 micron) for defatted soy flour.
Twin screw extruders can, sometimes use raw material with a particle size range up to 8 mesh (2360 micron) without affecting the textural properties of the final product.
It is also believed that proper preconditioning has a great effect on the ability of the extrusion cookers to utilize the larger particle size raw materials for textured protein production. Preconditioning is a time, temperature and moisture level relationship and controlling variables allows for the soy flour or grits to be evenly pre-moistened and pre-tempered.
Protein levels. The percentage of protein is normally inversely related to the remaining constituents of a raw material such as fat and fiber. For example, soybean protein content goes up as the oil and hulls are removed. Therefore, as a protein level of a raw material decreases, the textural integrity and water holding capacity decrease and the bulk density of the final products increases.
Adjustments in pH. Increasing the pH of vegetable proteins before or during the extrusion process will aid in texturization of the protein. Extreme increases in pH will increase the solubility and decrease the final product (1) Modifying pH above 8.0 also may result in the production of harmful lysinoalanines; (2) Lowering the pH has the opposite effect and will decrease protein solubility, making the protein more difficult to process. Undesirable sour flavors in the texturized vegetable protein products may be evident, if the pH is adjusted below 5.0.
Modifying the pH to the alkaline side will increase the water absorption. This is generally done by using calcium hydroxide or the more widely used sodium hydroxide at about 0.1% or as required.
Calcium chloride. Calcium chloride (CaCl2) is very effective in increasing the textural integrity of extruded vegetable proteins and also aids in smoothing its surface. Dosing levels for CaCl2 range between 0.5 and 2.0%. With the addition of CaCl2 and small amounts of sulfur, soybean meal containing 7.0% fiber may be texturized, retorted for one hour at 110°C and still maintain a strong meat- like texture. Sodium chloride (NaC1) does not appear to add any benefit to the texture of extruded vegetable proteins. In fact, it tends to weaken the textural strength.
The addition of sodium alginate will increase chewiness, water- holding capacity and density of extruded protein products. Sugar will also disrupt the textural development of soy proteins (3).
Soy lecithin. When added to formulations of vegetable proteins at levels up to 0.4%, lecithin tends to assist smooth laminar flow in the extruder barrel and die configuration, which allows the production of increased density soy products. The ability to make dense vegetable protein products is related to the higher degree of cross-linking that occurs during the extrusion process.
Sulfur and sulfur containing ingredients. Sulfur is known for its ability to aid in the cleavage of disulfide bonding, which assists the unraveling of long twisted protein molecules. This reaction with the protein molecules causes increased expansion, smooth product surface and adds stability to the extrusion process. These benefits, however, are not without some undesirable side effects that include off- flavors and aroma.
Sodium metabisulfite, sodium bisulfite, as well as cystine, can be used with effects similar to those from using sulfur.
The normal dosing levels for sulfur or sulfur derivatives - are in the 0.1 to 0.2% range. Cystine is used at approximately 0.5 to 1% level.
Color enhancers. When supplementing light colored meats with meat extenders made from textured vegetable proteins, it is desirable to bleach or lighten the color of the extruded meat extender. Bleaching age nts such as hydrogen peroxide are often used for this purpose. Dosing levels for the hydrogen peroxide range from 0.25 to 0.5%. Pigments such as titanium dioxide are also used at levels between 0.5 and 0.75% to lighten color, but at increased levels will weaken the textural properties of extruded vegetable proteins.
Extrusion Process
The above raw materials or combinations are generally mixed prior to the extrusion cooker, except in the cases where small liquid additions can be added directly to the base raw materials in the extrusion cooker itself.
Definition and functions of extrusion of textured proteins. Extrusion cooking has been defined as "the process by which moistened, expansile, starchy and/or proteinaceous materials are plasticized in a tube by a combination of moisture, pressure, heat and mechanical shear. This results in elevated product temperatures within the tube, gelatinization of starchy components, denaturization of proteins, the stretching or restructuring of tractile components and the exothermic expansion of the extruder" (4). Extrusion is widely used to accomplish this restructuring of protein-based foodstuffs to manufacture a variety of textured convenience foods. When mechanical and thermal energy are applied during the extrusion process, the macromolecules in the proteinaceous ingredients lose their native, organized structure and form a continuous, visco-elastic mass. The extruder barrel, screws and die align the molecules in the direction of flow. This realignment "exposes bonding sites that lead to crosslinking and a reformed, expandable structure' that creates the chewy texture in fabricated foods (5).
In addition to retexturing and restructuring vegetable food proteins, the extrusion cooking system performs several other important functions: It denatures proteins. Proteins are effectively denatured during the moist, thermal process of extrusion. Denaturation of protein "lowers solubility, renders it digestible and destroys the biological activity of enzymes and toxic proteins" (6). It causes deactivation of residual heat- labile growth inhibitors native to many vegetable proteins in a raw or partially processed state. Growth inhibitors exert a deleterious physiological effect on man or animals, as revealed by growth or metabolism studies. It controls raw or bitter flavors commonly associated with many vegetable food protein sources. Many of these undesirable flavors are volatile in nature and are eliminated through the extrusion and decompression of the protein at the extruder die. The use of preconditioning and atmospheric-venting devices in the design of the extrusion system also assists in volatilization and removal of off- flavors. It provides a homogeneous, irreversible, bonded dispersion of all microingredients throughout a protein matrix. This not only insures uniformity of all ingredients such as dyes throughout the product, but provides a means whereby minor ingredients can be intimately associated with potential reaction sites promoting cross-linking or other desirable che mical and physical modifications. And, it controls the shape and size of the final extruded protein in convenient and transportable portions for packaging in the retail or institutional marketplace.
Methodology of extruders relating to texturized proteins.
It is convenient to divide the basic components of an extrusion system barrel configuration, and the die and knife assembly. The design of each of these components is engineered to accomplish a specific function in the process of texturizing vegetable food proteins. Within the design features, the operating conditions are adjusted to vary the texture of the finished product. The effects of each processing step on the product will be addressed specifically.
A typical arrangement of the components of an extrusion system is shown in Figure 1. This arrangement includes a live bin/feeder, preconditioner and extrusion cooker. The live bin/feeder provides a means of uniformly metering the raw materials (granular or floury in nature) into a preconditioner or mixing cylinder and subsequently into the extruder itself. This flow of raw materials must be uninterrupted and rate-controllable. This component controls the product rate or throughput of the entire system. The feeder portion also can be used to initiate the preconditioning of raw materials through the injection of steam to control raw material temperature and moisture. Not all extruded products require a preconditioning step. It is particularly useful where the particle size of the raw vegetable protein is large (a grit or flake). This initial steam preconditioning promotes moisture and heat penetration of the individual particles, resulting in uniform moisture application and elevated raw material temperature. The raw material then passes through the atmospheric preconditioner or mixing cylinder where moisture can be uniformly applied in the form of water and/or steam to achieve a moisture content of 10 to 25%. If steam is applied, it is carefully metered into the raw material to precondition it at modest temperatures of 65 to lOO°C. The mixing cylinder is vented to avoid excess steam and undersirable volatile flavour components found in the raw vegetable protein. Flavours, coloring agents and other additives may also be introduced at this phase of the process to insure thorough and continuous mixing of all foodstuffs entering the extruder barrel. As mentioned earlier, the preconditioning step is also an effective means of initiating control of growth inhibitors found in many raw vegetable proteins.
Fig. 1 Cutaway view of a high capacity extrusion cooker.
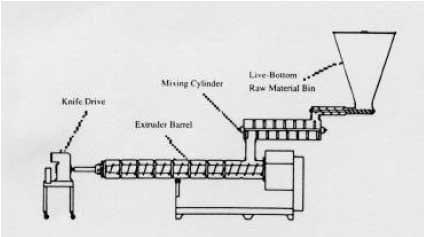
Most preconditioners (Fig. 2) contain one or two mixing! conveying elements which consist of rotating shafts with radially attached pitched paddles. Atmospheric or pressurized chambers may be utilized in this preconditioning step. Pressurized preconditioners can achieve higher discharge temperatures, but have the disadvantages of potential nutrient destruction and higher operating costs. Without the use of preconditioning, it is difficult to make good laminar-structured, textured soy protein. Unpreconditioned vegetable proteins have a strong tendency to expand rather than laminate due to nonuniform moisture penetration which does not allow uniform alignment of protein molecules. Uniform moisture penetration of raw ingredients significantly improves the stability of the extruder and final product quality. This moisture-temperature-time history allows the extrusion of raw materials having larger particle sizes without sacrificing final product quality.
Fig. 2 Typical preconditioners.
The preconditioner discharges directly into the extruder assembly which consists of the barrel and screw configuration. Here, the major transformation of the raw or preconditioned vegetable protein occurs, which ultimately affects the final product texture. Extruders are popularly classified as either being a single or twin screw design. In both designs, the impact on final product texture is affected by screw and barrel profile, screw speed, processing conditions such as temperature, moisture, pressure, raw material characteristics and die selection.
The initial section of the extruder barrel is designed to act as a feeding or metering zone and simply conveys the raw or preconditioned vegetable protein away from the inlet portion of the barrel and into the extruder. The product then enters a processing zone where the amorphous, free-flowing vegetable protein is worked into a colloidal dough. The compression ratio of the screw profile is increased in this stage to assist in blending water or steam with the raw material. The temperature of this moist, proteinaceous dough is rapidly elevated in the last 2-5 seconds of dwell time within the extruder barrel. Most of this heat is from mechanical energy dissipated through the rotating screw and may be assisted from the direct injection of steam or from external thermal energy sources. The screw profile can be altered by the pitch, flight height and angle, and steamlock diameter, which affects the conveying of this plasticized food material down the screw channel. The net flow patterns of the product within the screw are quite complicated and difficult to understand and describe. Retention times of 5-15 seconds, temperatures of 100 to 200°C and moisture levels of 15 to 30% all influence the protein dough quality just behind the die and the final product expansion. The temperature at this point actually melts the protein into a visco-elastic, plasticized mass having very high viscosities. This plasticized material is extruded through the die openings, and expansion occurs as the product is released to ambient pressures. Final product density has been shown to correlate with temperatures and moistures of extrusion.
Extruded meat analogs. It is possible to produce an extruded meat analog which has a remarkable similarity to meat in appearance, texture and mouthfeel (7). Although the process to extrude meat analogs is similar to that used for the production of meat extenders, it often uses two extruders or a long-barreled, single extruder. Through this configuration, the dwell time in the extruder is extended, the moisture content increased to 30% and the pressure maintained below 150 PSIG. The vegetable proteins exposed to this process are stretched, cooled and formed into uniform layers of parallel orientation and cut into bite-sized portions. The lower pressures used inboard of the die reduces product expansion and disruptions to the laminar structure. Due to the dense nature of these analogs, they are able to retain their integrity and meat- like texture even after retorting them for 2-3 hours.
The extruder barrel. The above discussion is valid for processing texturized protein on both single and twin screw extruders, however, there are differences between these two extruder barrel designs. Single screw extruders are the machines that have produced and still produce the greatest tonnage of textured soy protein products in the world. Over the years of extrusion development, the process has become more of a science, which has resulted in the ability to design screws, heads and shearlocks for single screw extruders for specific functions. This variety or selection of parts includes straight and spiral-ribbed jacketed heads which allow the control of friction or product shear, internally, while the flow of cooling water or heating fluids in the external jacket has allowed control of temperatures of the product as it passes through the various extruder sections. Coupling these heads with the wide range of extruder screws that are unrestricted in their number of flights, the flight pitches, cut flights, the screw volumes and the shearlocks between these screws allow for a very versatile machine that has been successful in producing a wide range of products. In the case of texturized proteins, the use of a narrow range of raw materials has been the limiting factor in the use of single screw extruders. Their use requires good uniform raw materials and a maintenance program to keep the extruder barrel parts within tolerances.
The twin screw extruder barrel generally used in the food industry consists of two corotating intermeshing self-wiping sets of screws. Because the screws intermesh they do have restrictions in screw design. They are limited by the flight profile and root diameter. The twin screw extruder has a segmented smooth bore head that is jacketed. The screws are also segmented so that their arrangement can be modified. The twin screw barrel design does have the advantage of a more positive feeding characteristic over the single screw barrel design. The twin screw design, as a result, has been more lenient in raw material specifications.
In either case, single or twin, the combination of preconditioning and the extruder barrel arrangement results in modification to the raw materials and the result is a texturized protein. Hydration and heating cause unravelling of the long twisted protein molecules in vegetable proteins. In the extrusion process, these molecules align themselves along the streamline flows of the screws and dies. The increase in shear temperature and retention time causes cross-linking to occur, yielding a textured product that is layered, and the resulting denaturation or cross-linking can be considered an irreversible endothermic chemical reaction. The extent of cross-linking seems to be a function of the time, temperature and moisture history and can be related to changes in apparent viscosity of the extrudate. The proper exposure to shearing action, as the protein molecules align themselves for crosslinking during the extrusion process, is important.
The rheology of soy proteins or soy protein doughs will not be discussed in this paper. Several papers published in the past describe the effects of shear rate, shear stress, adequate time and temperature history (5,8,9). When a proper screw profile for the extruder is derived, one can meet final product quality requirements with a much broader range of raw materials.
Die. Dies for texturized vegetable proteins should have smooth streamlined flows that do not disrupt or cause shearing effects to the already laminated and cross- linked protein molecules (8). Harper describes in detail the effects of various land lengths and an open area of dies for extruding texturized vegetable protein.
On-Going Research
Various studies have been performed to confirm and demonstrate the use of a broader range of raw materials. These studies include the examination of textural properties, and the work was performed with a Wenger TX-80 twin screw extruder at the Oak B. Smith Extrusion Research Center in Sabetha, Kansas.
Protein dispersibility index range tests. Commercially available ingredients were used in the tests. Soy flour (200 W) from Central Soya with a PDI of 70 and soy grits from A.D.M. (Nutrisoy) with a PDI of 20 and particle size of 20 mesh were mixed together to obtain five mixes with various PDI levels ranging from 20 to 70. These are shown in Table 1.
TABLE 1 Formulas and PDI's for PDI Range Tests
All the formulations were run under approximately the same extruder conditions and with the same final product densities. Samples from the five formulations were tested for water holding capacity (WHC) and textural integrity (TI). Table 2 shows that for the full range of PDI levels, there is no significant difference in water holding capacity or textural integrity, with the exception of Test #2. The increased WHC and textural integrity values in Test #2 can be attributed to the processing conditions of the extruder that yielded a lighter density product.
TABLE 2 Effects of Raw Material PDI On Final Product Quality
During these energy-conscious times we must point out that using raw materials with decreasing PDI levels also requires additional specific mechanical energy input to maintain the same quality of texture in the extrudate (Table 3). This is due to the reduction in available reactive sites for cross-linking in the lower PDI soy protein, thus requiring additional shearing action to expose those reactive sites.
TABLE 3 Required Energy Requirements for Extruding Soy Proteins at Various PDI Levels
Fat level tests. It is an advantage in certain situations to have the ability to texturize vegetable proteins having high levels of residual fat. For example, it may be more economically feasible to use mechanically extracted soy meals which have fat levels between 3 and 6.5% to produce meat extenders and meat analogues. It also has been documented that texturization of whole soybeans via extrusion processing improves the feeding quality of soy protein products (Table 4).
TABLE 4 Effect of Processing on Nutrient Performance
Table 5 shows the materials used, and Table 6 shows the processing conditions and product characteristics of extruded raw materials having various fat levels. In general, increased fat levels in vegetable proteins require increased shear energy input and higher temperatures to maintain a desired product integrity.
It can also be noted that the split and whole beans were texturized and they contained 18 to 20% fat. However, these products did not have desirable characteristics after rehydration. The defatted soy flour with 4.5% fat added into the raw material did produce a good laminar product with good integrity.
TABLE 5 Fat and Fiber Content of Raw Materials
TABLE 6 Effect of Raw Materials and Processing Conditions on Rehydrated Product characteristics
Fiber levels. Table 7 presents the materials used in these tests. As discussed earlier, fiber inhibits the cross- linking of protein molecules necessary for good textural integrity.
This is overcome by imparting more shear as the product passes through the extruder barrel. Results are in Table 8.
TABLE 7 Fiber Content of Commercially Available Soy Proteins
TABLE 8 Effect of Fiber Content on Final Product Quality
Particle size tests. In time past, particle size variations have been important in achieving the proper textural properties in a textured soy product. Table 9 shows particle size and PDI's of three raw ingredients that were extruded yielding the same bulk densities. It appears from Table 10 that the particle size had no significant effect on the product's textural properties. Preconditioning of the raw ingredients may be the highest contributor to the insensitivity to particle size variation for these test runs.
TABLE 9 Ingredients of Various Particle Size
TABLE 10 Effect of Raw Material Particle Size on Final Product Quality
Effects of screw profile and retention time. Table 11 shows the effect of screw profile on final product characteristics. The table has a section on continuous fighting of the extruder screws and a section regarding screw fighting that is interrupted or has breaks in the screw profile.
Three types of products were made of both continuous and interrupted fighting. These are dense chunks, light chunks and blown product (hamburger style TVP).
TABLE 11 Effect of Screw Profile on Final Product Characteristics
The processing conditions, preconditioning temperature, RPM, extrusion temperature, moisture added and energy input were kept as close as possible to the same for each case.
Product density was maintained; however, the water holding capacity went down especially on the light chunk and blown product, as interrupted fighting was used. The test data demonstrated that although adequate shear rates are necessary to enhance the cross-linking effects of protein molecules, over-shearing, once the cross-linking has begun to occur, may disrupt the layered structure of the protein molecules, resulting in decreased water holding capacities.
The effect of moisture on the rheology of soy protein doughs is shown in Table 11. Increased moisture levels increase mobility of the chemically reactive protein molecules, increasing the potential for reactive sights to come in close proximity, which facilitates cross-linking, resulting in increased densities of the extrudate.
Table 12 shows a 36% increase of retention time in the extruder barrel. This allows an approximately equal increase in the maximum capacity of the extruder, while maintaining an equivalent final product quality. At the same time, the required specific mechanical energy input per kilogram of extrudate is reduced.
TABLE 12 Effect of Retention Time on Maximum Capacity of Extruder
Product enhancement concepts. Due to consumer preferences there is a growing demand for marketing gimmicks. Therefore, product shape and design have become an important issue in extrusion processing. Recent developments allow increased flexibility in color development of extruded products.
Dyes or color pigments may be injected with high pressure pumps into the final sections of the extruder barrel to achieve special effects such as marbling, striping and multiple colored products. The conical designed final screw and head section that divides the product flow into separate chambers allows tremendous flexibility of product enhancement, not only with color schemes, but with the addition of heat-sensitive chemicals for textural development or reactions.
Post Extrusion Processes
These are processes that are performed on the extrudate prior to the drying and cooling system. The most predominate process would be particle size reduction. This is normally referred to as "wet milling," as the moisture content is high at this point. There are various devices that can be used for particle size reduction of textured protein and these can include hammermills, rotating cutting heads and shredders. Wet milling tests were performed on light and medium density chunks and the results are shown in Table 13.
Grinding devices used must be based on desired final product sizes and intended uses. Generally speaking the hammermill style device will yield a higher final bulk density as a result of fines generated. The rotating cutting head and shredder designs will generate less fines and maintain the approximate bulk density of the original sample. There are adjustments that can be made in each of these grinding devices to modify the particle size generated.
TABLE 13 Comparison of Wet Milling Devices for Textured Soy Protein
Drying and Cooling
In the extrusion processing of textured proteins the moisture content is elevated, and as a result, the cooked product must have the moisture reduced for safe storage prior to its use. Proper design and size selection of the dryer cooler is important for making good quality products, as well as overall cost of production, because this production step is generally the most costly.
There are many dryer/cooler designs, but for the purpose of discussion, we will review the tray-type dryer/coole r (Fig. 3), because it is the most popular style for textured soy protein production. Tray-type dryer/coolers can be built with various lengths, widths, number of passes, materials, conveyor styles, air flows, sanitary considerations and heating systems.
Fig. 3 Dryer/cooler.
The ability to construct dryer/cooler with various lengths, widths and number of passes allows proper sizing for the extruder output, as well as offering flexibility in equipment placement in existing or new facilities. The two pass design not only saves floor space, but allows the product to be turned over in the dryer, improving evenness of drying. Using varispeed motors on the dryer passes allows for changes in the product bed depth, and thus retention time of the product in the dryer.
Dryer/cooler cons truction materials include mild steel, stainless steel and combination of both. In human food production the dryer/coolers are normally equipped with stainless steel, at least where the product comes in contact with the dryer, or are all of stainless steel construction. Product-carrying conveyors are perforated mild or stainless steel trays that can be coated with teflon or silverstone. Also available are wire mesh screens that are carried on special supports. The wire mesh design has greater open area for even air flow and allows for smaller particles to be dried and retained on the conveyor.
Air flow is generally up through the product with the heating elements mounted in a single side plenum chamber above air recirculation fans. These recirculation fans create the static pressure below the dryer trays resulting in evenness of drying across the product bed. A percentage of air is exhausted to control the humidity in the dryer, while the balance is recirculated, which permits maximum efficiency in energy consumption. The heating elements mounted in the side plenum are generally steam coils or gas burners. This is usually based on the location of the plant and the cost of the fuel to be used. Steam coils are generally the most popular. Full insulation on all sides of the dryer coupled with the heating systems allow for safe operating temperatures up to 350° (175°).
Sanitary considerations include doors wherever possible for cleaning access and maintenance. Fines recovery systems remove fines from the bottom of the dryer/cooler and deposit them in a cross auger that removes them to the side of the dryer/cooler. Dryers are commonly equipped with integral coolers or extensions of the bottom dryer pass. Internal airlocks are used to reduce the amount of air leakage between these two zones. Air flow is generally down through the product and a percentage of this exhausted air is used as makeup air for the dryer, yielding a more efficient dryer, reducing energy consumption.
Final Products
The products discussed above include texturized proteins in the meat extender form, as well as chunk style products. The meat extenders, when ground to a particle size simulating hamburger, are used at a 25 to 30% level in dishes such as pizza, hamburgers, meatloaf, chili, tacos, enchiladas, sloppy joes and meat sauces. Chunk style products are effectively used in soups, stews, meat pies, dry soup mixes and oriental dishes.
Recently, a new line of natural flavors has come to our attention, and flavor response in meat extender products is remarkable. We have tested beef, ham, chicken, lobster, clam and shrimp flavors and have been quite impressed with their utility, flavor survival through the extrusion cooking process and lack of residual soya flavor in the end product. When blended with a system for producing soups, chowders or gravies, for example, their similarity to meat has proven to be excellent.
Particularly interesting is the idea of using natural flavors as opposed to synthetic flavors. Because the alcohol, fat or water bases of most synthetic flavors are quite volatile, most of the synthetic flavor's effectiveness is lost at the high temperatures necessary for structuring and moisture removal. Additionally, synthetic flavors are often leached out of the product and are often dispersed throughout the liquid phase during the rehydration of textured soy protein in the canning operation or in the making of soups or stews. Conversely, the natural flavor agents we have utilized seem to be enhanced by cooking, drying and rehydration steps and react in much the same manner as natural meats, in that thermal processing is required to bring out their flavor. When included in a flavor system such as soup stock or meat gravy, the product may be frozen or canned to provide stability. Additionally, systems may be designed for convenience type products which could include the textured vegetable protein extender in a gravy or soup stock to be finished by the end user with fresh vegetables, noodles or other constituents to develop nutritious, inexpensive and convenient complete meals, entrees or soups.
Discussions above on textural integrity and water-holding capacity help food scientists determine how texturized proteins will function in the food systems utilizing these products. The following test procedures are the ones used in the studies.
Procedure for determining water holding capacity.
(Equipment Needed: Beaker, sieve screen and scales)
Weigh out small amount of sample and record weight. Soak in room temperature water for 20 minutes. Let drain on screen for 5 minutes. Reweigh sample. (Rehydrated wt. - Original wt.)/Original wt. = Water absorption.
Test for measuring integrity of textured vegetable proteins.
(Equipment needed U.S. 20 mesh screen; gram scale; 600 ml. beaker; aluminium foil; autoclave, capable of holding 15 psi; food grinder with 1.8 in. holes in plate; and water bath. Ingredients needed: At least 50 g of sample and tap water.)
Begin test by soaking at least 50 g of sample in a 600 ml beaker with sufficient water to fully cover all of sample and allow for absorption. Let hydrate one hour, stirring occasionally. After hydration, cover beaker with aluminium foil and place in a preheated retort. Pressure cook for 30 mm under pressure at 15 psi (250°). Cool sample to 70-80° by putting beaker in running tap-water bath. Do not pour off excess water. Grind hydrated sample in food grinder fitted with plate with 1/8 inch diam. holes. If water comes out of grinder, keep with sample. Weigh out 50 g of the hydrated, cooked and ground sample and spread evenly on a U.S 20 mesh screen. Spray rinse the sample on the screen for one minute with tap water at ambient temperature under 12 psi. Spray nozzle should be approximately 9 in. from the screen and slowly moved back and forth and rotated so that the spray water contacts the entire screen surface during the rinsing operation. Flow of water should be about 3 gal in 1 mm. Shake off excess water. Drain sample on the screen for 4 mm. During the draining period, blot the bottom of the screen with paper towels. Weigh the residue. (This is most easily done by having the screen tared out on the scales before the test begins. Then you can weigh out the residue with the tared screen). To calculate integrity index, divide the weight of residue by the weight of hydrated sample.
Example:
If 50 g of sample were spray rinsed, and 55 g of sample was left on the screen after rinsing, 55/50 - 1. 1 integrity index.
Discussion
Many tests have been performed with a wide variety of raw materials. Soybean-based raw materials such as defatted soy flour, soy concentrates, soy isolates and soy grits with various particle sizes, fat levels and fiber levels can all be used to produce texturized proteins. The new developments in processing techniques will allow work to continue with raw materials such as glandless cottonseed flour, rapeseed proteins or canola concentrates, defatted peanut flour and defatted sesame flour in order to allow a higher utilization of these protein sources. Initial work with these raw materials on single screw extruders resulted in texturized products, however, the marketability of these products are questionable. The use of the twin screw extrusion cookers should revitalize the interest in these raw materials.
Texturized proteins have been successfully marketed and produced throughout the world as a result of food scientists' ability to apply the extrusion process to produce products that fit into the individual society's normal eating habits. Interactions of equipment manufacturers, end users and food scientists will result in further improvements and new developments in the art of producing and utilizing texturized proteins.
Acknowledgement
J. McNab of the Agricultural Research Council, Roslin, Scotland, performed metabolizable energy and fat digestion studies used to prepare Table 4.
References
1. Simonsky, R.W., and D.W. Stanley, Can. Inst. Food Sci. Technol. J. 15:294 (1982).
2. DeGroot, A.P., P. Slump, V.J. Feron and L.Van Beek, J. Nutr. 106:1527 (1976).
3. Boison, G., M.V. Taranto and M. Cheryan, J. Food Technol. 18:719 (1983).
4. Smith, O.B., "Textured Vegetable Proteins" presented at the World Soybean Research Conference, University of Illinois, Aug. 3-8, 1975.
5. Harper, Judson, Food Technol. 40:70 (1986).
6. Altschul, A.M., Basic Books, New York (1965).
7. Smith, O.B., "Products of Textured Soy Proteins," 1st Latin America Soy Protein Conference, Mexico City (Nov. 1975).
8. Holay, S.H., and J.M. Harper, J. Food Sci. 47:1869 (1982).
9. Hagaiv, R.C., S.R. Dahl and R. Villota, J. Food Sci. 51:367 (1986).