Introduction
Sustainability of dairy farm production systems can be measured by the intersection between profitability and environmental stewardship. It is clear that unprofitable farms are destined to go out of business. Similarly, although not as evident, farms that are not efficient in the use of input resources, having higher wastages, and consequently producing more emissions, are likely to be un-sustained in the long run. Dairy farm production systems need to remain profitable and at the same time environmental compliant to be sustainable. In simple terms, environmental efficiency or environmental compliance refers to the balance of nutrients used for production purposes. Using more of the input resources in effective production, and consequently having fewer emissions, distinguishes the most efficient systems. Several nutrients or compounds are of environmental interest: for example greenhouse gasses (GHG) because of global warming potential, P because of surface water eutrophication issues, or N because of groundwater contamination problems. This study deals specifically on the joint between greenhouse gasses emissions and profitability for 3 distinctive dairy farm production systems in Wisconsin – conventional, grazing, and organic; and it is an adaptation of Dutreuil et al. (2014) study.
Greenhouse gas emissions need to be reduced in order to limit undesirable outcomes of climate change (IPCC, 1994). Livestock operations are one of the largest sources of agricultural GHG emissions (EPA, 2009), and milk production is considered to be responsible for 4% of global anthropogenic emissions of GHG (FAO, 2010). An important challenge for the dairy industry is to reduce emissions of GHG while remaining economically competitive. The 3 main GHG are carbon dioxide (CO2), methane (CH4), and nitrous oxide (N2O) and their emissions are usually expressed on a CO2 equivalent basis (CO2 eq) to represent their global warming potential in the atmosphere. Methane and N2O have global warming potentials 25 and 298 times of that of CO2, respectively (IPCC, 2007). Sources of CO2 on the dairy farm include plant respiration, animal respiration, and microbial respiration in the soil and manure. Carbon dioxide can also be assimilated on the farm via carbon fixation (Rotz et al., 2011). Methane sources include enteric fermentation, manure storage, field application of manure and feces deposited on pasture or on the barn floor (Rotz et al., 2011). Sources of N2O on the farm include soil and manure through the processes of nitrification and denitrification (Rotz et al., 2011).
Simulation is a powerful tool to integrate the impact of management practices on both GHG emissions and economic outcomes within a whole farm system framework. In this study, two areas of management were targeted for mitigation strategies: feeding management because of its impact on enteric CH4 emission (Aguerre et al., 2011) and manure management because it is a major source of GHG emissions on dairy farms (Sommer et al., 2000, Chadwick et al., 2011, Thoma et al., 2013). The Integrated Farm System Model (IFSM) was used to define and study management strategies in different farm systems (Stackhouse-Lawson et al., 2012; Belflower et al., 2012; Rotz et al., 2007) and to assess simultaneously the combined effect of feeding and manure management strategies on GHG emissions and profitability.
Materials and Methods
Integrated Farm System Model
The IFSM was used to quantify economic and environmental impacts of different management strategies in the 3 defined dairy production systems. The IFSM is a simulation model that integrates the major biological and physical processes of a dairy farm and assesses economic and environmental performances given a set of management practices (Rotz et al., 2011).
Simulated farms and management scenarios
Data collected from a survey on 27 conventional farms, 30 grazing farms and 69 organic farms (Hardie et al., 2014) were used to characterize the 3 farm systems. In order to make a fair comparison and eliminate variation in simulation results due to difference in farm size, data from the surveys were scaled to the average Wisconsin surveyed farm in terms of land area (127 ha out of which 79 ha were owned and 48 ha were rented) and number of adult cows (85). Data presented in Table 1 used in the baseline simulations came directly from surveys. Same soil type (medium clay loam) and daily weather including minimum and maximum temperature, precipitation, and solar radiation for 25 years (1986-2010; Madison, WI) –available from official government sources– were used for the 3 simulated production systems. The 3 systems also used the same cost of diesel fuel ($0.68/L), electricity ($0.11/kWh), labor wage ($11.24/h), land rental ($215/ha), and fertilizers: nitrogen ($1.1/kg), phosphate ($1.199/kg), and potash ($1.001/kg), based on local market reports. Following knowledge gained during the survey, all crop operations but grain harvests were completed by on-farm labor and alfalfa was established for 3 years with oats as a cover crop, which was harvested using a 3-cut strategy, in which first and third cuts were harvested as silage and the second as hay. Also in the 3 farm systems, young stock animals were housed in a bedded pack barn. Estimates from previous studies were used for some data not available from surveys such as some economic parameters for feed prices (Rotz et al., 2007, Rotz et al. 2008) and veterinary and breeding costs (Kriegl, 2007).
Conventional farm.
Grass was established for 10 years with 10% White Clover and 90% Orchardgrass. Only one cut of hay was harvested from the 22.4 ha at the beginning of the grazing season. Older heifers and dry cows were grazed in this field the rest of the time. Cows were milked twice daily in a double 8 parlor. The number of heifers was 35 for the young ones (< 1 year old) and 40 for the old ones (> 1 year old). Manure was collected using a scraper with a slurry pump and stored in a 6-month storage pit. Eighty percent of the manure collected was applied to the cornfield. The remaining 20% was applied to the alfalfa field. Three different scenarios were simulated for the conventional farm. In the first scenario, lactating cows were grazed during the grazing season. As a result, the time of labor needed for the animal grazing was increased from 2 to 6 h/week. The impact of grazing on GHG emission was assessed using the same milk production (scenario 1, Table 2) and a 5% decrease in milk production (Vibart et al, 2008) (scenario 2, Table 2). Other scenario (scenario 3, Table 2) looked at the effect of manure management. In this scenario, the manure was incorporated in the soil the same day it was spread. At that same time the 6-month storage pit was replaced by a 12-month covered tank storage to limit emissions from the manure storage. Other scenarios looked at the combination of previous scenarios (scenarios 1 and 3, and 2 and 3, Table 2).
Table 1. Mean (standard deviation) of main characteristics of simulated dairy production systems
Grazing farm.
Grass was established for 5 years with 45% White Clover and 55% Orchardgrass. Two cuts of hay were harvested from the 61.9 ha and all the animals were grazed. Cows were milked twice daily using a pipeline system. The number of heifers was 34 for the young ones (< 1 year old) and 36 for the old ones (> 1 year old). Manure was collected using gutter cleaners and hauled daily (i.e., no storage manure on the farm). Fifty percent of the manure collected was applied to the cornfield, 20% to grassland, 20% to alfalfa field, and 10% to the oat field. Three different scenarios were simulated on the grazing system. In the fist scenario, the forage to grain ratio was set from high to low. A high forage to grain ratio consists of 83, 90 and 93 % of forage in the diet for the early, mid and late lactation cow, respectively. A low forage to grain ratio consists of 57, 68 and 80 % of forage in the diet for the early, mid and late lactation cow, respectively (Rotz et al, 2011). As a result, milk production was increased by 5% (Aguerre et al, 2011) (scenario 6, Table 2). A 10% increase was also simulated (Sterk et al, 2011) (scenario 7, Table 2). Other scenario looked at the effect of manure management (scenario 3, Table 2). The manure was now incorporated into the soil the same day it was spread at the same time that a 12- month covered tank was added to the farm. Other scenarios looked at the combination of previous scenarios.
Organic farm.
Two hectares on the organic farm were not available for crop production due to the organic regulation for buffer zones between organic and conventional land (USDA Agricultural Marketing Service. 2013; Rotz et al., 2007). Grass was established for 5 years with 35% White Clover and 65% Orchardgrass. Two cuts of hay were harvested from the 43 ha and all of the animals were grazed. Cows were milked twice daily using a pipeline system and housed in a tie stall barn. The number of heifers was 33 for the young ones (< 1 year old) and 40 for the old ones (> 1 year old). Manure was collected using gutter cleaners and hauled daily (i.e., no storage manure on the farm). Fifty percent of the manure collected was applied on cornfield, 20% on grassland, 20% on oats, and 10% on alfalfa. The same scenarios used on the grazing system were simulated on the organic farm (Table 2).
Results
Comparison of production systems
The 3 systems produced enough forages to feed 85 cows and their replacements. Due to higher yields, the conventional system was able to sell more forages than the grazing or the organic systems (236 vs. 192 and 94 tonne/year, respectively). However, in order to maintain a higher milk production than the 2 other systems, the conventional system needed to buy more grain (187 vs. 104 and 107 tonne/year, respectively) and more soybeans (19 tonne/year vs. grazing and organic systems selling it in quantities of 12 and 4 tonne/year, respectively).
The organic farm had a high income from milk sales (Figure 1) due to a higher milk price, and an intermediate feed cost ($149,744/year) leading to the highest net return to management ($59,120/year, Table 2). Moreover, the variation in net return across years was the smallest for the organic system. The conventional system had an intermediate net return to management of $23,895/year. The high milk production compared to the grazing system (9,735 vs. 7,256 kg/year) compensated for the high feed cost ($182,124/year) in the conventional system. Although the grazing system had the lowest feed cost ($134,133/year), it had low milk production that led to the lowest net return to management ($14,439/year; Table 2).
Figure 1. Feed costs and income on the simulated dairy farm systems ($/year)
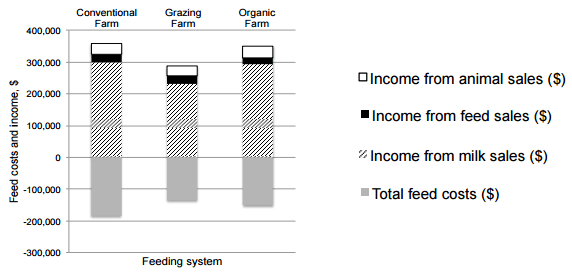
On a yearly basis, the conventional farm emitted the greatest amount of kg CO2eq/year GHG (476,623), the grazing system emitted the lowest amount (405,565), and the organic system had an intermediate level (454,780) (Table 2). However, when GHG emission was expressed in function of milk produced (CO2eq/kg milk) the emission from the conventional system was the lowest (0.58) followed by the grazing system (0.66) and the organic system (0.74). For the 3 systems, the major sources of GHG emissions were the GHG emitted from the barn floor, the animal respiration and the enteric fermentation when animals are housed inside the barn (housing facilities). Other important sources of GHG emissions on the conventional farm included secondary sources (4.60 kg CO2eq/cow per d), manure storage (3.93 kg CO2eq/cow per d) and feed production (3.47 kg CO2eq/cow per d). For the grazing and the organic farms, other important sources were feed production (4.11 kg CO2eq/cow per d and 4.60 kg CO2eq/cow per d, respectively) and grazing (6.75 kg CO2eq/cow per d and 6.44 kg CO2eq/cow per d, respectively) (Figure 2). These results are consistent with previous studies with respect to the reported GHG amounts and ranges and in the fact that the major factor affecting the amount of GHG emission per kg of milk is milk production (Gerber et al., 2011; Kristensen et al., 2011).
Table 2. Economic effects and greenhouse gas emissions for various dairy farm management changes. Bolded numbers indicate best results by row
Figure 2. Sources of GHG emissions on the studied dairy farm systems
Strategies to reduce GHG emissions
Conventional farm.
Scenario 1 simulated on the conventional system looked at the impact of grazing lactating cows. If the milk production was maintained at the same level, grazing lactating cows increased net return to management (+$7,005/year) and decreased GHG emission by 27.6% (-0.16kg CO2eq /kg of milk) (Table 2). Because lactating cows were grazing, less preserved forage was needed during the grazing season and the farmer was able to sell 33 tonne/year more forage than in the baseline scenario. This amount of forage sold contributed to an increase of $3,684/year from feed sales. Net return to management increased also because of a decrease of $3,856/year of total manure management cost. The time spent by the cows grazing decreased the amount of manure which needed to be stored and handled, reducing the cost of manure management. The decrease in GHG emission was possible for the same reasons. Because cows were spending time outside, GHG emission from housing facilities and manure storage decreased (-5.25 kg CO2eq/cow per year and -2.63 kg CO2eq/cow per year, respectively) and emission from grazing increased (+3.55 kg CO2eq/cow per year), resulting in a net reduction in GHG emission when grazing substitute for confinement feeding during part of the year. When milk production was decreased by 5% (scenario 2), GHG emissions decreased by 26% (-0.15kg CO2eq /kg of milk). However, the increase in income from forage sales was compensated by the decrease in milk production and the net return to management stayed almost the same compared to the baseline scenario. Consequently, the net return to management per kg of milk was slightly better for scenario 2 compared to the baseline scenario ($29.1 vs. $28.9/tonne of milk, respectively). The 12-month covered tank and the incorporation of manure the same day of application (scenario 3) led to an increase of total manure cost of $3,398/year. For this reason, the net return to management decreased to $20,359/year. The change in manure management was beneficial for GHG emissions, which decreased by 16% (-0.08kg CO2eq/kg of milk, Table 2). The decrease in emissions from the manure storage and from the field during feed production contributed to this improvement.
Scenario 4 and 5 looked at the interaction of previous scenarios simulated on the conventional system. If milk production was maintained at the same level (scenario 4), the adoption of grazing for the lactating cows and the changes in manure management led to an increase in net return to management (+$3,180/year) and a decrease in GHG emission of 31% (-0.18 kg CO2eq/kg milk). The change in net return was the result of the increase in income from feed sales, the decrease in total manure cost observed in scenario 1, and the increase in manure costs observed in scenario 2. The change in GHG emission was the result of a decrease in emission from the housing facilities and the manure storage. However, the overall decrease in GHG emission for scenario 4 compared to the baseline scenario did not add up to the decrease observed in scenarios 1 and 3. If milk production was reduced by 5% (9,329kg/cow per year, scenario 5), GHG emission decreased by 0.18 kg CO2eq/kg of milk, but net return to management was also reduced by $4,641/year compared to the baseline scenario.
Grazing farm.
Scenario 6 simulated on the grazing farm looked at the impact of changing the forage to grain ratio from high to low. This strategy did reduce GHG emission by 25.8% compared to the baseline scenario (-0.17 kg CO2eq/kg of milk), but also reduced substantially the net return to management (-$12,846/year or -$151/cow per year) when milk production was increased by only 5% (Table 2). In such case, the income from the increase in milk production and the additional forage sold (92 tonne/year) did not cover the expenses needed to buy the additional grain. Feed costs increased by $34,797/year compared to the baseline scenario. In regard to GHG emission, the increase of 2.39 kg CO2eq/ cow per year from secondary sources due to the grain purchased was offset by the reduction in emission from housing facilities (-2.28 kg CO2eq/cow per year), grazing (-2.16 kg CO2eq/cow per year) and feed production (-1.13 kg CO2eq/cow per year). When milk production was increased by 10% (scenario 7), the impact of changing the forage to grain ratio was essentially the same on GHG emission and the net return was less reduced (-$4,683/year) compared to the baseline scenario.
Adding a 12-month covered tank and incorporating the manure the same day of application (scenario 3) increased GHG emission (0.04 kg CO2eq/kg of milk) and decreased net return to management (-$3,565/yr) (Table 2). The reduction in net return was due to an increase of total manure cost of $3,521/year compared to the baseline scenario. The reduction of GHG emission from feed production (-0.27 kg CO2eq/cow per year) possible with the incorporation of manure the same day of application did not compensate for the increase in GHG emission from the manure storage (1.25 kg CO2eq/cow per year), leading to an overall increase of 0.79 kg CO2eq/cow per year.
When forage to grain ratio was set to low, milk production increased by 5% and a manure management change was imposed (scenario 9), net return was decreased by $16,407/year compared to the baseline scenario due to an increase in feed cost from the grain purchased and an increase in total manure costs. When milk production increased by 10% together with a change in manure management (scenario 10), net return was decreased by $8,247/year for the same reasons. The overall GHG emission was reduced by 0.13 and 0.15 kg CO2eq/kg of milk for a 5 and 10% increase in milk production, respectively (Table 2).
Organic farm.
Results in the organic system followed the same trend as for the grazing system. A 5% increase in milk production and having forage to grain ratio from high to low (scenario 6) decreased the net return to management by $9,766/year and decreased the GHG emission by 0.23 kg CO2eq/kg of milk (Table 2). With a 10% increase in milk production and forage to grain ratio low (scenario 7), GHG emissions were reduced by 0.25kg CO2eq/kg of milk while net return to management was still slightly increased. A 10% increase in milk production was sufficient to maintain net return compared to baseline scenario in spite of an increase in feed costs (Table 2). In scenario 3, net return to management decreased by $4,855/yr because of an increase of total manure cost and GHG emission increased by 0.06 kg CO2eq/kg of milk. With a 5% increase in milk production and a change in manure management (scenario 9), net return to management decreased by $14,793/yr and GHG emissions decreased by 0.18 kg CO2eq/kg of milk compared to the baseline scenario. With a 10% increase in milk production and a change in manure management (scenario 10), net return to management decreased by $4,403/year and GHG emission decreased by 0.20 kg CO2eq/kg of milk (Table 2).
Conclusions
Opportunities exist to reduce GHG emissions and maintain or even increase profitability by feeding management strategies, regardless of the type of dairy production system: conventional, grazing, or organic. In contrast, under the simulation conditions of this study, manure management changes to reduce GHG emission had a negative impact on profitability. However, the evaluation and the implementation of mitigations strategies should be based on farm system specific characteristics. Despite great strengths in modules such as crops, machinery, feed storage, or grazing, the IFSM has limitations in dairy herd management options. For instance, it assumes a fixed number of herd groups and ignores herd dynamics related to reproduction or replacement, which have important economic and environmental impacts. A sensitivity analysis including more herd-related management strategies together with uncertain factors are recommended for further study.
Acknowledgements
This research was supported by Agriculture and Food Research Initiative Competitive Grant no. 2010-51300-20534 from the USDA National Institute of Food and Agriculture.
Originally published in Proc. VI Brazilian Symposium on Sustainable Agriculture and III International Symposium on Sustainable Agriculture, Federal University of Viçosa, Brazil. 26-27 September 2014.
References
Aguerre, M. J., M. A. Wattiaux, J. M. Powell, G. A. Broderick and C. Arndt, 2011. Effect of forage-to-concentrate ratio in dairy cow diets on emission of methane, carbon dioxide, and ammonia, lactation performance, and manure excretion. J. Dairy Sci. 94:3081-3093.
Belflower, J. B., J. K. Bernard, D. K. Gattie, D. W. Hancock, L. M. Risse, and C. A. Rotz, 2012. A case study of the potential environmental impacts of different dairy production systems in Georgia. Agricultural Systems. 108:84-93.
Chadwick, D., S.G. Sommer, R. Thorman, D. Fangueiro, L. Cardenas, B. Amon, and T. Misselbrook, 2011. Manure management: Implications for greenhouse gas emissions. Animal Feed Science and Technology. 166-67:514-531.
EPA (Environmental Protection Agency). 2009. Inventory of U.S. Greenhouse Gas Emissions and Sinks: 1990-2007. EPA, Washington, DC.
FAO, 2010. Greenhouse Gas Emissions from the Dairy Sector. A Life Cycle Assessment. Food and Agriculture Organisation of the United Nations, Animal Production and Health Division.
Gerber, P., T. Vellinga, C. Opio, H. Steinfeld, 2011. Productivity gains and greenhouse gas emissions intensity in dairy systems. Livestock Science. 139:100-108.
Hardie, C., M.A. Wattiaux, M. Dutreuil, R. Gildersleeve, N. Keuler and V. Cabrera, 2014. Feeding Strategies on certified organic dairy farms in Wisconsin and their impact on milk production and income over feed costs. J. Dairy Sci. (accepted, 24 March 2014).
IPCC (Intergovernmental Panel on Climate Change). 2007. Climate change 2007: The physical science basis. Contribution of working group I to the Fourth Assessment Report of the Intergovernmental Panel on Climate Change. Chapter 2, Changes in atmospheric constituents and in radiative forcing. Available at: http://www.ipcc.ch/pdf/assessment-report/ar4/wg1/ar4- wgl-chapter2.pdf. Accessed 14 April 2014.
IPCC (Intergovernmental Panel on Climate Change). 1994. Radiative Forcing of Climate Change. The 1994 Report of Scientific Assessment. Working Group of IPCC WMO. UNEP. pp 1-28.
Kriegl, T. 2007. Major Cost Items on Wisconsin Grazing and Confinement Dairy Farms. Center for Dairy Profitability, University of Wisconsin-Madison/Extension. http://cdp.wisc.edu/pdf/glgnfacsheet7_yr6.pdf. Accessed April 15, 2013.
Kristensen T., L. Mogensen, M. Trydeman Knudsen, J. E. Hermansen, 2011. Effect of production system and farming strategy on greenhouse gas emissions from commercial dairy farms in a life cycle approach. Livestock Science. 140:136-148.
Rotz, C.A., G.H. Kamphuis, H.D. Karsten, and R.D. Weaver. 2007. Organic Dairy Production Systems in Pennsylvania: A Case Study Evaluation. J. Dairy Sci. 90:3961-3979.
Rotz, C. A., H. D. Karsten, and R. D. Weaver, 2008. Grass-based dairy production provides a viable option for producing organic milk in Pennsylvania. Forage and Grazinglands http://www.plantmanagementnetwork.org/pub/fg/research/2008/organic/. Accessed April 23, 2013.
Rotz, C.A., M.S. Corson, D.S. Chianese, F. Montes, S.D. Hafner, and C.U. Coiner. 2011. Integrated Farm System Model, Reference Manual Version 3.4. Pasture System and Watershed Management Research Unit, USDA Agricultural Research Service. www.ars.usda.gov/SP2UserFiles/Place/19020000/ifsmreference.pdf Accessed October 26, 2012.
Sommer, S. G., S. O. Petersen, and H.T., Sogaard. 2000. Greenhouse gas emission from stored livestock slurry. J. Environ. Qual. American Society of Agronomy, Madison. 29(3):744-751.
Stackhouse-Lawson, K. R., C. A. Rotz, J. W. Oltjen, and F. M. Mitloehner, 2012. Carbon footprint and ammonia emissions of California beef production systems. Journal of Animal Science. 90:4641-4655.
Sterk, A., B. E. O. Johansson, H. Z. H. Taweel, M. Murphy, A. M. van Vuuren, W. H. Hendriks, J. Dijkstra, 2011. Effects of forage type, forage to concentrate ratio, and crushed linseed supplementation on milk fatty acid profile in lactating dairy cows. J. Dairy Sci. 94:6078-6091.
Thoma, G., J. Popp, D. Nutter, D. Shonnard, R. Ulrich, M. Matlock, D. S. Kim, Z. Neiderman, N. Kemper, C. East and F. Adom, 2013. Greenhouse gas emissions from mil production and consumption in the United States: A cradle-to-grave life cycle assessment circa 2008. International Dairy Journal. 31:S3-S14.
USDA Agricultural Marketing Service. 2013. National Organic Program. http://www.ams.usda.gov/AMSv1.0/nop US Department of Agriculture, Agricultural Marketing Service. Accessed April 19, 2013.
Vibart, R. E., V. Fellner, J. C. Burns, G. B. Huntington, and J. T. Green Jr, 2008. Performance of lactating dairy cows fed varying levels of total mixed ration and pasture. Journal of Dairy Research. 75:471-480.