This study aimed to investigate the prevalence, antibiogram of Pseudomonas aeruginosa (P. aeruginosa), and the distribution of virulence genes (oprL, exoS, phzM, and toxA) and the antibiotic-resistance genes (blaTEM, tetA, and blaCTX-M). A total of 285 fish (165 Oreochromis niloticus and 120 Clarias gariepinus) were collected randomly from private fish farms in Ismailia Governorate, Egypt. The collected specimens were examined bacteriologically. P. aeruginosa was isolated from 90 examined fish (31.57%), and the liver was the most prominent infected organ. The antibiogram of the isolated strains was determined using a disc difusion method, where the tested strains exhibited multi-drug resistance (MDR) to amoxicillin, cefotaxime, tetracycline, and gentamicin. The PCR results revealed that all the examined strains harbored (oprL and toxA) virulence genes, while only 22.2% were positive for the phzM gene. On the contrary, none of the tested strains were positive for the exoS gene. Concerning the distribution of the antibiotic resistance genes, the examined strains harbored blaTEM, blaCTX-M, and tetA genes with a total prevalence of 83.3%, 77.7%, and 75.6%, respectively. Experimentally infected fish with P. aeruginosa displayed high mortalities in direct proportion to the encoded virulence genes and showed similar signs of septicemia found in the naturally infected one. In conclusion, P. aeruginosa is a major pathogen of O. niloticus and C. gariepinus. oprL and toxA genes are the most predominant virulence genes associated with P. aeruginosa infection. The blaCTX-M, blaTEM, and tetA genes are the main antibiotic-resistance genes that induce resistance patterns to cefotaxime, amoxicillin, and tetracycline, highlighting MDR P. aeruginosa strains of potential public health concern.
Pseudomonads are one of the most threatening fish pathogens that induce ulcerative syndrome and hemorrhagic septicemia1. Various bacterial pathogens affect a wide range of aquatic species and are responsible for considerable economic losses, worldwide. It was estimated that up to 50% of farms fish are lost due to bacterial infection before their marketing. The economic losses are mainly attributed to poor growth, high mortalities, and improper flesh quality2–5. Pseudomonas aeruginosa is a part of normal fish microbiota, however under stressful conditions such as malnutrition and overcrowding the bacteria have become highly opportunistic and pathogenic, causing serious illness including, hemorrhagic septicemia, gill necrosis, abdominal distension, splenomegaly, friable liver, and congested kidney6.
P. aeruginosa is an aerobic Gram-negative motile, non-spore-forming rods, catalase, and oxidase-positive7. Besides, this bacterium possesses many virulence-related determinants including cell-mediated and secreted virulence types. The cell-mediated types include; pilli, fagella, and lipopolysaccharide (LPS) which are commonly involved in bacterial colonization and motility, delivery of active proteins into the host cells, and establishment of persistent infections8. Likewise, the secreted virulence factors fortify the inflammatory processes, induce severe tissue damages, facilitate bacterial invasion and dissemination, and accelerate the progression of diseases9.
Indeed, the identification of Pseudomonas spp. is essential for accurate diagnosis, outbreaks prediction, and preventive and/or prophylactic measures implementation in aquaculture10. Like most of invading pathogens, phenotypic characterization of P. aeruginosa required protracted and complicated steps of morphological and biochemical identifications, which were intractable, time-wasting, and occasionally unreliable11. Therefore, the molecular-based detection of this pathogen could help to obtain a full idea about the ecological importance of such pathogens and rescind the shortcomings of conventional methods12. Most of the Pseudomonas species showed different resistance patterns against various antimicrobial agents13. specifically, P. aeruginosa could develop innate and/or acquired resistance to several antimicrobial agents due to the active efflux of antibiotics and the permeability of its outer membrane9. Thus, the molecular typing of most inherited antibiotic-resistance genes should be performed to avoid the emergence of antibiotic-resistant strains that have a global health concern14.
Nowadays, serious attempts for further detection of P. aeruginosa were considered not only for its economic impact but also for its public health importance. Pseudomonas infection, generally induced by P. aeruginosa has public health concern and causes healthcare-associated illnesses for many consumers15. Pseudomonas species are presently cataloged as a food-borne illness that affects humans by consuming spoiled foods and ready-to-eat products, as well as manipulating the contaminated seafood16,17. Although few studies have claimed possible routes of disease transmission, people with weakened immune systems or those works with infected fish are usually at risk6. P. aeruginosa infection could occur in humans via the consumption of raw fish and its byproducts18. It is reported that the typical colonies of P. aeruginosa isolated from fish are closely related to those causing hospital-acquired pneumonia in humans17.
The application of the antimicrobial susceptibility testing is ultimately required for disease control. Globally, the extensive use of antimicrobial agents in the aquaculture sector, as well as transmission of multidrug-resistant bacterial pathogens between terrestrial and aquatic ecosystems have been reported19. The antibiotic resistance mechanism mostly occurs due to the transfer of R-Plasmid20. Various reports investigated the occurrence and transmission of antibiotic resistance between human and animal food21, with less attention to fish, so that fewer data are available about the antimicrobial resistance in fish22.
Several virulence factors are commonly associated with P. aeruginosa especially exotoxin A and exotoxin S that are controlled by a cell to cell signaling patterns, in addition, exotoxin A is responsible for the inhibition of protein-biosynthesis, while Exotoxin S is a bi-functional protein that enhances the ribosyltransferase and GTPase activity resulting in cell-apoptosis23. Furthermore, P. aeruginosa produces phenazine compounds, which are biologically active substances involved in bacterial competitiveness and virulence in both human and animal hosts24. The outer membrane proteins (L-lipoproteins) of P. aeruginosa are incriminated in the bacterial resistance to antiseptics and antibiotics25. The resistance of P. aeruginosa to the β-lactam antibiotics including penicillin (1st, 2nd, and 3rd generations) and cephalosporin (such as cefotaxime) is mainly attributed to the Extended Spectrum Beta-lactamases (ESBLs). blaCTX-M and blaTEM are the main Extended Spectrum β-lactamases genes that induce such type of resistance26.
In this context, the present study aimed to identify the phenotypic characteristics of P. aeruginosa strains, retrieved from two freshwater cultured fish species (Oreochromis niloticus and Clarias gariepinus) and their antibiogram. Furthermore, the genotypic characterization of the virulence genes: oprL; outer membrane lipoprotein-L, exoS; exotoxin S gene, phzM; phenazine-modifying gene, and toxA; exotoxin A gene as well as the antibiotic-resistance genes: blaTEM and blaCTX-M; ESBLs genes, and tetA; tetracycline resistance gene was performed to examine their potential for bacterial pathogenicity and probe their potential mechanisms of resistance to commercially available antibiotics.
Methods
Animal ethics. All methods were carried out following relevant guidelines and regulations. Handling of fish and all the experimental protocols carried out by well-trained scientists according to the guidelines of the Animal Ethics Review Committee of Suez Canal University. All used protocols are approved by Suez Canal University, Egypt.
Fish sampling. A total of 285 fish samples represented as 165 O. niloticus (70 apparently healthy and 95 moribunds) and 120 C. gariepinus (55 apparently healthy and 65 moribunds) were collected randomly; (5 sampling points at two months interval) from two private freshwater farms located at Ismailia Governorate, Egypt over the period of July 2018 to February 2019. The farms implemented semi-intensive culture system and applied the same management and treatment programs. Based on the clinical history, the farms routinely used different antibacterial agents such as amoxicillin, cefotaxime, tetracycline, norfoxacin, and gentamicin for regular prophylactic and treatment protocols. Fresh specimens of apparently healthy and moribund fish were transferred alive in aerated plastic bags to the laboratory of Microbiology, Faculty of Veterinary Medicine, Suez Canal University for further clinical and bacteriological examinations. Samples from liver, kidneys, and spleen were collected aseptically following the protocols of Yanong27.
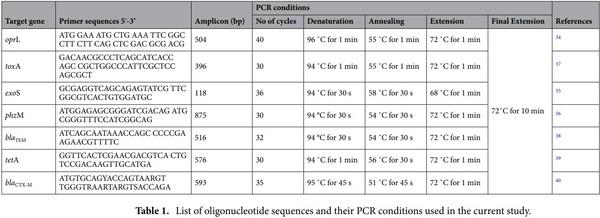
Clinical and postmortem inspections. Fish were clinically examined for the presence of external and internal lesions according to Austin et al.28. Briefly, the fish were examined in a sterile manner using a three-line incision in the case of O. niloticus or a V-shaped incision in the case of C. gariepinus. Antiseptic treatment of the skin was performed using 70% ethyl alcohol. The incision was carried out with sharp pointed scissors, which were introduced into the anus in such manner that the intra-abdominal point remained steady while the cut was made in close contact with the ventral side to avoid internal tissue damage. The abdominal wall was removed; the internal organs were exposed and examined macroscopically for any gross abnormalities.
Isolation and identifcation of P. aeruginosa. A loopful sample from the collected internal organs was directly streaked onto cetrimide agar and MacConkey’s agar (Oxoid, UK), and left incubated at 37 °C for 24 h under aerobic condition. The production of yellowish-green fluorescent pigment is commonly associated with Pseudomonads29. All suspected colonies were harvested and purified for phenotypic and biochemical characteristics. Briefly, all isolates were identified morphologically using Gram’s stain and biochemically using various biochemical tests; catalase, oxidase, urease, indole, methyl red, Voges Proskauer, citrate utilization, H2S production, mannitol fermentation, and gelatin hydrolysis, as well as for their motility using hanging drop technique according to Mac Faddin30. Furthermore, the identified isolates were confirmed using a species-specific set of primers (PaF: 5′-GGGGGA TCTTCGGACCTCA-3′; PaSR: 5′-TCCTTAGAGTGCCCACCCG-3′) targeting 16S rRNA gene of P. aeruginosa as described elsewhere by Spilker et al.31.
Antimicrobial susceptibility testing. The susceptibility of the retrieved isolates to different commercial antimicrobial agents (Oxoid), including ofloxacin (5 µg), amoxicillin (10 µg), cefotaxime (30 µg), tetracycline (30 µg), levofloxacin (5 µg), gentamicin (10 µg), norfoxacin (10 µg), tobramycin (10 µg), and colistin sulfate (25 µg) was evaluated using a disc diffusion method32. The selected antimicrobial agents are representatives of the drugs used commonly in the aquaculture sector in Egypt and were selected according to the National Antimicrobial Resistance Monitoring System records. The test was performed using Muller Hinton agar plates (Oxoid, UK) and the plates were incubated at 37 °C for 24 h. The test was conducted according to the instructions of the Clinical Laboratory Standards Institute (CLSI) criteria33.
Molecular typing of the virulence and antibiotic‑resistant genes of the isolated P. aeruginosa strains. DNA of purified strains was extracted using a silica-based membrane QIAamp DNA Mini kit (Catalogue no. 51304) according to the manufacturer’s instructions. Genomic DNA templates were quantified using Nanodrop (Nanodrop 1000, Thermo Scientific, UK), adjusted to 100 ng μL−1, and stored at −20 °C until used for PCR. Ninety representative P. aeruginosa strains (the same strains that were tested for the antimicrobial susceptibility) were tested for the detection of virulence genes, four sets of primers targeting (oprL, exoS, phzM, and toxA) genes were selected based on the previous publications of Xu et al.34, Winstanley et al.35, Finnan et al.36, and Matar et al.37, respectively. Further, to verify the resistance of retrieved strains to the commercially available antibiotics, three sets of primers targeting blaTEM, tetA, and blaCTX-M genes were also selected according to Colom et al.38, Randall et al.39, and Fazeli et al.40, respectively. All primers supplied by (Metabion Company, Germany), and their oligonucleotides sequences and PCR conditions are given in Table 1. PCR reactions (25 μL) were amplified in T100 Gradient Thermocycler (Biometra, Jena, Germany) using EmeraldAmp GT PCR Master Mix (Code No. RR310A, Takara, Japan). A reaction with no template DNA was used as a negative control, while a virulent reference strain of P. aeruginosa (multidrug-resistant to amoxicillin, cefotaxime, tetracycline, and gentamicin), kindly provided by Animal Health Research Institute in Dokki, Cairo, Egypt, was used as positive control. The products were screened by horizontal 1.5% (w/v) agarose gel electrophoresis (AppliChem GmbH, Darmstadt, Germany) and then photographed.
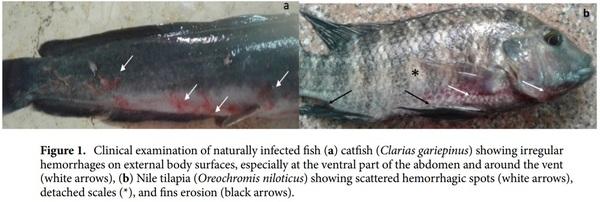
Pathogenicity test. Acclimation period. A total of 150 healthy O. niloticus weighing 45±3 g with no history of previous infections were collected randomly from Fish Research Center, Suez Canal University, Egypt, and left acclimated in a clean fiberglass tank of 1 m3 holding capacity for 15 days prior to the challenge. Oreochromis niloticus was selected as a model for the present study due to its local availability and ease of cultivation, handling, and transportation. The tank was filled with sand-filtered, UV-sterilized, dechlorinated tap water with an average salinity of 0.3±0.1 g L−1. Dissolved oxygen was monitored at 5±1 mg L−1 using automatic air suppliers (RINA, Genova, Italy), while the water temperature was maintained at 27±0.52 °C. Tank pH was regulated at 7.5 and 13 h light/11 h dark cycle was adopted. Ammonia and nitrite were measured twice a week and never exceeded 0.05 and 0.25 mg L−1, respectively. The fish were fed two times daily (09:00 and 20:00 h) until visual satiety on a commercial pellet of 30% crude protein (Skretting, Alexandria, Egypt). The organic wastes and other debris were siphoned and 30% of the water was replaced daily to reduce the toxicity of ammonia. Fish that showed normal reflexes with no apparent lesions selected for the challenge trial.
Challenge trial. It was performed according to the directive on the protection of animals used for scientific purposes and following the ethical approval sheet mentioned above. One hundred and twenty of acclimated O. niloticus were equally distributed into three groups; each contributed 2 glass aquaria of 80 L and 20 fish holding capacity. The trial was performed in duplicates (n=2). The fish of the first group (C) were injected intraperitoneally (IP) with 100 µL of sterile phosphate buffer saline and served as a control, while the fish of the other groups (T1 and T2) were injected with 100 µL of the overnight culture of virulent P. aeruginosa strains (A and B, respectively) at a concentration of 3×107 cells mL−11 . The isolates were selected based on the fact that all recovered isolates have identical biochemical and molecular properties. One of these isolates (A) harbored oprL, toxA, and phzM virulent genes, while the other (B) just encoded the oprL and toxA genes. For inocula preparation, bacteria were routinely cultured on tryptic soy broth (Oxoid) at 37 °C for 24 h. Thereafter, the growing bacteria were adjusted to the desired concentration using 0.5 McFarland standards and by the Helber counting chamber. The pathological lesions and cumulative deaths were recorded daily among experimental groups for 14 days post-challenge. Moribund and freshly dead fish were collected, examined immediately to verify the cause of death. Mortalities were considered only when the injected strain was recovered from the experimentally infected fish (Koch’s postulates).
Statistical analysis. The Chi-square was carried out to analyze the data to test the null hypothesis of various treatments using the statistical analysis software (SAS, Sofware version 9.4, SAS Institute, Cary, NC). The signifcance level was (P< 0.05).
Results
Clinical and postmortem findings. In the present study, a total of 285 fish samples represented as 165 O. niloticus and 120 C. gariepinus were collected randomly and examined. The clinical inspection revealed that 95 O. niloticus (95/165, 57.5%) and 65 C. gariepinus (65/120, 54.1%) were moribund and showed the signs of hemorrhagic septicemia. Regardless of the fish species, most of the naturally infected fish shared the same typical clinical signs, including hemorrhages on external body surfaces, mainly at the ventral aspect of the abdomen and around the vent (Fig. 1a). Others showed fins erosions, skin darkness, and detached scales (Fig. 1b). Internally, the infected fish showed typical signs of hemorrhagic septicemia represented by pale liver, necrotic gills, engorged spleen, and abdominal dropsy with reddish ascetic exudates (Fig. 2a,b).
Bacteriological assay. The bacteriological examination revealed that all retrieved isolates were motile, Gram-negative bacilli, arranged in double or short chains. The colonies reacted positively to catalase, oxidase, nitrate reduction, gelatin hydrolysis, citrate utilization, and mannitol fermentation, while they were negative for H2S production, urease, Voges Proskauer, indole, and methyl red. Based on the morphological and biochemical characteristics, all isolates were identified as P. aeruginosa. The typical isolates of P. aeruginosa displayed large irregular colonies with a fruity odor and produced a yellowish-green fluorescent pigment on cetrimide agar (C.A) at 37 °C for 24 h. The bacteria grew on MacConkey’s agar and showed fat, smooth, non-lactose fermenting colonies with regular edge and alligator skin like from the top view. Furthermore, all isolates were positive
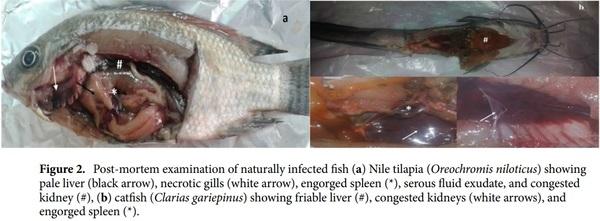
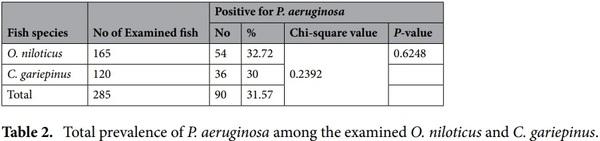
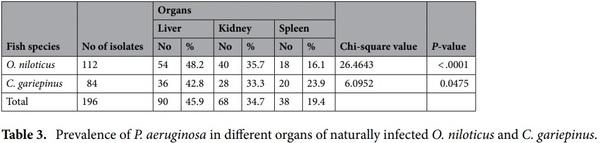
for the PCR amplification of species-specific 16S rRNA gene. The total prevalence of P. aeruginosa among the examined fish was 31.57%. The highest prevalence was recorded in O. niloticus (54/165, 32.72%) followed by C. gariepinus (36/120, 30%). None of the examined apparently healthy fish yielded P. aeruginosa, moreover, P. aeruginosa was isolated from 54 moribund O. niloticus (54/95, 56.8%) and 36 moribund C. gariepinus (36/65, 55.4%). The statistical analysis demonstrated that there was no significant difference in the prevalence of P. aeruginosa in O. niloticus and C. gariepinus (P> 0.05) (Table 2). Regarding the prevalence of P. aeruginosa in various infected organs, the liver was the most prominent infected organ, followed by the kidney and spleen (Table 3). The presence of P. aeruginosa in at least one organ of the fish was considered positive for the bacterium. The statistical analysis showed a significant difference in the prevalence of P. aeruginosa among the internal organs of the examined fish (P< 0.05).
Antibiogram of the isolated P. aeruginosa strains. The antimicrobial susceptibility testing was conducted on 90 representative isolates of P. aeruginosa (one isolate/each infected fish; isolated from the liver). As shown in Table 4, the tested strains showed different resistance patterns to various antimicrobial agents (P< 0.0001). The examined strains were entirely sensitive to colistin sulfate (100%), highly sensitive to norfoxacin (88.89%), while exhibited remarkable resistance to amoxicillin (83.3%), cefotaxime (77.7%), tetracycline (75.6%), and gentamicin (67.6%). Additionally, out of them, 50 strains (50/90, 55.5%) showed multi-drug resistance to four antimicrobial agents; amoxicillin, cefotaxime, tetracycline, and gentamicin (Table 6).
Molecular typing of the virulence and antibiotic‑resistance genes of the isolated P. aeruginosa strains. Several antibiotic-resistance and virulence genes associated with a natural outbreak of P. aeruginosa were selected based on the previous publications and were examined in ninety representative P. aeruginosa strains (the same strains that were tested for the antimicrobial susceptibility). The results showed that all tested strains harbored oprL gene (100%) with specific amplicons size of 504 bp (Fig. 3, Table 5), as well as toxA gene (100%) with amplicons size of 396 bp (Fig. 4, Table 5), while 20 strains (22.2%) were positive to the phzM gene with fragments size of 875 bp (Fig. 5, Table 5). On the contrary, none of the tested P. aeruginosa strains were
positive for exoS gene (Fig. 6, Table 5). The statistical analysis revealed a significant difference in the prevalence of various virulence genes among the tested strains (P< 0.0001).
Concerning the distribution of the antibiotic resistance genes, 75 strains (83.3%) were positive for the blaTEM gene with a specific amplicon size of 516 bp, while 68 strains (75.6%) harboring tetA gene with a specific amplicon size of 576 bp, in addition, 70 strains (77.7%) harboring the blaCTX-M gene and gave specific amplicon size of 593 bp (Table 5, Figs. 7,8 and 9).
In the present study, as illustrated in Tables 4 and 6; the isolated strains exhibited a remarkable resistance to amoxicillin (83.3%), cefotaxime (77.7%) tetracycline (75.6%), and gentamicin (67.6%). Furthermore, Fify strains (50/90, 55.5%) showed multi-drug resistance to four antimicrobial agents: amoxicillin, cefotaxime, tetracycline, and gentamicin and harbored blaTEM, blaCTX-M, and tetA antimicrobial resistance genes. Out of which,
forty-one strains harbored two virulence genes (oprL and toxA), while nine strains harbored three virulence genes (oprL, toxA, and phzM). The statistical analysis revealed a non-significant difference in the prevalence of various antimicrobial-resistance genes among the tested strains (P > 0.05). The distribution of the multi-drug resistance patterns, antimicrobial resistance genes, and virulence genes among the tested P. aeruginosa strains (n=90) illustrated in Table 6.
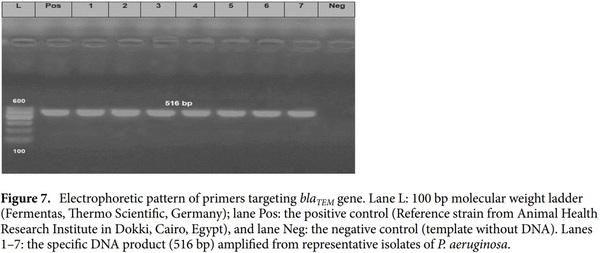
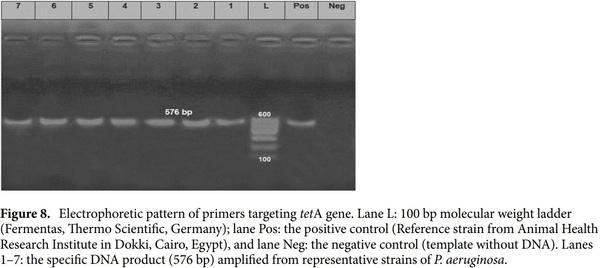
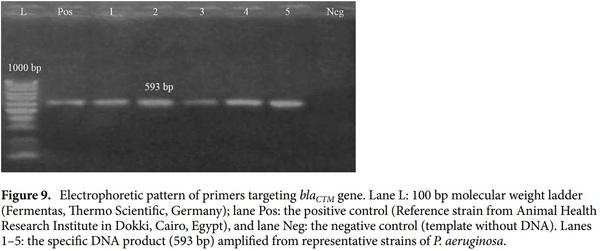
Pathogenicity (Challenge trial). The clinical signs, as well as fish morbidity and mortality, were recorded in all experimental groups for 14 days post-challenge. The results demonstrated that the fish of the control group did not reveal any mortalities or pathological lesions, while those of the other groups displayed high mortalities and pathognomonic lesions of hemorrhagic septicemia, similar to those reported in naturally infected fish. It was noted that cumulative deaths were relatively associated with encoded virulence genes (Fig. 10), where the maximum mortality rate (87.5%) was recorded in T1 group, followed by T2 group (67.5%). Indeed, O. niloticus inoculated with a virulent strain A produced higher mortalities in a shorter time compared to those exposed to strain B. Approximately, 87.5% of infected fish in T1 group died within 7 days post-inoculation, while those of T2 group showed delayed mortality (67.5%) up to 12 days post-inoculation. Clinically, most of the experimentally infected fish showed exophthalmia, abdominal ascites, hemorrhagic gills, detached scales, and skin ulcers.
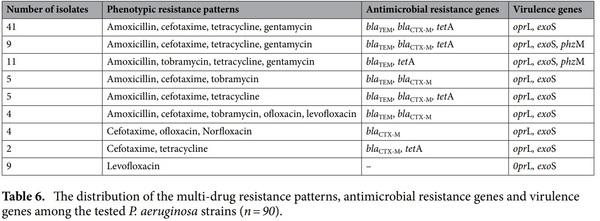
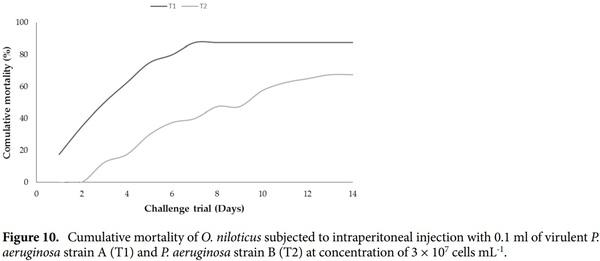
The postmortem findings revealed that the challenged fish displayed typical signs of septicemia manifested by congested liver, enlarged spleen, and accumulation of serous bloody fluid in the abdominal cavity. In terms of bacteriology, P. aeruginosa was successfully isolated from skin ulcers and internal organs of dead and moribund fish, and the results confirmed that all isolates belong to P. aeruginosa based on biochemical characteristics and molecular typing.
Discussion
Pseudomonas aeruginosa is a ubiquitous pathogen and is one of the principal causes of septicemia in freshwater fish, resulting in tremendous economic losses in fish producing sectors worldwide41. In the present study, a total of 285 fish samples (165 O. niloticus and 120 C. gariepinus) were collected randomly from private freshwater farms at Ismailia Governorate, Egypt, for clinical and bacteriological examinations. The results of clinical inspection revealed that 95 O. niloticus (95/165, 57.5%) and 65 C. gariepinus (65/120, 54.1%) were moribund and exhibited the typical signs of hemorrhagic septicemia, skin ulcerations, and fn rots. Moreover, internally the infected fish showed pale liver, engorged spleen, and abdominal dropsy with reddish ascetic exudates. These findings agreed with those obtained by Eissa et al.1 and Magdy et al.42 who reported that the postmortem findings due to P. aeruginosa infection were ascites, hepatic and renal necrosis, and congestion of all internal organs. The disease induced by P. aeruginosa is mainly associated with exophthalmia, skin discoloration, hemorrhage, detached scales, and abdominal distension43. Concerning the phenotypic characteristics of P. aeruginosa, all the isolated strains exhibited the typical phenotypic characteristics, culture characters, and biochemical characteristics of P. aeruginosa. These findings are nearly similar to those obtained by Aprameya44.
In the present study, P. aeruginosa isolated from the examined fish with a prevalence of 31.57%. The highest prevalence recorded in O. niloticus (32.72%) followed by C. gariepinus (30%), in agreement with Magdy et al.42 who recorded that the prevalence of P. aeruginosa in O. niloticus and C. gariepinus was 34.4% and 27.5%, respectively. Interestingly, none of the apparently healthy fish yielded P. aeruginosa, while the bacteria recovered from all moribund O. niloticus and C. gariepinus with a total prevalence of 56.8% and 55.4%, respectively. Regarding the distribution of P. aeruginosa in various internal organs, the liver was the most prominent infected organ, followed by the kidney and spleen, which is consistent with those reported by Eissa et al.1. Variations in prevalence could be related to the geographical distribution, environmental factors, host susceptibility, and the season of sample collection. Regarding the antimicrobial susceptibility testing, the examined strains were sensitive to colistin sulfate (100%), followed by norfoxacin (88.89%), while showed remarkable resistance to amoxicillin (83.3%), cefotaxime (77.7%) tetracycline (75.6.8%), and gentamicin (67.6%). Furthermore, Fify strains (50/90, 55.5%) showed multi-drug resistance to four antimicrobial agents: amoxicillin, cefotaxime, tetracycline, and gentamicin and harbored blaTEM, blaCTX-M, and tetA antimicrobial resistance genes. Out of which, forty-one strains harbored two virulence genes (oprL and toxA), while nine strains harbored three virulence genes (oprL, toxA, and phzM). These findings consistent with those recorded by Eid et al.45 and Nasreen et al. 46 who reported the resistance to tetracycline and gentamycin. Globally, several antimicrobial agents are frequently used for the treatment and/ or prevention of fish bacterial diseases. The indiscriminate use of antibiotics, as well as the emerging antibiotic resistance genes, could result in the occurrence of multi-drug resistant (MDR) strains47–50. Therefore, the routine application of antibiotic sensitivity testing is significant to select the specifically effective antibiotic and overcome such a problem51–53.
In the present study, the PCR results revealed that all the tested strains were positive for oprL and toxA genes, in agreement with Kenneth54. L- Lipoproteins refer to the outer membrane proteins associated with P. aeruginosa that enable the microorganism to resist the antiseptics and variable antimicrobial agents. L- lipoproteins are restricted to Pseudomonads, so it could be a reliable target used in both identification and virulence determination of Pseudomonads in clinical specimens55. Exotoxin A is an extracellular product of virulent P. aeruginosa that is encoded by the toxA gene in their chromosome. It acts by inhibition of protein-biosynthesis in the host cell, resembling the action of diphtheria toxin56.
On the contrary, none of the tested strains was positive for the exoS gene, while 20 strains were positive to the phzM gene. These results agreed with those recorded by Nowroozi et al.57. The presence of the phzM gene in 22.2% of the examined strains indicates their ability to produce a phenazine toxin, which in turn enhances their survival rate and colonization to the host even under adverse environmental circumstances58,59. Concerning the distribution of the antibiotic-resistance genes: the examined strains harbored blaTEM, blaCTX-M, and tetA genes with a total prevalence of 83.3%, 77.7%, and 75.6%, respectively, in agreement with Ndi and Barton60, and Ishida et al.61. The presence of these genes explains the phenotypic resistance of the tested P. aeruginosa strains to cefotaxime, amoxicillin, and tetracycline62.
Regarding the pathogenicity trial, fish challenged with P. aeruginosa provided high mortality rates in direct proportion to the encoded virulence genes and showed similar signs of septicemia found in the naturally infected one. Our results are in a good agreement with Magdy et al.42 who noticed marked histopathological alterations in C. gariepinus experimentally challenged with P. aeruginosa. The present findings paralleled with those reported by Devakumar et al.63 who observed degenerative changes in all tissues (brain, ovary, liver, and gills) of crabs infected with P. aeruginosa. Likewise, Derwa et al.64 demonstrated that O. niloticus experimentally infected with P. aeruginosa clinically suffered from exophthalmia, scale losses, skin ulcerations, and external hemorrhages over all the body surfaces and at the base of fins, while, internally they showed a distended gall bladder, congestion, and enlargement of liver, spleen, and kidneys. The degenerative changes could be related to the fatal and subversive influence of bacterial toxins, enzymes, and bioactive extracellular components, which promote tissue damage and cell necrosis23. The elaboration of exotoxins: groups of proteins, is the most crucial factor in P. aeruginosa pathogenicity. The exotoxins are proven to induce liver necrosis, hemorrhage, and renal nephrosis65.
In conclusion, P. aeruginosa is one of the major recurrent emerging pathogens frequently isolated from O. niloticus and C. gariepinus. The recovery of multi-drug resistant (MDR) strains of P. aeruginosa gave a warning to the potential and proper use of antibiotics. The most frequent antibiotic-resistance genes associated with P. aeruginosa isolated from fish are blaTEM, tetA, and blaCTX-M genes that induced resistance patterns to amoxicillin, tetracycline, and cefotaxime, respectively. Routine application of the antimicrobial susceptibility testing is necessary to prevent the emergence of antibiotic-resistant strains of potential public health concern. oprL and toxA genes are the most predominant virulence genes associated with P. aeruginosa.