Summary
Modern-day broilers are capable of converting dietary feed ingredients into chicken-meat at ratios approaching 2:1 and constitute a more ecologically sustainable protein source for uman onsumption than alternative sources. Similarly, as discussed, chickenmeat production emits less CO2-equivalent greenhouse gases than most alternative animal protein industries. Clearly urther improvements in feed conversion ratios of broiler chickens are highly desirable from both environmental and economical standpoints. These desirable effects are mediated via both irect and indirect mechanisms. Direct mechanisms include reduced faecal N and P losses with obvious beneficial effects on local environments. Indirect benefits include alleviation of ressure on agricultural land, commodity supply and a reduced need for water. The implications of these concepts are discussed herein as well as a selection of strategies by which FCR ay be controlled.
Introduction
"Professor Ross Garnaut pointed out an interesting inverse relationship this week. Over the past three years the science of global warming has become more clear and more alarming, but in the same period, fewer Australians believed it was true." These two sentences were written by Lenore Taylor in the Sydney Morning Herald of March 12 2011. Clearly, the debate about "global warming" or "climate change" has become both emotive and highly politicised. Nevertheless, it is irrefutable that meat- roducing industries have tangible impacts on the environment, including greenhouse gas emissions, all of which would be attenuated by improvements in feed conversion ratio (FCR). That the production of poultry products has a relatively low environmental footprint is of relevance and will be discussed herein.
Background
In 2007, the Food and Agriculture Organisation (FAO) estimated that world poultry meat production was 86.2 million tonnes and according to the Australian Bureau of Agricultural and Resource Economics (ABARE,2008), Australian poultry meat production was 845,000 tonnes. Thus, Australian poultry meat production represents about 1% of global poultry meat production. Despite our small share of the global output, per capita consumption of chicken is high in Australia (40 kg per capita per year) and chicken has overtaken beef as the meat of preference. To meet consumer demand, about 520 million chickens are processed yearly. At an average carcass weight of 1.75 kg, this corresponds to 910,000 tonnes of chicken meat or, assuming a 72 % yield, 1.2 million tonnes live weight. Assuming an overall FCR of 1.8, this equates to an annual feed requirement of 2.3 million tonnes for broiler chickens alone, excluding broiler breeders. This in turn translates to 1.3 million tonnes of cereal grain (mainly wheat and sorghum) and 0.5 million tonnes of soybean meal, which is imported from Brazil and Argentina. At a cost of $415 per tonne for a broiler diet, the cost of feed for the local broiler industry is $944 million, which represents up to 70% of the total cost of production. Hence, quite apart from ecological considerations, a 5% improvement in FCR represents a financial saving of $47 million to the Australian industry, a saving that would be a hundred-fold greater if applied to global poultry meat production.
Greenhouse gases
According to a 2006 FAO report, meat production is responsible for 14–22% of the 36 billion tonnes of CO2- equivalent greenhouse gases produced globally each year (Fiala, 2009). It is predicted that global consumption of poultry meat will increase between 2000 and 2030 at an average annual rate of 2.51%, compared with 1.85% for pork and 0.92% for beef (Fiala, 2008). These trends would ameliorate greenhouse gas emissions from meat production because the production of 1 kg of poultry meat generates 1.1 kg of CO2 equivalents as opposed to 3.8 kg of CO2 equivalents for pork and 14.8 kg of CO2 equivalents for beef (Fiala, 2008). If Australians consumed only poultry meat, and no beef or pork, then the output of CO2 equivalents as a result of meat production would decrease by 84% and total greenhouse gas emissions for Australia would be reduced by 15%. Thus, although largely based on price, the increasing preference of Australian consumers for chicken over beef is, albeit unwittingly, generating a decline in greenhouse gas emissions. However, although the poultry industry has an advantage in a relatively low 'footprint' from a greenhouse gas point of view, the use of such information to gain an advantage over other animal protein industries is ill advised. In the opinion of the authors, solidarity rather than competition is required in the various animal protein sectors, i.e., chicken, lamb, beef, pork and fish. Ultimately, marketing of animal protein to the public based on sustainability metrics is likely to result in a reduction in the size of the global animal industry, a loss of jobs in rural areas and a stronger emphasis on vegetarian and vegan options.
Phosphorus
Although the environmental impact of greenhouse gases has received considerable scrutiny, it is not possible to over-emphasise the ecological significance of phosphorus (P). P is a critical nutrient in respect of a myriad of biochemical processes and skeletal integrity in food-producing animals and in consumers of animal products. Crucially, analogous to the state of oil and petroleum reserves, global reserves of rock phosphate are not renewable. The world is now faced with a P crisis driven by declining global reserves of rock phosphate (Abelson, 1999). Indeed, it has been predicted that P production will peak prior to 2040 and that commercially viable rock phosphate deposits will be depleted in less than 100 years (Cordell et al., 2009). Furthermore, new sources of phosphate are increasingly of poor or variable quality and may be contaminated with trace minerals such as magnesium.
Australian broiler diets contain 17,000 tonnes of P from a range of feedstuffs. If half of this amount were derived from dicalcium phosphate (DCP), at a P content of 180 g/kg, 47,000 tonnes of DCP would be required. While the finite reserves of rock phosphate are being depleted, the P that is included in poultry diets is poorly utilised. Moreover, non-retained P that is lost in effluent from pig and poultry production units contributes to the eutrophication of freshwater reserves, which is an environmental concern (Daniel et al., 1998). The majority of P in feed ingredients of plant origin is phytatebound and is only partially available to monogastrics, largely because of calcium (Ca) levels in diets and the insolubility of Ca–phytate complexes formed de novo in the gut (Wise, 1983). However, phytate-P can be liberated by phytate-degrading enzymes to enhance P digestibility, and phytase enzymes are now commonly included in poultry diets (Selle and Ravindran, 2007). In the landmark study of Simons et al. (1990), an Aspergillus niger-derived phytase reduced P excretion in broiler chickens by up to 47%, which attenuates P pollution of the environment by the poultry industry.
Selection for feed efficiency
Zhang and Aggrey (2003) reviewed the genetic variation in feed efficiency of broiler chickens. As demonstrated by Havenstein et al. (2003), the growth performance of broiler chickens has been enhanced over the past 50 years, mainly because of genetic selection. However, it appear that more emphasis was placed on weight gain than on efficiency of feed conversion during this period (Table 1).
Table 1. Comparative growth performance of Ross 308 male broilers (2001 strain) and Athens-Canadian Randombred Control male broilers (1957 strain) offered chronologically appropriate diets (Havenstein et al., 2003).
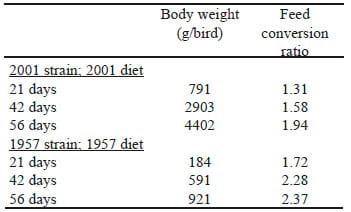
At 42 days of age, 2001 broilers offered contemporary diets had a body weight of 2903 g compared with 591 g for their 1957 counterparts, a five-fold difference in growth rate. In contrast, the improvement in 42-day FCR was a relatively modest 30.7% (2.28 vs 1.58 for 1957 vs 2001 broilers, respectively). However, it may be deduced from this study that at a body weight of 2.0 kg, the 2001 birds would have a feed efficiency of 1.48 in comparison to 3.89 for their 1957 counterparts (Figure 1). Thus, on the basis of weight-corrected FCR, the improvement in feed efficiency is 62.0% and the average annual improvement is 0.98%. It is noteworthy that a 2 kg broiler with an FCR of 1.48 would be converting 2.11 kg of feed into 1 kg of chicken meat in 2001. Presently, it is likely that a proportion of broiler chickens that are processed at 35 days of age convert less than 2 kg of feed into 1 kg of chicken meat, which is far superior to the FCR for pork and beef.
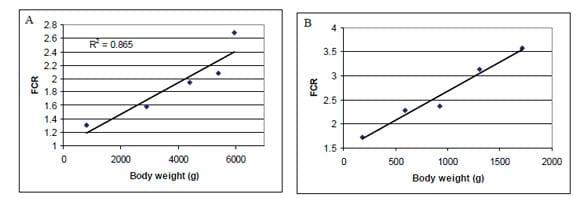
Figure 1. Relationships between body weight and feed conversion ratio in 2001 strain Ross 308 male broilers (Figure 1A) and 1957 strain Athens-Canadian Randombred Control male broilers (Figure 1B) offered chronologically appropriate diets (Havenstein et al., 2003).
Table 2. Comparison of broilers selected for poor or good feed conversion efficiency (adapted from Aggrey et al., 2008)
whereas the retention and digestibility of protein and Ca were superior in the 'good converters'. Thus, the data of Aggrey et al. (2008) indicate that digestion of protein and absorption of amino acids are limiting factors in the 'poor converters', which is not the case for starch digestion and glucose absorption. Similarly, there is an association between more efficient feed conversion and enhanced digestibility and retention of Ca, which does not apply to P.
Calcium (or limestone) – a possible 'anti-FCR factor'
The association between Ca and enhanced feed conversion is of interest. Ca concentrations in the lumen of the gastrointestinal tract were lower in the 'good converters' in the Aggrey et al. (2008) study. It is tempting to speculate that the additional Ca in the gut of 'poor converters' may have contributed to their inferior feed efficiency and protein digestibility. In general, most dietary Ca is present as limestone, which has an extremely high acid binding capacity and tends to increase the pH of digesta (Shafey et al., 1991).
High Ca concentrations in the gut may increase the extent of de novo ternary protein–phytate complex formation in the small intestine, in which protein and phytate are linked by Ca2+, which serves as a cationic bridge between the two moieties. Presumably, the quantity of protein that can be bound by Ca2+ and phytate in ternary complexes is dependent on the molecular weights of proteins and polypeptides in the small intestine. Montagne et al. (2003) determined the molecular weights of proteins along the small intestine of pre-ruminant calves. In diets containing soy protein concentrates or isolates, nearly 60% of protein in ileal digesta had a molecular weight in excess of 20,000 Daltons. Consequently, it appears that protein binding as ternary protein–phytate complexes in the small intestine may be sufficient to disrupt protein digestion and amino acid absorption.
Perhaps of greater importance is the ability of Ca to interact directly with protein, including soy protein (the coagulation of soy protein by Ca is an integral step in the preparation of tofu). Kroll (1984) reported that Ca2+ has the capacity to bind soy protein via the side-chain carboxyl groups of aspartic acid and glutamic acid and via the imidazole group of histidine. Interactions between Ca2+ and the 11S fraction of soy protein have been investigated (Sakakibara and Noguchi, 1977). Ca has the capacity to coagulate soy protein (Torikata et al., 1987) and reduce soy protein solubility (Gifford and Clydesdale, 1990). More recently, Canabady- Rochelle et al. (2009) investigated Ca interactions with soy protein using isothermal titration calorimetry, in which Ca-induced soy protein aggregation may be irreversible, depending on the pH. In addition, Ca may react with fatty acids to form Ca-soaps in the gut lumen, which may limit the utilisation of energy from lipids, particularly saturated fatty acids (Leeson, 1993). Finally, Ca also affects the phytate/phytase axis in that Ca–phytate complex formation renders the substrate less susceptible to enzyme degradation by phytases (Selle et al., 2009).
Feed form and processing
The transition from mash to steam-pelleted broiler diets has enhanced feed efficiency. As reviewed by Jensen (2000), broilers across five studies that were offered pelleted diets converted feed 3.63% more efficiently than their counterparts that were fed mash diets (1.86 vs 1.93, respectively). However, on a weight corrected- FCR basis, the improvement was 7.92% (1.86 vs 2.02, respectively). Much of this advantage can be attributed to less overall wastage of feed in pelleted form coupled with the fact that pelleted feed facilitates prehension. Jensen et al. (1962) found that under a 12-hour lighting protocol, 21 to 28-day-old broilers offered mash diets spent 14.3% of the time eating 38 g of feed during a 12- hour day. Broilers offered pelleted diets consumed 37 g of feed, but in 4.7% of the time, simply because pellets are "easier-to-eat" than mash or because prehension is facilitated.
However, as championed by Cumming (1994), an emerging strategy is to offer broilers whole grain in addition to a pelleted concentrate. The provision of whole grain has economic advantages from a feedmill production standpoint and is thought to enhance gut integrity. Whole grain feeding stimulates gizzard development and function and increases gizzard weight. Several studies have assessed the effect of whole grain feeding on feed efficiency in broilers and a total of 17 studies were indentified in which the median response was an improvement of 2.93% in FCR. However, in 13 ex 17 studies, positive improvements in FCR ranging from 0.13% to 12.50% were recorded. For example, Wu et al. (2003) found that 20% whole wheat improved weight gain by 2.1% (818 vs 801 g/bird) and feed efficiency by 5.6% (1.496 vs 1.590) in broilers up to 21 days of age. In addition, Plavnik et al. (2006) recorded improvements of 3.9% in weight gain (2525 vs 2431 g/ bird) and 5.8% in feed efficiency (1.815 vs 1.927) in broilers from 6–46 days post-hatch using 25% whole grain. More recently, Svihus et al. (2010) compared 15% whole wheat and a proprietary diet with a ration in which 15% ground wheat was incorporated into cold pellets with the proprietary diet in broilers from 16 to 25 days. The whole wheat regime improved feed efficiency by 12.5% (1.563 versus 1.786) in this comparison in association with reduced feed intakes. Consequently, there are tangible indications that whole grain feeding and improved gizzard function enhances feed efficiency.
The separate provision of part of the dietary grain component as whole grain and the feeding of a 'twoway' diet (whole grain plus the balance of the diet in pelleted form) is a practice that has been adopted by the majority of broiler integrators in Australia. However, the Poultry Research Foundation is investigating the feasibility of a 'three-way' diet in which calcium (Ca), as shell-grit limestone, is a third component in addition to whole grain and the pelleted concentrate. Depending on feeding patterns, the separate provision of Ca may permit broilers to consume the balance of their diet with relatively low Ca concentrations in the gut, which may improve feed efficiency, while maintaining a selfregulated Ca intake that is adequate to ensure skeletal integrity. This project is in its infancy but preliminary results are encouraging.
Dietary manipulation for feed efficiency
The addition of in-feed antibiotics (IFAs) to broiler diets usually generates improvements in feed efficiency. For example, an Australian study (Pensack et al., 1982) found that 10 mg/kg of avoparcin, a glycopeptide antibiotic, improved feed efficiency by 11.4% (2.71 vs 2.40) in 63-day-old broilers. However, more conservative improvements in feed efficiency (2.7– 4.1%) were recorded in 28 American and European studies. Lewis and O'Beirne (1994) compared the gross energy required to manufacture avoparcin with the saving in gross energy that accrues from its inclusion in diets for food-producing animals and argued that the use of avoparcin was 'very beneficial' in the context of energy conservation. Interestingly, avoparcin was more advantageous in this respect when included in broiler chicken diets than when included in diets for pigs or cattle. Nevertheless, the use of avoparcin as an IFA has been discontinued in several countries, including Australia, because it is a vancomycin analogue. The incidence of vancomycin-resistant enterococci (VRE) among chicken farms in Taiwan was monitored between 2000, when avoparcin was banned, and 2003. Lauderdale et al. (2007) reported that the incidence of VRE declined from 25% (15/60 farms) in 2000 to 8.8% (7/80 farms) in 2003 because of the discontinuation. The use of IFAs was banned in Europe in 2006; consequently, although IFAs have the potential to improve FCR, it is increasingly unlikely that chicken-meat producers will be able to take advantage of them.
The timetable for the impending demise of IFA is unpredictable; nevertheless, it has triggered a frenetic quest for alternative feed additives to enhance feed efficiency. However, the efficacy of the majority of alternatives evaluated is questionable. Organic acids are widely used in weaner pigs, perhaps more because of their acidifying effect than because of their antimicrobial activity. Potassium diformate, which dissociates into formic acid and potassium formate in the gut, was evaluated in Australian weaner pigs. It improved feed efficiency by 7.6% (1.33 vs 1.44) and weight gain by 23.7% (266 vs 215 g/day) at an inclusion rate of 18 g/kg (Selle et al., 2003). However, the outcomes of addition of potassium diformate to poultry diets as a growth promotant (Selle et al., 2004) or as a treatment for broilers challenged with necrotic enteritis (Mikkelsen et al., 2009) were inconsistent. Organic acids, like IFAs, possess antimicrobial activity and it is claimed that 'favourable manipulation of the gut microflora' is an important mode of their action. Although this premise has met with acceptance, its validity is open to challenge.
Feed enzymes are not usually considered replacements or alternatives to IFAs, probably because they do not directly influence the gut microflora. Nevertheless, both phytate-degrading enzymes (Selle and Ravindran, 2007) and non-starch polysaccharide (NSP)-degrading enzymes in diets based on 'viscous grains' (Choct, 2006) have the capacity to enhance feed efficiency in broilers. However, in Australia, the inclusion of phytate-degrading enzymes in wheatand sorghum-based broiler diets and NSP-degrading enzymes in wheat-based diets is now a routine practice. The possibility remains that advances in enzyme technology and the practical application of feed enzymes will permit further improvements in feed efficiency.
Ca and phytate interact in the gut to form Ca–phytate mineral complexes and phytate may have the capacity to bind one-third of dietary Ca (Selle et al., 2009). Thus, dietary inclusion of phytase will effectively liberate Ca by preventing the formation of these complexes. It is likely that the Ca equivalence of phytase is presently underestimated, perhaps more so at high inclusion rates. Lower Ca levels in phytase supplemented-diets, accompanied by appropriate reductions in P level, may enhance feed efficiency responses to phytase.
Formulation of poultry diets to meet nutrient requirements is a key objective of good nutritional practice. However, until recently, many poultry diets were formulated on a total amino acid basis rather than on a digestible amino acid basis. Formulation of poultry diets on a digestible amino acid basis is unequivocally advantageous, as it improves both FCR and the environmental impact. One of the major advantages of formulation on a digestible amino acid basis, especially when used in conjunction with synthetic amino acids (lysine, methionine and threonine), is that it facilitates a reduction in dietary crude protein concentration. This reduction improves feed efficiency, as the amount of surplus amino acids that the bird must deconjugate and excrete is reduced. Similar benefits exist for phase feeding, a strategy that aims to meet changes in nutrient requirements as the bird ages.
An approach that has been adopted to some extent in pigs as a means of improving feed efficiency is the formulation of diets on the basis of net energy (NE) rather than digestible energy. A comparison of NE versus metabolisable energy for formulating broiler diets is a core objective of the current Australian Poultry Cooperative Research Centre.
Ion Balance and Hofmeister Effects
The lyotropic or "Hofmeister" series is a biochemical grouping of anions and cations based on their ability to precipitate protein (Collins and Washabaugh, 1985). These ion-specific effects are thought to be associated with changes in the thermodynamics of the water matrix where the strongly hydrated anions and weakly hydrated cations act as 'stabilizing agents' (kosmotropes) and the weakly hydrated anions and strongly hydrated cations act as 'destabilizing agents' (chaotropes). Media that contains high concentrations of kosmotropic anions and cations results in increased protein precipitation due to a reduction in 'free' water concentrations and vice versa for the chaotropic ions. These effects are complex and involve interactions with pH and temperature, and the hyhobicity or polarity of the interacting surface (Zhang and Cremer, 2010). The mechanisms behind these effects are not clear and the reason for this obscurity is the complex ion-specific interactions that occur i.e. difficulty in separating opposing forces of the chaotropic and kosmotropic anions and cations and also sensitivity in methodology to explore the effects on water structure (Evans and Niedz, 2008). However, data does exist that suggests ions influence hydrogen bonding in water, altering 'bulk' water structure. In this context ions which exhibit weaker interaction with water than is the case for water to water, are known as chaotropic and those that exhibit stronger interactions with water than does water to itself are known as kosmotropic (Collins and Washabaugh, 1985). Hofmeister effects are well represented in the scientific literature and have been explored in various disciplines including soil chemistry, protein chemistry, water and solute chemistry (Evans and Niedz, 2008) and even lyotropic effects in insect physiology. However, to the authors knowledge there have been no reports on the effects of 'ion-soups', from a lyotropic perspective, on nutritional biochemistry and in particular, mineral and amino acid digestibility and the effect on FCR.
Avian diets are an 'ion soup' containing variable concentrations of carbonates, sulphates, nitrogenous compounds as well as various salts such as NaCl, NaHCO3 and metal ions such as K, Mg, Fe, Zn, Cu, Mn, Ca and Sr and non-metal ions such as P, S, Cl, Se and N.
Figure 2 Representation of Hofmeister anions with increasing chaotropic potency from left to right (adapted from Leontidis, 2002; Zhang and Cremer, 2006).
Figure 3 Representation of Hofmeister cations with increasing chaotropic potency from left to right (adapted from Hess and van der Vegt, 2009)
Many of these ions directly influence enzyme function and protein solubility. Specific effects depend on protein concentration and type, pH and water potential. According to the Hofmeister series, ions are ordered by their ability to increase protein solubility ("salting in" or chaotropic), with increasing chaotropic potency on the extreme right (see Figures 2 and 3).
Chloride is regarded as being the pivot point between kosmotropic ("salting out") anions on the left and the chaotropes on the right. The relative ranking of anions in this series is dictated by four variables: charge, size, hydration strength and polarizability (the latter is not independent of size). Kosmotropic ions tend to be small and to have low polarizability and electric fields at short distances and lose their hydrating water reluctantly (Leontidis, 2002). Chaotropic ions tend to be large and have weak electric fields and a loose hydration shell, which they part with easily. Hence, the size, charge and hydration strength of ions dictates whether they are chaotropic (increase protein solubility) or kosmotropic (reduce protein solubility). Kosmotropic ions compete for water aggressively, dehydrating the surface of competing moieties, especially if they are already inherently hyhobic, whereas chaotropic ions lose their hydrating water easily and contribute to the persistence of solubility at the interface.
The relevance of such ion-specific effects to avian nutrition and their contribution to variance in FCR is not clear and is the focus of research in our laboratory. However, it is theoretically possible to modify digestibility, especially that of hyhobic proteins such as kafirin in sorghum through modification of the Hofmeister 'pull' by altering the ion balance in the diet to favour the chaotropes. In this regard, it is noteworthey that phytate, which has six esterified phosphate moieties, is an 'uber-kosmotrope'. Ions of importance in this context include Na, Cl, K, Ca, P and also phosphates, sulphates and phytate.
Conclusions
Irrespective of the methods by which it is accomplished, improved feed efficiency of broilers will reduce the environmental impact of chicken-meat production and increase the economic viability of the industry. Consequently, both ecological and financial factors are driving the quest for enhanced feed efficiency and this is superimposed on the fact that the environmental impact of chicken-meat production is inherently less than some alternative meat production systems.
This paper was presented at the Recent Advances in Animal Nutrition (RAAN), July 2011. Engormix.com thanks for this huge contribution.