Approximately two billion tonnes of cereal grains and 140 million tonnes of legumes and oilseeds are produced annually throughout the world. This production is associated with an estimated 230 million tonnes of fibrous material as part of a variety of by-products. In addition, the ‘dietary fibre’ contained within cereals and legumes is used inefficiently by the intensive livestock industries.
At present, enzyme supplements are used extensively in wheatand barley-based diets for poultry, both broilers and layers. This is an area that is both well researched and well documented. Although this area will not be elaborated in this review, the efficacy and success of exogenous enzymes for cereal-based diets has been highlighted by Charlton (1996), who commented that 90 to 95% of all broiler diets in the UK and Ireland include enzymes to supplement these cereals in order to improve their feeding value and enhance litter conditions.
Pentosanase (arabinoxylanase) is generally added to wheatbased diets (and also to rye-based diets where applicable) to counter the antinutritive effects of pentosans (arabinoxylans) that cannot be hydrolysed by the endogenous enzymes, and which cause decreased feed conversion and ‘sticky droppings’. Another enzyme, ß-glucanase, is generally added to barleybased diets to hydrolyse the ß-glucans that cause similar problems in birds.
Interest in the use of enzymes in the pig industry, especially for the newlyweaned pig, is gaining momentum. In poultry it is clear that the antinutritive effects of soluble non-starch polysaccharides (NSP) are associated with the viscous nature of these polysaccharides; however, the viscosity of digesta does not appear to interfere with the process of digestion in the young pig to the same extent. The most probable explanations for improved performance of young pigs fed enzymes are effects on feed transit time, endogenous losses, NSP degradation and(or) changes in gut flora.
Whilst there has been widespread acceptance of cereal-hydrolysing enzymes in the poultry industry, and to some extent in diets for the weaned pig, relatively less attention has been paid to the remaining 25 to 35% of, for example, broiler feed, which is not derived from cereal grains, but which is supplied predominately as vegetable protein (Pugh and Charlton, 1995). Given this, there would appear to be an incentive to develop an enzyme preparation tailored to sources of vegetable protein, such as soybean meal, peas and beans, and oilseed/legume mixtures, to enhance their nutritive value for the pig and poultry industries. The purpose of this paper is to review research conducted with Vegpro, an enzyme complement developed by Alltech Inc., to improve the efficiency of digestion in sources of vegetable protein. It is first necessary, however, to examine the chemistry and structure of ‘dietary fibre’ to establish a basis for enzyme design.
CHEMISTRY AND STRUCTURE OF PLANT CELL WALLS
The commonly used vegetable protein feedstuffs, whilst relatively high in energy and protein, also contain anti-nutritional factors in the form of NSP, oligosaccharides and phytase, as well as proteins such as lectins, tannins and trypsin inhibitors (e.g. glycinin, ß-conglycinin in soybean meal). Much has been written about the chemistry, structure and physicochemical effects of cereal NSP in diets for pigs and poultry, in particular that of the broiler chicken (see Annison and Choct, 1994). The polysaccharide structures commonly found in feed ingredients of plant origin are depicted in Figure 1, and the concentration of NSP in cereals and legumes used commonly in the intensive livestock industries are listed in Table 1.
Table 1. Total NSP levels and major NSP in legumes (after Annison and Choct, 1993, and Choct, 1997).
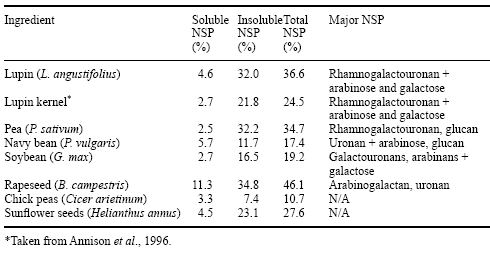
In simple terms, most dietary fibre polysaccharides are plant cell wall components intimately associated with other polysaccharides and(or) noncarbohydrates such as (glyco)proteins, phenolic esters and lignin (Selvendran, 1984; Annison and Choct, 1994; Bjergegaard et al., 1997). The main components that make up dietary fibre are listed in Table 2. The exact structure and resulting physicochemical properties of cell wall polysaccharides are determined primarily by (a) the pattern in which the polysaccharides and other components are arranged, and (b) the bonding between molecules of the cell wall components (Annison and Choct, 1993).
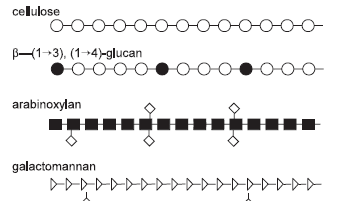
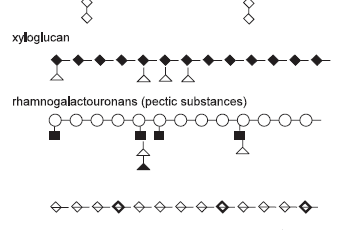
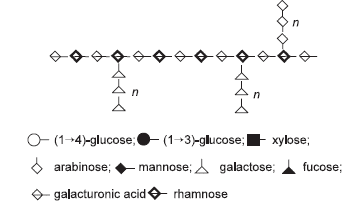
Figure 1. The polysaccharide structures commonly found in feed ingredients of plant origin (after Smits, 1997).
Table 2. Components of dietary fibre (after Selvendran, 1984).
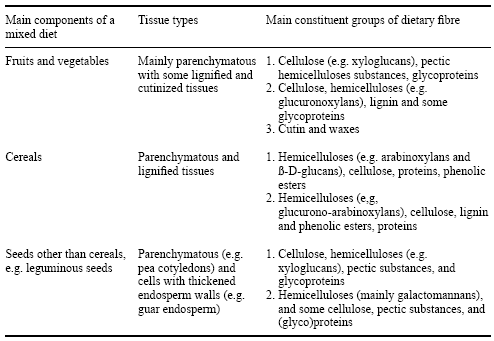
Plant cell walls comprise a complex group of high molecular weight compounds that are secreted by and surround the cells. Plant cell walls can be considered as plant cell structures composed of discrete layers, with the middle lamella in the centre of the cell wall between adjacent cells. The layer formed after the middle lamella is the primary cell wall and toward the cell cytosol is found the secondary cell wall consisting of a distinct sublayer. The formation of the secondary cell wall is a thickening process and a function of plant maturity (Bjergegaard et al., 1997).
In the development of the primary cell wall of dicotyledonous plants such as legumes, pectic substances (as calcium salts) are deposited on the cell plate to form the middle lamella that cements the cells together. Hemicelluloses, additional pectic substances and glycoproteins are then deposited as an amorphous matrix of macromolecules closely associated with the cellulose microfibrils that are the chief structural elements. The main cell wall polymers of parenchymatous tissues of dicotyledons are pectic substances, hemicelluloses (e.g. xyloglucans) and cellulose (Figure 2). In contrast, the cell walls of cereal grains (wheat, barley) contain very little pectic substances, with the primary cell walls having cellulose microfibrils closely associated with glucomannan. These fibrillar structures are embedded in an amorphous matrix of hemicelluloses that consist predominately of arabinoxylans and(or) ß-D-glucans, some of which are cross-linked by phenolic esters and(or) proteins (Selvendran, 1984).
The constituents of cell walls, therefore, fall into three groups: the fibrillar and matrix polysaccharides that are formed simultaneously, and the encrusting substances (lignins) that are formed during secondary thickening. The fibrillar polysaccharides, which are the basic structural units of microfibrils, comprise mainly cellulose. The matrix polysaccharides are made up of both linearly oriented polymers and highly branched polysaccharides. There are two major fractions of the matrix polysaccharides:
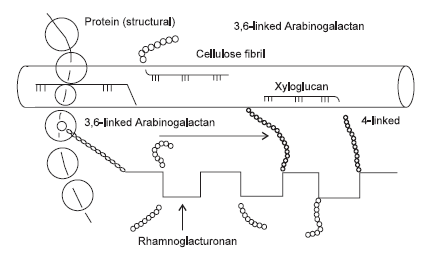
Figure 2. A general model of a primary plant cell wall (after Chesson et al., 1995).
1. the pectic substances, or those polysaccharides solubilized by chelating agents (e.g. EDTA), such as arabinans, galactans and arabinogalactans which lack the rhamnogalactouronan backbone, and
2. the hemicelluloses, or those polysaccharides solubilized by alkali.
Polysaccharides in legumes, therefore, are more complex in structure than the relatively simple polysaccharides found in cereals. They contain a mixture of colloidal polysaccharides called pectic substances (galactouronans, galactan and arabinans) (Figure 3). Neutral polysaccharides such as xyloglucans and galactomannans have also been reported, although the occurrence, structure and levels of these polysaccharides differ among legumes. This makes it difficult to target the legume polysaccharides for feed enzyme supplementation (Annison and Choct, 1993). For effective degradation of cell wall polysaccharides the combined action of different polysaccharidases is required, but prerequisite is a detailed understanding of the type and structure of the substrates and of the specificities of the enzyme activities used.
In addition, plant cell walls contain appreciable amounts of proteins as structural (glyco)proteins (arabinogalactan protein, hydroxyproline-rich glycoproteins, proline-rich proteins and glycine-rich proteins), lectins and endogenous enzymes, e.g. myrosinase in rapeseed (Bjergegaard et al., 1997). The total amount of protein in cell walls may account for 10 to 30% of the total dietary fibre mass. In most cases, especially in diets for monogastric animals, this protein will remain encapsulated within the polysaccharide matrix and therefore be unavailable to the animal. Release of this protein may lead to an increase in amino acid availability to the animal.
THE USE OF ENZYMES TO INCREASE THE NUTRITIVE VALUE OF VEGETABLE PROTEINS
There have been relatively few studies investigating the use of enzymes in diets containing legumes. One problem is that because poultry diets often contain considerable amounts of cereals, it is difficult to assess the efficacy of enzyme preparations targeting NSP in legumes because the enzymes frequently contain arabinoxylanase and ß-glucanase activity and will hence hydrolyse the cereal NSP. In one study, Castanon and Marquardt (1989) added enzyme preparations of microbial origin (protease, cellulase, polygalactouronase and a-amylase) to diets for broilers containing field beans (V. faba), and reported a significant increase in weight gain and decrease in feed:gain when cellulase, protease, and cellulase plus protease were added.
Interestingly, these authors reported an increase in the variability between replicates in the presence of enzymes, an effect that may have been caused by the very high level of field beans in the diet (90.6%). Autoclaving and fermenting also resulted in production gains; however, the use of enzymes under these treatments failed to provide any additional production benefits.
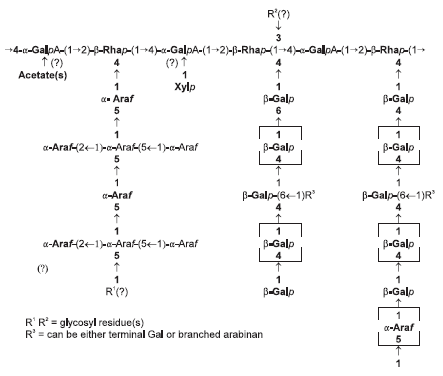
Figure 3.The structure of pectin from a leguminous plant (M. Choct, personal communication).
Brenes et al. (1993) reported a significant increase in the nutritive value for broilers of Canadian lupins by supplementation of the diet with an appropriate mixture of crude enzymes (ß-glucanases, xylanases, hemicellulases, pectinases, proteases, inulinase and a-galactosidases), autoclaving, and dehulling. Enzyme addition to lupin-containing diets appeared to exert its beneficial effect by increasing dry matter, protein and oligosaccharide digestibilities and nitrogencorrected apparent metabolizable energy (AMEn) values. Annison et al. (1996) also reported an increase in AMEn values when an enzyme preparation was added to a diet containing dehulled lupins, although they failed to observe differences in protein and starch digestion.
In 1993 a joint research project between Alltech Inc. and the British Agricultural Development and Advisory Service (ADAS) was established to examine the potential for enzyme supplementation of vegetable protein sources used in poultry diets. Particular emphasis was placed on soybean meal and modified rape/pea/bean products used in poultry diets. Enzyme complements were evaluated at the European Bioscience Centre in Ireland to ascertain the most suitable, and then tested at the Roslin Research Institute in Scotland in trials measuring nitrogen-corrected true metabolizable energy (TMEn) in broilers.
The enzyme complement, called Vegpro, was tested initially with three vegetable protein sources. The first two were the legume/oilseed rape compounds for which the enzyme was originally developed, and which generally comprise full-fat canola or rapeseed (‘00’) combined with peas or beans in a 50:50 ratio and heated to enhance digestibility. Soybean meal was included as the third protein source. In a subsequent trial, the complement was modified to more specifically address the NSP composition of soybean meal, with Vegpro being added to both extracted soybean meal and full-fat soybeans. A third trial examined effects of Vegpro on amino acid digestibility in 3-weekold broilers. Pugh and Charlton (1995) and Charlton (1996) provide a complete account of this work; however, the results of the first two trials are listed in Tables 3 and 4.
Table 3. The effect of Vegpro addition (1 kg/tonne) on the TMEn value of three sources of vegetable protein fed to broiler chickens (after Pugh and Charlton, 1995).
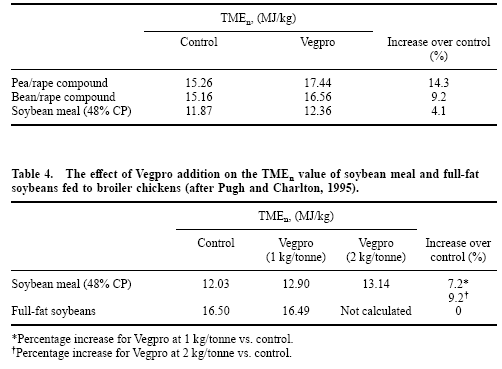
Use of Vegpro resulted in significant increases in the TMEn of all vegetable proteins tested, except for that of full-fat soybeans. These data have been supported by further research conducted with broiler chickens (see Charlton, 1996). In the second trial (Table 4), doubling the level of enzyme inclusion did not double the response, suggesting that a threshold level of digestion in vivo brought about by exogenous addition of Vegpro had been reached. Further, Pugh and Charlton (1995) commented that (a) addition of Vegpro reduced the variation in TMEn between replicates, and (b) there were no ill effects of the higher inclusion level of Vegpro on the birds.
In more recent trials, McNab and Bernard (1997) tested the efficacy of Vegpro by determining the TMEn and true amino acid digestibility in soybean meal. In their first experiment, these authors addedVegpro to 48% crude protein soybean meal at concentrations of 0, 1 or 2 kg/tonne and fed it to starved (48 hours) roosters (50 g/bird). From the excreta voided in the ensuing 48 hours, TMEn and true digestibility coefficients of the constituent amino acids were determined and were found to improve by around 9%. In a second study using Canadian soybean meal, Bernard and McNab (1997) determined TMEn and true amino acid digestibilities in both 3-week-old chicks and adult birds in the presence of Vegpro added at 2 kg/tonne. Addition of Vegpro increased TMEn by 7.4% in chicks but not adult cockerels. Similarly,Vegpro addition increased the true digestibility coefficient of most essential amino acids in diets fed to chicks whereas there was no response in adult birds (Table 5).Acomparison of these two studies suggests that there is considerable variation in the nutritive value of soybean meal, and that the expected positive response in energy and amino acid digestibility of soybean meal to Vegpro may therefore not always occur. The difference in response based on age of the bird raises questions about enzyme insufficiency at certain ages, transit time of digesta, and(or) gut conditions (e.g. pH or bile salt levels) related to optimal enzyme digestion.
The recent work of Schang et al. (1997) highlights the importance of diet density and diet type on the response toVegpro. Schang et al. (1997) conducted a 2 x 2 x 2 factorial experiment with the respective factors being nutrient density [100% (High) or 90% (Low) of energy and available amino acid requirements], diet type (corn/soy or corn/soy/full-fat soy/wheat bran), and enzyme addition (Vegpro) (Figure 4). Each treatment consisted of five replicates with 10 birds per replicate.
In this trial, 1-day-old Arbor Acres broiler males were placed in battery pens and fed with starter (1 to 21 days), finisher (22 to 42 days), and market (43 to 53 days) diets. The enzyme Vegpro was added at 300 and 200 g/tonne for the starter and finisher/market periods, respectively. Birds fed low nutrient density diets had a lower body weight gain (2.59 vs. 2.86 kg, P < 0.05) and a higher feed conversion ratio (2.24 vs. 2.04, P < 0.05) than birds fed the high nutrient density diet. The addition of enzyme to birds fed the high nutrient density diet had no effect at slaughter (P > 0.05), suggesting that the benefits of using enzymes may be limited when added to diets formulated to exceed animal nutrient requirements.
Schang et al. (1997) reported that the response to Vegpro depended on nutrient density and also varied according to diet type, such that the addition of Vegpro to low density diets containing corn/soy/full-fat soy/wheat bran resulted in significant improvements in body weight gain (4.7%) and feed conversion (1.5%). No effect was observed whenVegpro was added to the low density diet containing only corn/soy. Interestingly, birds fed diets containing Vegpro had a larger abdominal pad indicative of increased energy availability.
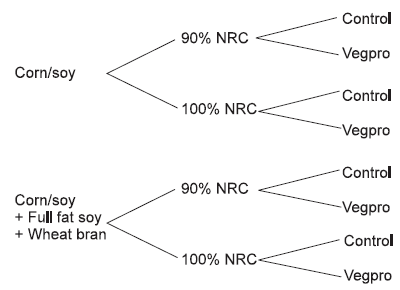
Figure 4.Diagrammatical representation of the experimental design used by Schang et al., 1997.
Table 5. Effect of Vegpro on TMEn and true amino acid digestibilities of soy for 3-week-old chicks and adult cockerels (after Bernard and McNab, 1997).
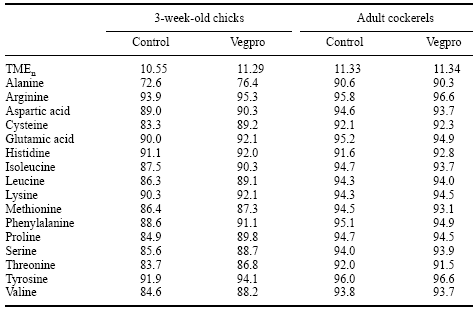
But how may energy and amino acid utilization be enhanced simultaneously? Walsh et al. (1997) conducted a study in vitro using cellulase and protease preparations (Alltech Inc), and in which the amount of glucose and the amount of a-amino nitrogen released from samples of whole rapeseed were examined using each enzyme singly or in combination. Treatment of whole rapeseed with cellulase caused a release of reducing sugars (measured as glucose), whereas the use of protease did not promote a significant release of glucose. However, a synergistic positive effect on glucose release was observed when cellulase and protease were added to the substrate together (Table 6).
Table 6. Treatment of whole rapeseed with cellulase, protease, and cellulase + protease, and its effect on the release of reducing sugars (expressed as glucose equivalent) and amino acids/peptides (expressed as a-amino N) (after Walsh et al. 1997). Values are mean (SEM).
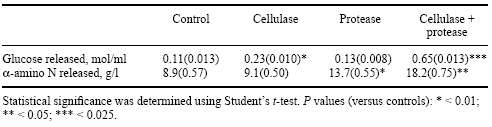
The hull of rapeseed contains 12 to 16% protein and 44% crude fibre, the bulk (73%) of which comprises cellulose (Bell, 1984). The various structural components present in the seed coat are intimately associated; and partial degradation of one component would most likely expose the additional structural components to enzymatic attack. This synergism is also reflected in the enzyme-mediated protein degradation seen. While treatment with protease resulted in significant release of a-amino nitrogen from whole rapeseed, the presence of cellulase augmented this effect (Table 6). These data provide evidence that protein is ‘bound up’ amongst structural polysaccharides in the seed coat, and that its release (and eventual utilization by the animal) is dependent upon the action of carbohydrate-hydrolysing enzymes such as cellulase to ‘disperse’ the protein-NSP matrix.
In an associated experiment, Walsh et al. (1997) obtained a preparation of crushed rapeseed/pea meal mix and subjected it to a cellulase/protease cocktail to examine its effects on particle size. Enzymatic treatment of this feedstuff mix caused a more homogeneous mix with reduced particle size (Table 7), and a visual reduction in the whole rapeseed content of the feed mix. Reduction in particle size mediated by enzymatic action on the fibrous components of a feedstuff is likely to increase digestibility by providing more accessible nutrients that were previously protected within the cell wall matrix.
Table 7. Effect of a cellulase + protease enzyme combination on particle size distribution of a commercial rapeseed/pea meal mix (after Walsh et al., 1997).
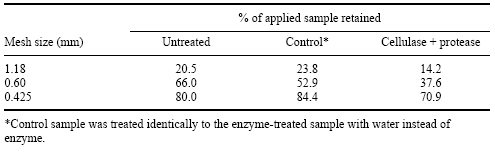
APPLICATION OF VEGPRO IN PIGS
The efficacy of Vegpro for pigs has been determined in only a limited number of trials. In the most comprehensive trials to date, Lindemann et al. (1997a,b) used 120 pigs (60 barrows and 60 gilts; mean initial and final bodyweights of 26 and 109 kg, respectively) to assess the effects of enzyme on pig performance. Dietary treatments were structured as a 2 × 2 factorial of energy level (High vs. Low: corn/soybean meal ± 20% wheat middlings) and enzyme addition (Vegpro at 1 kg/tonne, or 0.1%). Diets were formulated to meet or exceed NRC (1988) estimates while ensuring that lysine was the first limiting amino acid with a constant lysine:energy ratio. The difference in energy level between the high and low energy diets was 6.3% (14.56 vs. 13.64 MJ DE/kg for growers, and 14.86 vs. 13.92 MJ DE/kg for finishers). Pigs had ad libitum access to feed and water.
The addition of wheat middlings decreased average daily gain (ADG) (828 vs. 758 g/day, P < 0.01), increased daily feed intake (2.36 vs. 2.61 kg/day, P < 0.05) and increased feed:gain (2.85 vs. 3.45, P < 0.01). Enzyme inclusion decreased feed:gain in the growing period (2.74 vs. 2.54, P < 0.10). However, single degree-of-freedom comparisons for the effects of the enzyme indicated an increased (P < 0.05) ADG in the growing and total periods (771 vs. 837 g/day and 799 vs. 855 g/day, respectively) and a decreased feed:gain in the growing period (2.57 vs. 2.29, P < 0.10) for pigs fed the high-energy diet (Table 8).
Wheat middlings consist of fine particles of wheat bran, wheat shorts, wheat germ, wheat flour and some mill tailings. As such, there is generally a degree of variability in the quality of wheat middlings. Lindemann et al. (1997a,b) included wheat middlings in their trials to dilute the energy content of the diet. However, and because middlings are wheat by-products, it is little surprise that Vegpro had no influence in the diet containing middlings because they served to dilute the quantity of substrate available to the enzyme, thereby reducing the response. It is also noteworthy that the response was diminished in the finishing period (compared to the growing period), a result again that can be partially explained at least by a substrate reduction phenomenon.
Similar improvements using a corn/soy diet were reported from a trial conducted in Brazil (Central Cooperative of Santa Catarina). In this trial, pigs weighed an average of 26 kg and were fed a corn/soybean diet with or without Vegpro (1 kg/tonne) for 88 days. After this time, pigs fed the diet containing Vegpro were 6 kg heavier due to an 8% increase in average daily gain (788 vs. 730 g/day). These pigs also converted feed more efficiently (2.83 vs. 2.98, an improvement of 5%) despite consuming similar amounts of feed (2.23 vs. 2.18 kg/day for pigs fed diets with and without Vegpro).
Table 8. Effect of addition of Vegpro to diets on the performance of growing/finishing pigs (after Lindemann et al., 1997b).
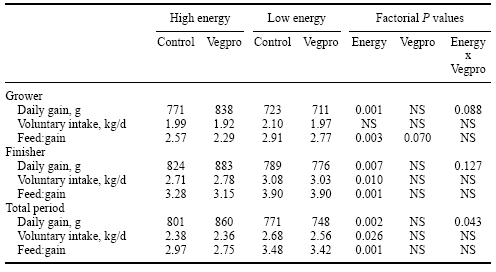
Using younger pigs, Lindemann et al. (1997a) weaned pigs at 21.4 days of age and 6.8 kg onto complex diets having 0, 0.075 or 0.15% Vegpro. For the 3 weeks following weaning, there were no effects (P > 0.10) observed for daily gain (362, 372, 379 g/day) and daily feed intake (521, 521, 529 g/day); however, a linear improvement (P < 0.07) was observed for feed:gain (1.45, 1.40, 1.39 respectively).
In a series of farm trials conducted in the UK and reported by Kitchen (1997), the efficacy of Vegpro was tested in growing pigs. A control diet was manufactured from cooked and uncooked cereals, cereal grain by-products, oilseed products and by-products including extracted soybean meal, fish meal, whole heat-treated rapeseed, milk products, vegetable oils and vitamins and minerals. The test diet included Vegpro added at 500 g/tonne. Five groups of pigs were fed the control diet and four groups were fed the test diet.
Pigs fed the diet containing Vegpro ate more feed but also converted it more efficiently, resulting in a 62 g/day (P > 0.05) greater growth rate compared to pigs fed the control diet (Table 9). However, when expressed on the basis of pig liveweight produced/tonne of feed, pigs fed Vegpro produced an extra 27.9 kg per tonne of feed – equivalent to £33.46 extra income at a liveweight price of £1.20/kg (Kitchen, 1997).
Table 9. Performance of pigs fed diets with and without the addition of Vegpro included at 500 g/tonne (after Kitchen, 1997). Values are means (S.D).
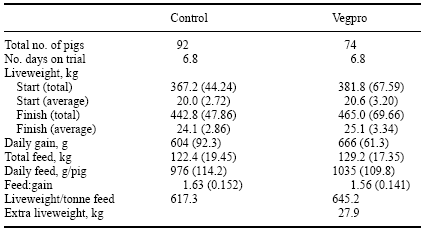
THE IMPORTANCE OF OLIGOSACCHARIDES IN VEGETABLE PROTEINS
Oligosaccharides (a-galactosides) are found in relatively high concentrations in some vegetable proteins. In lupin seeds for example, high levels (70 to 120 g/kg; Trugo et al., 1988; Evans et al., 1993) of oligosaccharides of the raffinose, stachyose and verbascose series are found that are not digested by endogenous enzymes present in the small intestine. Pigs and poultry lack the enzyme (a-galactosidase) needed to hydrolyse oligosaccharides to galactose and sucrose; hence there has been interest in the use of microbially derived a-galactosidase in diets as a means of increasing the nutritive value of lupins. Furthermore, NSP and a-galactosides in combination can constitute up to 48% of lupin seed dry matter (Gdala and Buraczewska, 1996), representing a considerable wastage of nutrients given that NSP and oligosaccharides will not be digested completely in the small intestine.
In poultry, Carré et al. (1995) reported very low digestibilities of NSP in both broiler chickens and adult cockerels when both soybean meal (325 g/kg) and peas (475 g/kg) were included in diets. These authors reported total tract digestibilities of NSPof around 6% or less (range 2.8 to 6.1%). Total tract digestibility of the a-galacto-oligosaccharides was much higher, with a mean value of 0.867 recorded for broilers and 0.99 for adult birds. These data suggest that there is considerable microbial degradation of these compounds in the digestive tract of birds, although no measurements were made of the extent of hydrolysis of a-galacto-oligosaccharides prior to their entry into the caeca. This is likely to be important given the possibility of digestive upsets caused by the passage of a-galacto-oligosaccharides into the caeca, as well as the energetic implications of digestion in the small intestine and absorption as glucose versus production of volatile fatty acids and their subsequent absorption (Müller et al., 1989). Here, in addition to reducing excreta organic matter and moisture content, the use of an exogenous a-galactosidase may be appropriate.
In pigs, Gdala et al. (1997) added an a-galactosidase preparation to diets containing several cultivars of lupins that were fed to young pigs (11 to 24 kg) fitted with post-valvular T-cecum (PVTC) cannulae in order to determine ileal oligosaccharide and apparent amino acid digestibility. These authors reported that the addition of microbial a-galactosidase had a significant positive effect both on the ileal digestibility of oligosaccharides (commensurate with increased ileal gross energy digestibility) and most amino acids (Table 10). These data support the work of van Barneveld et al. (1996, 1997) who removed a proportion of the oligosaccharides in lupins by ethanol extraction and then fed the oligosaccharide-free lupins to pigs. Van Barneveld et al. (1996, 1997) reported improvements in ileal and faecal digestible energy in the vicinity of 0.5 MJ DE when oligosaccharides were removed from lupins; and also reported improvements in ileal amino acid digestibility.
These data in pigs appear in contrast to those observed in poultry. Numerous workers using canola meal or soybean meal with both broilers and adult birds (cockerels and laying hens) have reported no beneficial effect of removing the oligosaccharides or adding an a-galactosidase preparation to diets containing these legumes. In fact, all these authors reported decreases in the energetic values of these feedstuffs under these conditions (Slominski et al., 1994; Irish et al., 1995; Hughes, personal communication). In addition, Hughes (personal communication) reported an increase in digesta viscosity when oligosaccharide-free dehulled lupins were fed to broiler chickens.
ENERGY VALUE OF VEGETABLE PROTEIN SOURCES
At the Monogastric Research Centre at Massey University in New Zealand, we have recently investigated the apparent digestible energy content and whole-tract amino acid digestibility of a number of vegetable protein sources, namely soybean meal, peas, beans, lupins and canola (rapeseed) meal, in the presence of Vegpro. In this study (Pluske et al., unpublished data), growing pigs (35 to 45 kg) were housed individually and fed a source of vegetable protein, which was included in a basal diet consisting of corn starch and sugar. Inclusion rates varied from 17.5% for tick (field) beans to 40% for peas. The inert marker chromium oxide was added to determine digestibility. Following a 7 day acclimatization period, a ‘grab sample’ of faeces was collected daily for 5 days, bulked, and will be subsequently analysed for gross energy, Chromium, nitrogen and amino acids. Completion of the laboratory analysis for this study will contribute additional information on the value of Vegpro for growing pigs.
Table 10. Ileal digestibility (%) of dry matter, gross energy, oligosaccharides and selected amino acids in piglets fed on lupin-based diets without or with a-galactosidase supplementation (after Gdala et al., 1997).
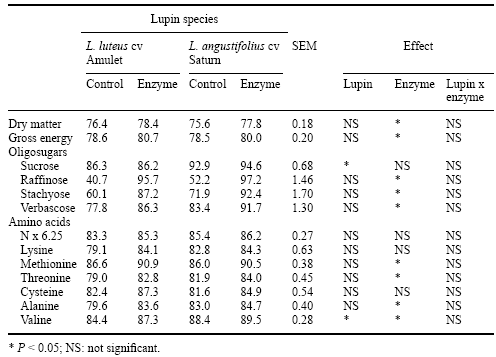
CONCLUSIONS
The research outlined in this paper illustrates that advantages in productivity and profitability for the pig and poultry industries are tenable with the use of the enzyme Vegpro. These data should be viewed against the ‘current state of play’ in many parts of the world, especially Europe, with respect to the ban on antibiotic growth promoters and likely changes in the use of animal protein supplements. Increasing the nutritive value of feedstuffs such as soybean meal, rapeseed, rapeseed meal, beans and peas by the use of Vegpro offers potential to reduce diet cost commensurate with enhanced production. Moreover, increasing the digestibility of amino acids has the potential to reduce total dietary nitrogen concentration which, coupled with the improved digestibility (efficiency), will reduce the amount of nitrogen entering the environment. This will assist in alleviating the concerns of legislators and regulatory bodies.
Finally, the practical use of Vegpro in diets has been outlined by Charlton (1996), and he suggests three ways to use the supplement:- Simple addition to the diet: Vegpro added on top of the standard diet will enhance bird (and pig?) performance, although diets formulated in excess of requirements will show little benefit to Vegpro inclusion;
- As another raw material in the diet: Using the ‘average’ improvements observed in energy and amino acid digestibility, and the enzyme cost, a new ingredient (such as ‘48% soybean + enzyme’) can be added to the matrix and used in least-cost diet formulation; and
- Relax cost constraints in formulation: the changes in energy and amino acid digestibility with Vegpro reduce overall diet costs.
REFERENCES
Annison, G. and M. Choct. 1993. Enzymes in poultry diets. In: Enzymes in Animal Nutrition – Proceedings of the 1st Symposium (eds. C.Wenk and M. Boessinger), p. 61. Schriftenreihe aus dem Institut für Nutztierwissenschaften, ETH Zürich.
Annison, G. and M. Choct. 1994. Plant polysaccharides – their physiochemical properties and nutritional roles in monogastric animals. In: Biotechnology in the Feed Industry: Proceedings of Alltech’s 10th Annual Symposium (eds. T.P. Lyons and K.A. Jacques), p. 51. Nottingham University Press, Loughborough, Leics, UK.
Annison, G., R.J. Hughes and M. Choct. 1996. Effects of enzyme supplementation on the nutritive value of dehulled lupins. Br. Poult. Sci. 37:157.
Bell, J.M. 1984. Nutrients and toxicants in rapeseed meal: a review. J. Anim. Sci. 58:996.
Bernard, K. and J.M. McNab. 1997. Effect of Vegpro on true amino acid digestibilities of Canadian 48% crude protein soybean meal fed to either three week old chicks or adult cockerels. In: Biotechnology in the Feed Industry – Proceedings of Alltech’s 13th Annual Symposium. Poster presentation.
Bjergegaard, C., H. Sørensen and S. Sørensen. 1997. Dietary fibres – important parts of high quality food and feeds. J. Anim. Feed Sci. 6:145.
Brenes, A., R.R. Marquardt, W. Guenter and B.A. Rotter. 1993. Effect of enzyme supplementation on the nutritive value of raw, autoclaved and dehulled lupin (Lupinus albus) in chicken diets. Poult. Sci. 72:1731.
Carré, B., J. Gomez and A.M. Chagneau. 1995. Contribution of oligosaccharide and polysaccharide digestion, and excreta losses of lactic acid and short chain fatty acids, to dietary metabolisable energy values in broiler chickens and adult cockerels. Br. Poult. Sci. 36:611.
Castanon, J.I.R. and R.R. Marquardt. 1989. Effect of enzyme addition, autoclave treatment and fermenting on the nutritive value of field beans (Vicia faba L.). Anim. Feed Sci. Technol. 26:71.
Charlton, P. 1996. Expanding enzyme applications: higher amino acid and energy values for vegetable proteins. In: Biotechnology in the Feed Industry– Proceedings of Alltech’s 12th Annual Symposium (eds. T.P. Lyons and K.A. Jacques), p. 317. Nottingham University Press, Loughborough, Leics, UK.
Chesson, A., C.W. Forsberg and E. Grenet. 1995. Improving the digestion of plant cell walls and fibrous feeds. In: Recent Developments in the Nutrition of Herbivores. Proceedings of the IVth International Symposium on the Nutrition of Herbivores (eds. M. Journet, E. Grenet. M.-H. Farce, M. Thériez and C. Demarquilly), p. 249. INRA Editions, Paris.
Choct, M. 1997. Non-starch polysaccharides: chemical structure and nutritional significance. Feed Mill. Int., June: 13.
Evans, A.J., P.C-K. Cheung and N.W.H. Cheetham. 1993. The carbohydrate composition of cotyledons and hulls of cultivars of Lupinus angustifolius from Western Australia. J. Sci. Food Agric. 61:189.
Gdala, J. and L. Buraczewska. 1996. Chemical composition and carbohydrate content of seeds from several lupin species. J. Anim. Feed Sci. 5:403.
Gdala, J., A.J.M. Jansman, L. Buraczewska, J. Huisman and P. van Leeuwin. 1997. The influence of a-galactosidase supplementation on the ileal digestibility of lupin seed carbohydrates and dietary protein in young pigs. Anim. Feed Sci. Technol. 67:115.
Irish, G.G., G.W. Barbour, H.L. Classen, R.T. Tyler and M.R. Bedford. 1995. Removal of the alpha-galactosides of sucrose from soybean meal using either ethanol extraction or exogenous alpha galactosidase and broiler performance. Poult. Sci. 74:1484.
Kitchen, D.I. 1997. Enzyme applications in corn/soya diets fed to pigs. In: Biotechnology in the Feed Industry – Proceedings of Alltech’s 12th Annual Symposium (eds. T.P. Lyons and K.A. Jacques), p. 101. Nottingham University Press, Loughborough, Leics, UK.
Lindemann, M.D., J.L. Gentry, H.J. Monegue and G.L. Cromwell. 1997a. Determination of the contribution of an enzyme combination to the growth performance of pigs. J. Anim. Sci. 75 (Suppl. 1): 184 (Abstr.).
Lindemann, M.D., J.L. Gentry, H.J. Monegue, G.L. Cromwell and K.A. Jacques. 1997b. Determination of the contribution of an enzyme combination (Vegpro) to performance in grower-finisher pigs. In: Manipulating Pig Production VI (ed. P.D. Cranwell), p. 247. Australasian Pig Science Association, Werribee, Victoria, Australia.
McNab, J.M. and K. Bernard. 1997. The effect of proteases (Vegpro) on the true metabolisable energy (TMEn) and true digestibility of amino acids in soybean meal. Poult. Sci. 76 (Suppl. 1): 133 (Abstr.).
Müller, H.L., M. Kirchgessner and F.X. Roth. 1989. Energy utilisation of intracaecally infused carbohydrates and casein in sows. In: Energy Metabolism of Farm Animals (eds. Y. van der Honing andW.H. Close), p. 123. Wageningen Pers, Pudoc, The Netherlands.
National Research Council. 1988. Nutrient Requirements of Pigs. 9th Revised Edition. National Academy Press, Washington DC, USA.
Pugh, R. and P. Charlton. 1995. Enzyme applications for plant proteins: time to look beyond cereals. In: Biotechnology in the Feed Industry – Proceedings of Alltech’s 11th Annual Symposium (eds. T.P. Lyons and K.A. Jacques), p. 393. Nottingham University Press, Loughborough, Leics, UK.
Schang, M.J., J.O. Azcona and J.E. Arias. 1997. Effects of a soya enzyme supplement on performance of broilers fed corn/soy or corn/soy/full-fat soy diets. Poult. Sci. 76 (Suppl. 1): 132 (Abstr.).
Selvendran, R.R. 1984. The plant cell wall as a source of dietary fibre: chemistry and structure. Am. J. Clin. Nutr. 39:320.
Slominski, B.A., L.D. Campbell andW. Guenter. 1994. Oligosaccharides in canola meal and their effect on nonstarch polysaccharide digestibility and true metabolizable energy in poultry. Poult. Sci. 73:156.
Smits, C.H.M. 1997. Viscosity of dietary fibre in relation to lipid digestibility in broiler chickens. PhD Thesis,Wageningen Agricultural University. The Netherlands.
Trugo, L.C., D. Almeida and R. Gross. 1988. Oligosaccharide content in the seeds of cultivated lupins. J. Sci. Food Agric. 45:21.
Van Barneveld, R.J., L.E. Olsen and M. Choct. 1996. Effect of lupin oligosaccharides on energy digestion in growing pigs. Proc. Nutr. Soc. Aust. 20: 114.
Van Barneveld, R.J., L.E. Olsen and M. Choct. 1997. Lupin oligosaccharides depress the apparent ileal digestion of amino acids by growing pigs. In: Manipulating Pig Production VI (ed. P.D. Cranwell), p. 230. Australasian Pig Science Association, Werribee, Victoria, Australia.
Walsh, G.A., D.R. Headon and R.F. Power. 1997. Enzymatic treatment of rapeseed: synergistic effects of cellulase and protease. In: Biotechnology in the Feed Industry – Proceedings of Alltech’s 13th Annual Symposium. Supplement 1, Enclosure Code Enz 1.1.
Authors: J.R. PLUSKE1 and M.D. LINDEMANN2
1 Monogastric Research Centre, Massey University, Palmerston North, New Zealand
2 Department of Animal Sciences, University of Kentucky, Lexington, Kentucky, USA