In temperate regions of the world forage legumes growing in spring and summer are frequently stored as silage for delayed use during periods of feed shortage. However, in contrast with most grass species, they often undergo fermentation pathways that are detrimental from a nutritional perspective.
In the ensilage process there is an early and short aerobic phase followed by a major anaerobic phase during storage time, and finally a new exposure to air when feeding the ensiled forage. A wide range of epiphytic organisms are brought into silage fermentation at harvest time with enterobacteria, lactobacilli and clostridia the main groups involved in the anaerobic phase. Yeasts and molds are also present in quantities that are related to the amount of oxygen available (Woolford, 1984).
Under ideal conditions plant sugars should ferment to lactic acid in the shortest possible time and achieve a pH low enough to preserve forage from further fermentation.When an early acidic condition is established, plant nutrients are largely preserved and animals show good responses to inclusion of silage in the diet.
However, there are a number of interactions among plant composition, microbial populations and environmental factors that make it very difficult in practice to achieve such ideal conditions. Probably the most significant factors involved are the availability of plant sugars, the plant buffering capacity, the amount of oxygen permitted in the system and the initial numbers of different epiphytic organisms. These factors vary with plant species, plant morphology, agronomic traits, weather and ensiling management, resulting in significant variation in silage quality.
In this respect, there has been a special interest in legume silage research, not only because such silages can provide high levels of protein of plant origin, but also because they are the most difficult to ensile. This difficulty is due to low sugar content, high buffering capacity, high protein and high moisture contents.
The practical result of undesirable fermentation patterns is a reduced performance in animals fed such silage (McDonald et al., 1991). One would assume that proper laboratory analyses would identify any nutrient deficiency or imbalance so that appropriate supplementary feeds could be provided.
However, routine crude analyses have been shown to poorly reflect animal performance (Jones et al., 1996). In particular, the ammonia level in silage has been extensively used to describe fermentation quality and nutritional value of silages (Wilkinson, 1990). However, other than in very poorly preserved silages this index is unsatisfactory for explaining animal performance/silage quality relationships.
There are other low molecular weight nitrogenous compounds which are also precursors of an early rumen ammonia peak (Pichard and Rybertt, 1993) but they are ignored when relying solely on ammonia levels as an index of plant protein breakdown during ensiling.
Silage acidity is also frequently regarded as an important quality index; however, modern silage making has evolved to rather restricted fermentation systems by means of wilting and use of certain additives that promote a faster endpoint. Etheridge et al. (1993) and Choung and Chamberlain (1993) have reviewed the problems of silage acids with regard to voluntary intake.
Silage in vitro digestibility is another important analysis performed on silage samples. It is used either as such or as a basis for further estimation of metabolizable energy (ME), but those values can be very misleading when the weight and energy contribution of volatile compounds are ignored.
In fact, well-fermented silages show a significant reduction in plant cell wall digestibility with respect to the original forage (Jaurena, 1996), but direct calorimetry on original and ensiled forages show minor or even positive changes in energy concentrations after ensiling (McDonald et al., 1991). In this respect, estimations of ME on the basis of plant cell wall disappearance (Goering and Van Soest, 1970) fail to recognize the higher energy value of silages derived from alcohols and some acids.
In silage feeding, kinetics of rumen fermentation may be critical due to the asynchrony between massive release of lowmolecularweight nitrogenous compounds and the supply of readily available energy sources for rapid microbial mass growth (Pichard and Rybertt, 1993).
When planning silage feeding strategies, digestion values obtained after 48 hrs incubation in rumen fluid coupled with knowledge of crude protein levels provide only limited nutritional meaning. The amount of rumen fermentable energy in silages is low in comparison to fresh forages, thus reducing the potential value of metabolizable protein estimates (AFRC, 1992). In turn, the supply of nitrogen from feed to the rumen is often well beyond the animal’s ability to utilize it prior to salivary urea recycling. Also, the severe protein breakdown in poorly preserved silages further reduces the supply of metabolizable protein to the host animal and may also be limiting for rapid microbial growth.
It is well known that ultimately the best assessment of silage quality is obtained by animal performance experiments, but the need for rapid laboratory results is also recognised. The challenge is to provide meaningful analyses to nutritionists who assemble feeding strategies for diverse animal production situations.
Objectives of the current work
In this work we collected a number of alfalfa silages being used by dairy farmers in order to subject them to a comprehensive analytical assessment and discussion of the nutritional implications. Additionally, alfalfa silages made from a known fresh forage were prepared in order to obtain four types of fermentation patterns and investigate the meaning of their differing analytical profiles.
MATERIALS AND METHODS
Survey of farm silages
Eleven silages were sampled from an equal number of farms. All silages had been made in the spring-summer period from essentially pure alfalfa stands (Medicago sativa L.).
In each silo the external face was discarded and several representative subsamples were pooled to make one sample of approximately 10 kg. These samples were sealed in air tight bags and stored in boxes with dry ice until arrival in the laboratory where they were divided for fresh analyses, freeze-drying, oven drying and juice extraction.
Analyses of fresh material were conducted immediately and the others stored at –20 °C.
Experimental silages
Alfalfa was ensiled in experimental silos of 1 m
3 volume following four different strategies in order to obtain fermentation types the closest possible to homolactic, heterolactic, acetic and butyric patterns. The first was wilted and inoculated with Lactobacillus plantarum, well compacted and rapidly sealed.
Heterolactic fermentation was obtained with similar but less strict techniques and inoculation with a mixture of lactobacilli, clostridia and coliforms. The acetic pattern was obtained with direct cut silage and coliform inoculum. The butyric type was obtained by slow filling and application of an inoculum cultured from soil spores obtained near animal facilities. All silages were stored for 90 days and then opened for sampling and analysis.
Laboratory analyses
In fresh forage pH and buffering capacity were determined as in Playne and McDonald (1966) and the titratable acidity determined as milliequivalents of sodium hydroxide (NaOH) per kg of dry matter required to raise silage pH to 7.
Total nitrogen was determined by Kjeldhal (AOAC, 1984). Insoluble nitrogen was determined in the filter residue after a mineral buffer extraction and the soluble nitrogen calculated as the difference from total nitrogen (Pichard, 1977). Ammonia nitrogen was determined by the catalyzed indophenol reaction (Chaney and Marbach, 1962) in proteinfree extracts as described by Pichard (1977). The unavailable nitrogen was measured as the residual nitrogen insoluble in acid detergent (ADIN) as per Goering and Van Soest (1970).
Extraction of protein for determining true protein and running electrophoresis utilized samples of 100 mg freeze-dried and finely ground silages or 10 ml of pressed juice mixed with 50 ml buffer (borax, 1 mM; sodium phosphate, 26 mM; citric acid, 10 mM, sodium taurocholate, 0.0091%; b-mercaptoethanol, pH 9.0). The samples were heated to 100 °C in a water bath and thoroughly mixed until complete cleared of solids.
Subsamples of this extract were centrifuged at 1000 rpm for one minute and supernatants analysed for protein and subjected to electrophoresis.
Protein nitrogen was determined in the extract by the modified Lowry method developed by Tan et al. (1984) in order to avoid interference from phenolic compounds. The molecular weights of remaining proteins were determined in silage extracts prepared by the same procedure but substituting the sodium taurocholate by sodium dodecyl sulfate. Gel electrophoresis (PAGE-SDS 12.5%) was performed according to the method of Laemmli (1970) in a sodium chloride-enriched buffer as modified by Lobos and Mora (1991).
Amino acids were determined by high pressure liquid chromatography (HPLC) following acid hydrolysis and derivatization using a reverse phase column C18 3.9 x 300 mm at 46 °C in a dilution gradient for 67 minutes (Cohen, 1990). The amines putrescine, cadaverine, histamine and g-aminobutyric acid were determined as their benzoic derivative benzilamides by HPLC as described by Krizek (1993).
Plant cell wall fractions were determined by the detergent system (Van Soest et al., 1991). Reducing sugars were determined in filtrates of water extracts boiled with sulfuric acid by the Somogy reaction as described by McDonald and Henderson (1964). Starch was determined as free glucose (glucose oxidase Sigma Kit 315) in the residue washed with 85% ethanol and sequentially treated with a-amylase and amyloglucosidase based on the methods of McRae (1994) and Karkalas (1985).
Organic acids (citric, malic, succinic) were determined by HPLC (Schneider et al., 1987) and volatile fatty acid (VFA) and lactic acid in silage extracts by gas chromatography in a Carbopack BDA column 4% Carbowax 20M (Supelco 1-1889) using a Fisons GC8000 instrument (Fussell, 1987).
In vitro rumen digestions were determined after 24 and 48 hrs incubation by the method of Goering and Van Soest (1970) in freeze-dried coarsely ground samples. The rumen inoculum donor was a mature cow fitted with a rumen cannula and fed a mixed alfalfa silage wheat straw diet (50:50).
Results and discussion
CHEMICAL CHARACTERIZATION OF FARM AND EXPERIMENTAL SILAGES
All farm silages had been wilted to approximately 30%, including two which were heavily wilted to 37 and 46 % dry matter (DM). The fermentation parameters differed widely among samples, revealing the diversity and complexity of the microbe-plant-silage management interactions (Table 1). The pH ranged from 4.2 to 5.9, being related to moisture content and quality of fermentation.
Similarly, titratable acidity varied widely from 166 meq/100g in a badly conserved silage to 812 meq/100g in a wellpreserved forage. The fatty acid profiles in farm silages yielded an average 8 and 3% lactic and acetic acids, respectively, with acetic acid less variable among samples than lactic acid. There were two very high butyric samples (I9 and J10) plus four other samples that contained more than 5% of total acids as butyric. Ethanol was found in all samples at an average concentration of 0.4%, and deviations had no biological significance.
The experimental silages were less wilted and their composition confirms that four different fermentation patterns were successfully obtained. All but the butyric sample had the same pH and similar titratable acidity.
The lactic-type silage had the largest quantity of total acids and a wide lactic/acetic ratio. The heterolactic and acetic silages had very similar fermentation patterns, although the former had a greater quantity of total acids. The composition of the butyric silage represented a typically poor fermentation dominated by clostridia with high pH, low titratable acidity and lactic acid representing only 20% of total fatty acids.
FATES OF NITROGENOUS COMPOUNDS
The total nitrogen content of silages ranged from 2.49 to 3.65% due mainly to different plant morphological stages at harvest time (Table 2). The buffer solubility of nitrogenous fractions showed significant variation among samples and values were not in agreement with the expected ranking based on the fermentation pattern of each sample.
In samples that had the best fermentation, such as A1 and M13, we observed high percentages of soluble nitrogen. Conversely, both in farm and experimental samples, a relatively lower solubility was observed in low-lactic high-butyric silages. The fact that all farm silages had been wilted suggested that during fermentation there was a temperature-induced condensation and polymerisation of amino-aldehyde bases, thus reducing the solubility of nitrogenous compounds.
Even though ADIN values were not very high (with the exception of sample J10), values were well above the normal ranges for fresh alfalfa. The average buffer solubility in the farm silage samples was close to 60% and ranged from 46 to 69%.
The soluble nitrogen fraction has frequently been considered to be mainly non-protein nitrogen (NPN).
However, even though most NPN must be in the soluble fraction, our results show that in good silages there is a significant amount of soluble nitrogen that corresponds to true protein and peptides, which is consistent with the findings of Rooke and Nsereko (1993) in grass silages.
In Figure 1 the points above the line show the fraction of nitrogen not accounted for by the NPN. This corresponds to true protein contained in the soluble nitrogen fraction, in spite of the fact that the NPN fraction would be inflated by ADIN. In other terms, not all the true protein can be found in the insoluble nitrogen fraction.
Table 1. Dry matter content and fermentation profile of farm and experimental alfalfa silages.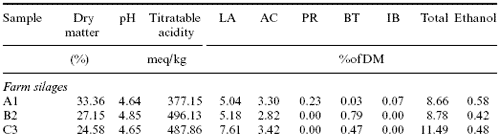
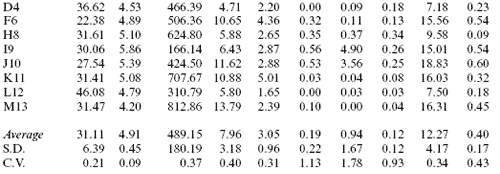
1LA=lactic, AC=acetic, PR=propionic, BT=butyric, IB=isobutyricAmmonia accounted for 7-37% of total nitrogen and was especially high in silages I9 and J10.Within experimental samples, the butyric-type silage also had 20% ammonia. Amines were found to be quite high in several samples, even in some that exhibited rather low ammonia levels. Samples B2, C3, F6, H8, I9 and J10 contained more than 4% total amines, with cadaverine, putrescine and g-aminobutyric the most important (Table 3).
In all experimental silages including the lactic acid one, which presumably underwent a very good fermentation, the amine levels (mainly cadaverine and putrescine) were quite high. The fact that silages with good volatile fatty acid profiles contained significant amounts of biogenic amines may be one of the factors involved in the poor animal responses frequently noted in legume silages (Gill et al., 1987).
These findings, however, would not be supported by Van Os et al. (1995) who found that biogenic amines from silages are degraded by rumen microbes with increasing efficiency as they adapt to diets with high amine content. This feature would prevent passage of amines to the intestine.
Earlier work by Buchanan-Smith (1972) proved, however, that plasma infusion of amines caused a significant intake depression. Bends in the silage titration curves due to the presence of amines (Offer et al., 1994) were clearly shown. Amines have been closely correlated with voluntary intake in the animal. The large amount of nitrogenous compounds found as amines represent reduction in the amino acid fraction, and this may be the main negative effect of amines, particularly if there is a selective utilization of certain labile amino acids for their formation.
Figure 1.Relationship between soluble nitrogen and non-protein nitrogen fractions in farm silages.
Within the NPN fraction the amino acids represent the main compound not accounted for beyond ammonia, amines and ADIN. Interestingly, in high-lactic low-ammonia low-amine silages 25-45% of the total nitrogen was found in this amino acid-rich fraction. Even though this fractionmight contain other compounds like amides or nitrogen oxides not reduced to free ammonia, the size of this fraction may have great significance in the utilization of proteins by ruminants fed silages.
The protein breakdown observed in a set of samples with very distinct fermentation patterns showed that a variable quantity of nitrogenous compounds remained insoluble through silage fermentation.
If ADIN is subtracted from this insoluble fraction, the rest would presumably correspond to high molecular weight true proteins. This fraction ranged from 21 to 44%, being little affected by the quality of the fermentation and quite independent of the amounts of ammonia and amines often used as indicators of the extent of protein breakdown.
This fraction of insoluble proteins preserved in spite of poor silage fermentation may be less degradable, probably due to structural protection within plant tissues or to a combined effect of heating during fermentation. The possibility of acid inhibition of proteases should be ruled out because most of the silages had fairly high pH values, particularly those with poor fermentation.
Table 2.Composition of nitrogenous compounds in alfalfa silages (% of total N).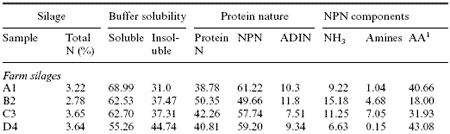
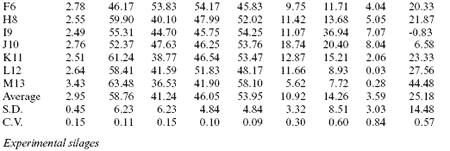
1Amino acid-rich fraction estimated as total NPN minus ADIN, ammonia-N and amine-N.The electrophoretic analyses of extractable proteins showed a massive breakdown of high molecular weight proteins in most silage samples. The large subunit of Rubisco (54 kDa), which is clearly seen in fresh alfalfa, essentially disappeared and very weak and diffuse bands were observed in all samples.
Only in silage M13 did a new and thick band appear at 30 kDa with several smaller bands in other positions (Figure 2). This is consistent with the good fermentation pattern of this sample derived from a crop ensiled very quickly under excellent field conditions using a lactic acid bacterial inoculant. In the other samples most of the protein was washed out of the gel, i.e., under 10 kDa.
Table 3.Amines in alfalfa silages (% of total N).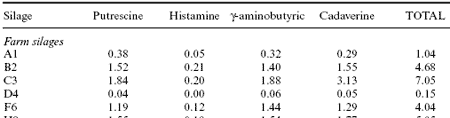
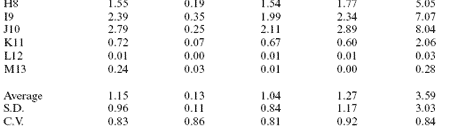
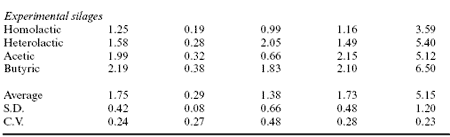
The amino acid profile of farm silages showed a wide variation among samples. Some consistency was found regarding a few amino acids when grouping and comparing the best fermented four silages and the poorest four silages.
It was observed that arginine, alanine and phenylalanine had significantly higher values in poorly preserved silages, but for serine and threonine the reverse was observed (Table 4). For the other amino acids, no relationship could be found with any of the composition and fermentation parameters studied.
In samples of farm silages there was no information about the original composition of the forages ensiled, but if we compare the composition of good silages with reported values for legume forage species (Table 5), it can be observed that arginine, lysine, histidine and aspartic acid are in low levels; in the poorly fermented ones, the same amino acids remain low with the addition of serine. When comparing the amino acid profiles of silages and rumen microbes it was found that aspartic and glutamic acids, arginine, tyrosine, methionine and lysine were very low.
Many of the amino acids show increased values with respect to original forages, but it must be kept in mind that when reporting molar proportions the reduction of any one causes a proportional increase in all the others. In fact, during fermentation some amino acids have been degraded and the values in Table 5 indicate that there was a selective utilization of some. Also it shows that the profile in silage amino acids deviates significantly from the typical composition of rumen microbes that are supposed to provide a high quality protein to the host animal.
Figure 2. Protein electrophoresis of silage extracts with contrasting fermentation patterns.Table 4. Major changes observed in amino acid profile in alfalfa silages with contrasting fermentation patterns1.
1Values between brackets are standard deviations of the mean.In terms of protein quality for high-producing ruminants and the existing concept about the importance of protein solubility, these findings allow an opposing hypothesis for silages in regard to the role of soluble and insoluble proteins and the ‘rumen bypass’ phenomenon.
First, the insoluble nitrogen components, either true protein or NPN, will be found mostly in the particulate fractions of the digesta. These will slowly release nitrogen to the rumen by means of progressive mechanical breakdown (mastication/rumination) and enzymatic activity of bacterial proteases.
Consequently, this insoluble nitrogen fraction would have a low rumen by-pass potential because of the relatively slow particle breakdown rate, and conversely a good chance of being taken up by ruminal bacteria following enzymatic hydrolysis.
Table 5. Amino acid profile in good and poor silages compared with fresh legumes and rumen microbes1.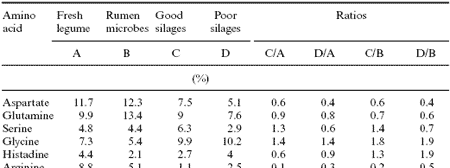
1Good silages are L12, M13, A1 and D4. Poor silages are I9, J10, C3 and B2.Our results, in agreement with the findings of Harrison and Blauwiekel (1994), suggest that in well-preserved silages amino acids and peptides are the most important components of the larger soluble nitrogen fraction. Considering that this fraction can either be used by rumen bacteria or pass through the rumen with the liquid phase, in animals fed silage with a high proportion of soluble N there is the probability this component will pass through the rumen without being taken up by bacteria.
Such ‘escape’ might be enhanced because of the depletion in readily available energy that prevents bacteria from using large amounts of peptides and amino acids, although some of it could be inefficiently used as an energy source. In a theoretical calculation for a cow with a high level of silage intake one can assume a dilution rate of the liquid phase out of the rumen of 12 %/hr, a rate of carbohydrate digestion of 8%/hr and a high microbial yield of 4 gN/100g fermented carbohydrate. Under such conditions a large proportion of soluble nitrogen compounds can escape microbial uptake.
Ammonia can be absorbed across the rumen wall, but amino acids and peptides would be utilized to a lesser degree in the rumen epithelium and may be washed out with the liquid phase thus by-passing the rumen and providing a source of nutrients ready for absorption in omasum (Matthews andWebb, 1995) and duodenum.
The hypothesis of intact peptides flowing post-ruminally has also been suggested by Wallace and Cotta (1988) on the basis of a high peptide accumulation found in rumen. The poor utilization of silage proteins for bacterial synthesis in the rumen reported by Tamminga et al. (1991) and his suggestion about the convenience of wilting grass to over 50% dry matter before ensiling in order to improve protein utilization, may be related to the hypothesis presented here. This is that soluble proteins in well-preserved silages, presumably rich in peptides and amino acids, have a good chance of escaping bacterial uptake in the rumen and would result in less efficient microbial protein synthesis.
A severe wilting would lead to heated silages, reduced solubility of nitrogen compounds and increased ruminal retention time, thus improving the synchrony with the energy made available from slow fermentation of structural carbohydrates. This would in turn improve the efficiency of protein synthesis.
The strategy suggested by these authors is in agreement with the objective of maximizing ruminal protein synthesis, but it fails to recognise that soluble peptides and amino acids may be more efficiently utilized by the host animal if by-passed and not recycled through rumen microbes. Such behaviour of soluble nitrogen compounds in wellpreserved silages, namely peptides and amino acids, is of great nutritional interest although the problem of amino acid balance at that level remains an open question.
ANALYSIS OF ENERGY YIELDING COMPOUNDS FOUND IN FERMENTED SILAGES
Silages are known to consume plant sugars during anaerobic fermentation in quantities dependent on the extent of the fermentation. When fresh, most legume species have low sugar content and consequently the residual quantity after ensiling is very low (or is essentially nil).
The majority of alfalfa silages surveyed (11/15) had less than 1.0 % residual sugars regardless of the quality of the fermentation. The remaining samples (4/15) had between 1.0 and 3.8% sugars. In turn, the residual quantity of starch ranged from 1.6 to 3.6% which is much lower than normal values reported in samples of fresh alfalfa by Van Soest (1994) and Jaurena (1996).
Although some authors have suggested that to a large extent starch is not a fermentable substrate in the silage (Woolford, 1984), these low values confirm the findings of Valinotti and Pichard (1993) who reported that most of alfalfa starch disappeared during ensiling. Endogenous plant amylases may be responsible for this (Goodwin and Mercer, 1990), which might be envisaged as a positive fate as it helps provide fermentable energy in legume silages as long as it yields sugars at early fermentation times.
The total amount of nonstructural carbohydrate was on average 3.2%, ranging from 1.8 to 5.8% (Table 6). These are low values with respect to the high ruminal energy demand for efficient utilization of the large early supply of nitrogen. Other ingredients in the silage that help to support this energy demand are the residual organic acids, mainly succinic, but acids account for only an additional 1.9% of the fermentable dry matter.
Table 6. Residual carbohydrates and organic acids in silages available for rumen fermentation (% of dry matter).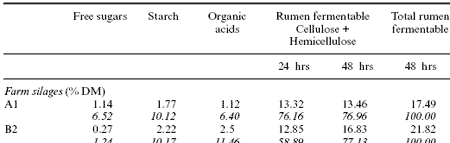
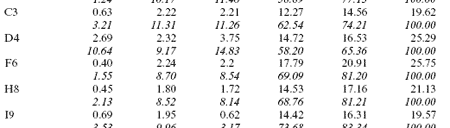
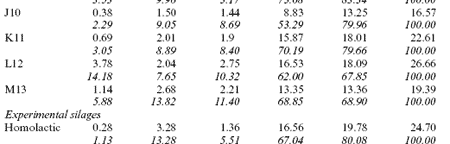
1Italic numbers represent % of total.The amount of readily fermentable components including starch was on average only 5% of the plant weight. This accounts for less than 25% of the total fermentable carbohydrate. In contrast, over 60% of the silage nitrogen is in low molecular weight compounds that will be released in the rumen shortly after consumption.
Most of the fermentable carbohydrates correspond to plant cell wall components which have a reduced availability in the rumen owing to the silage fermentation. In the silo hemicellulose is exposed to acid solubilization of the most labile fractions thus becoming more refractory to rumen bacteria compared to the original forage (Huhtanen and Jaakkola, 1993). This was confirmed in the experimental silages. Plant cell wall digestibility decreased from 52% in the fresh forage to 48 and 42% in silages with lactic and butyric-type fermentations, respectively.
Even though in all alfalfa silages a large proportion of the cell wall fermentation was accomplished by 24 hrs (Table 6), the small quantity of readily fermentable carbohydrate suggests that in early stages of fermentation this source of ATP is limiting and bacteria must take a less efficient route by burning proteins in order to obtain the required energy. The energy pattern observed in these farm and experimental silage samples is well recognised in the RFE system used in the UK for calculation of potential metabolizable energy (AFRC, 1992).
Based on the composition of fresh alfalfa samples reported by Smith (1973), Dijkshoorn (1973), Pichard and Innocenti (1987) and Van Soest (1994), the authors have calculated that the sum of sugars, starch, organic acids and digestible plant cell wall components adds up to 35-40 % of the plant weight with 55% of it derived from structural carbohydrates.
In the silage samples studied, the sum of the same fractions added up to only 22% with 77% of it being structural components (Table 7). This represents a contrasting situation regarding the quantity and quality of energy sources available for rumen fermentation. The fraction that disappeared is partially converted to fatty acids which do not provide energy for microbial growth in the rumen. The results confirm that the pattern of energy supply to the rumen bacteria is much altered as a consequence of silage fermentation.
Table 7.Summary of rumen fermentable carbohydrates and organic acids in alfalfa silages (% of dry matter)1.
1Values between brackets show the contribution (%) of fractions to total fermentable matter.
2Lowest absolute value found among 15 silage samples.
3Highest absolute value found among 15 silage samples.
Agricultural and Food Research Council. 1992. Nutritive Requirements of Ruminant Animals: Protein. Report N° 9, Nutr. Abstr. and Rev., Series B. Vol. 62(12). CAB International,Wallingford, Oxon, England.
A.O.A.C. 1984. Association of Official Analytical Chemists. Official Methods of Analysis. 14th Ed., Washington D.C., USA. Buchanan-Smith, J.G. 1972. Forage protein in ruminant animal production, BSAP Occasional Publication 6:611-617.
Chaney, A.L. and E.P. Marbach, 1962. Modified reagents for the determination of urea and ammonia. Clin. Chem. 8:130-132.
Choung, J.J. and D.G. Chamberlain. 1993. Effects of addition of lactic acid and post-ruminal supplementation with casein on the nutritional value of grass silage for milk production in dairy cows. Grass & Forage Sci. 48:380-386.
Cohen, S.A. 1990. Analysis of amino acids by liquid-chromatography after pre-column derivatization with 4-Nitrophenylisothiocyanate. J. of Chromatography 512:283-290.
Dijkshoorn, W. 1973. Organic acids and their role in ion uptake. In: Chemistry and Biochemistry of Herbage Vol. 2:163-188 (G.W. Butler and R.W. Bailey, eds.) Academic Press, London and New York.
Etheridge, M.O., C.R. Stockdale and P.D. Cranwell. 1993. Influence of method of conservation of lucerne on factors associated with voluntary intake in sheep. Australian J. Exp. Agric. 33:417-423.
Fussell, R.J. 1987. Determination of volatile fatty acids (C2-C5) and lactic acid in silage by gas chromatography. Analyst 112:1213-1216.
Gill, M., L.R.S. Thiago and J.G. Buchanan-Smith. 1987. Intake problems associated with ensiled forages. In: Feed Intake by Beef Cattle (F.N. Owens, ed.) Oklahoma State University Misc. Publ. 121:341-352.
Goering, K.H. and P.J Van Soest. 1970. Forage fiber analysis. USDAARS, Agric. Handbook 379.
Goodwin, T.W. and E.I. Mercer. 1990. Introduction to Plant Biochemistry. 2nd Ed., Pergamon Press, Oxford, New York.
Harrison, J.H. and R. Blauwiekel. 1994. Fermentation and utilization of grass silage. J. Dairy Sci. 77:3209-3235.
Huhtanen, P. and S. Jaakkola. 1993. The effects of forage preservation method and proportion of concentrate on digestion of cell wall carbohydrates and rumen digesta pool size in cattle. Grass and Forage Sci. 48:155-165.
Jaurena, G. 1996. Analisis de la contribucion de los granos como aditivos para ensilajes de alfalfa. Tesis grado de Magister, P. Universidad catolica de Chile.
Jones, R., A.Winters and J. Cockburn. 1996. Change in amino acid content of inoculated grass silage and its effect on animal production. In: Biotechnology in the Feed Industry. Proc. 12th Annual Symposium (T.P. Lyons and K.A. Jacques, eds). Nottingham University Press, Nottingham, Leics. UK. pp. 249-259.
Karkalas, J. 1985. An improved enzymatic method for the determination of native and modified starch. J. Sci. Food and Agric. 36:1019-1027.
Krizek, M. 1993. Biogenic amines in silage. 2. The occurrence of biogenic amines in silages. Arch Tierernahr. 43(2): 169-177.
Laemmli, U.K. 1970. Cleavage of structural proteins during the assembly of the head of bacteriophage T4. Nature, Aug. 15, 227(259):680-685.
Lobos, S. and S.G. Mora. 1991. Alteration in the electrophoretic mobility of OmpC due to variation in the ammonium sulphate concentration in sodium dodecyl sulphate policrylamide gel electrophoresis. Electrophoresis 12:448-450.
Matthews, J.C. and K.E. Webb, Jr. 1995. Absorption of L-carnosine, L-methionine and L-methionylglycine by isolated sheep ruminal and omasal epithelial tissue. J. Anim. Sci. 73:3464-3475.
McDonald, P., A.R. Henderson and S.J.E. Heron. 1991. The biochemistry of silages, 2nd Ed., Chalcombre Publications, U.K.
McDonald, P. and A.R. Henderson. 1964. Determination of watersoluble carbohydrates in gass. J. Sci. Food Agric. 15:395-398.
McRae, G. 1983. Starch analysis. In: Methods for the analysis of feeds eaten by ruminants. Division of Animal Production, Ian Clunies Rooss Animal Res. Lab., CSIRO, Melbourne, Australia.
Offer,N.W., R.J. Dewhurst and C. Thomas. 1994. The use of electrometric titration to improve the routine prediction of silage intake by lambs and dairy cows. Proc. 50thWinter Meeting, British Society of Animal Production, Scarborough, Paper 1.
Playne,M.J. and P. McDonald. 1966. The buffering constituents of herbage and of silage. J. Sci. Food Agric. 17:264-268.
Pichard, G. 1977. Forage nutritive value. Continuous and batch rumen in vitro fermentation and nitrogen solubility. PhD Thesis, Cornell University, Ithaca, New York.
Pichard, G. and E. Innocenti. 1987. Tabla de composicion de forrajes de la zona central de Chile. Ciencia e Inv. Agr. 14(2):143-158.
Pichard, G. and G. Rybertt. 1993. Degradacion de las proteınas en el proceso de ensilaje. Ciencia e Inv. Agr. 20 (2):402–429.
Rooke, J.A. and V.L. Nsereko. 1993. Peptide nitrogen in grass silages. In: Proc. 10th Intl. Conf. on Silage Research, Dublin, pp. 62-63.
Schneider, A., V. Gerbi and M. Redoglia. 1987. A rapid HPLC method for separation and determination of major organic acids in grapes,musts and wines. Am. J. Enol. Viticult. 38(2):151-155.
Smith, D. 1973. The nonstructural carbohydrates. In: Chemistry and Biochemistry of Herbage, Vol. 1:106-156. (G.W. Butler and R.W. Bailey, eds.) Academic Press, London and New York.
Tamminga, S., R. Ketelaar and A.M. Van Vuuren. 1991. Degradation of nitrogenous compounds in conserved forages in the rumen of dairy cows. Grass and Forage Sci. 46:427-435.
Tan, L.U.L., M.K.H. Chan and J.N. Sadler. 1984. A modification of the Lowry method for detecting protein in media containing lignocellulosic substrates. Biotechnol. Lett. 6(3):199-204.
Valinotti, P. and G. Pichard. 1993. “Efecto de la inoculacion bacteriana sobre la fermentacion del ensilaje de alfalfa fresca suplementada con carbohidratos solubles”. XIII Reunion Asociacion Latinoamericana de Produccion Animal. (ALPA). Ciencia e Investigacion Agraria. Mayo- Agosto. 22 (2):38-39.
Van Os, M., B. Lassalas, S, Toillon. and J.P. Jouany. 1995. In vitro degradation of amines by rumen micro-organisms. J. of Agric. Sci. Camb. 125:299-305.
Van Soest, P.J., J.B. Robertson and B.A. Lewis. 1991. Methods for dietary fiber, neutral detergent fiber, and nonstarch polysacharides in relation to animal nutrition. J. Dairy Sci. 74:3583-3597.
Van Soest, P.J. 1994. Nutritional Ecology of theRuminant. 2nd Ed., Cornell University Press, Ithaca, New York
Wallace, R.J. and M.A. Cotta. 1988. Metabolism of nitrogen-containing compounds. In: The rumen microbial ecosystem (P.N. Hobson, ed.), Elsevier Applied Science, London and New York.
Wilkinson, M. 1990. Sampling and analysing silage. In: Silage U.K., 6th Edition. Chalcombe Publications.
Woolford, M.K. 1984. The silage fermentation. Microbiology Series Volume 14, Marcel Dekker, Inc., New York. 13