1. Introduction
The pelleting operation is one of the most expensive processes with regards to the fixed and variable costs. For this reason, efficient pellet production is aimed and pellet press should deliver a high yield of dust-free and resistant pellets to optimize the feed mill operations. Besides the types of ingredients, amount of added fat, feed particle size, die’s compression rate, conditioning temperature and moisture or binders inclusion, of which effects have been extensively reported by many authors [1-13], other factors, such as expander heat treatment, roller to die distance and press throughput, also affect pellet physicochemical properties.
Briggs et al. [5] observed that increasing press throughput, but keeping the other production parameters constant, resulted in a loss of pellet physical quality. Probably the faster feed passage rate through the conditioner and consequently a shorter steam exposure time negatively affected the feed conditioning. Lowe [14] also mentioned that an impairment of pellet physical quality should be expected with higher production rate due to reduced feed retention time inside the press die channels, which negatively affects compression rate and starch gelatinization.
A larger pressure applied to the feed inside the die hole favors the aggregation of feed particle, supporting well-consolidated pellet formation. The slot between the roller and die is where the initial compression of conditioned feed takes place. Thus, a wider roller-die gap results in an extra feed compression prior its entry into the die channels, and this additional compacting force enhances pellet density and resistance [15]. However, if this gap is too wide, the press production rate is excessively reduced, and as a consequence, darkened and overcooked pellets may appear due to prolonged feed retention time in the die or even pellet press choke up may occur. Each pellet press has its ideal gap where the pellet physical quality is optimized. According to Lara [16], the distance between the roller and die should be around 1.5 mm to 2.0 mm to optimize pellet quality.
Increasing the thermal processing degree by means of feed expansion is another validated procedure to improve pellet quality. Muramatsu et al. [12] observed that conditioning-expanding-pelleting process led to an additional 205 g of intact pellet/kg of feed when compared to a conventional conditioning-pelleting process. In a similar way, Lundblad et al. [17] compared pellet quality in feed conditioned at 82 °C for 20 s versus feed submitted to the same conditioning parameters but further expanded at 121 °C. These authors reported that the extra heat treatment led to an enhancement in pellet durability index (PDI) from 81.8% to 92.3%, when compared to simple conditioned-pelleted diets. On the other hand, the aggressive heat treatment in expander induces greater denaturation of dietary proteins. Therefore, conditioned-expanded feed show reduced in vitro assessment of the feed protein solubility in KOH, when compared to conditioned feed [12]. Veloso et al. [18] observed that after an expander treatment at 130-136 °C, soybean meal protein solubility in KOH decreased from 80.6% to 64.1%.
Some strategies can be implemented to enhance feed pellet manufacturing. The productivity and efficiency of the pellet mill may be improved by correct application of these tools. The objective of this study was to evaluate the effect of thermal processing, press throughput and roller-die gap on physicochemical properties of broiler feed pellets.
2. Material and Methods
2.1 Feed Processing
The experimental diets were manufactured in a feed mill located in Goiás State, Brazil. The feed was a standard broiler grower diet, containing corn, soybean meal and animal by-products (Table 1). The ingredients were first directed to a pre-grinding sieve, and all the coarse ingredients that did not pass through this screen were ground in a hammer mill. All diet components were blended in a paddle type mixer (6,000 kg capacity). Mixing time was divided in three phases: dry mixing (45 s), liquid addition (60-90 s) and wet mixing (25 s).
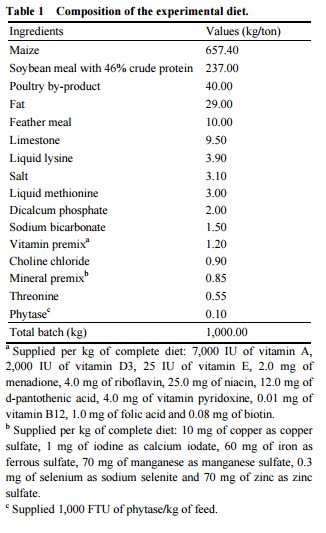
For the conditioned-expanded-pelleted feed, diets were steam-conditioned for 30 s at 80-82 °C under a steam pressure of 1.5-2.0 bar. The mash was then transported to an expander, with average mash retention time of 5 s and an average temperature of 110 °C and to the pellet press (die specifications: 660 mm diameter, 60 mm deep and 4.5 mm diameter die holes, without relief). For the simple conditioned-pelleting treatment, diets were steam-conditioned and then transported to the pellet press using the same equipment parameters that were used for the conditioned-expanded-pelleted treatment.
2.2 Production and Sampling of Test Feed
A total of 1,440 tons (240 batches of 6 tons) of a broiler diet was manufactured in this experiment. The three main factors (two thermal processes, three roller-die gap and five press throughput) were combined in a 2 × 3 × 5 factorial arrangement in an eight randomized block design consisting of eight production series, totaling 30 different combinations. These combinations were repeated in eight production series distributed along the experimental period.
The sampling point was between the pellet press and the cooler. Eight samples of pelleted feed (one sample per production series), corresponding to eight replicates, were collected for each experimental treatment for determination of amount of intact pellets (%), PDI (%), protein solubility in KOH (% of the total protein content) and degree of starch gelatinization (% of total starch content). These pelleted feed samples were cooled under environmental conditions for 24 h before the chemical analysis.
2.3 Feed Analysis
Feeds from the different treatments were analyzed for the following chemical parameters:
(1) PDI: determined according to method S269.4 from ASAE standards [19];
(2) Amount of intact pellets: 200 g of feed is sieved in a screen of 3.0 mm round holes screen and the portion of feed that is retained in this screen is considered as intact pellets;
(3) Protein solubility in KOH: determined according to method described by Parsons et al. [20];
(4) Amount of gelatinized starch (% of total starch): determined according to method 27 of Compendio Brasileiro De Nutrição Animal [21];
(5) Moisture content: determined according to the method 930.15 referenced at the Association of Official Analytical Chemist International [22];
(6) Particles size: determined according to the method referenced by the American Society of Agricultural and Biological Engineers [23].
2.4 Statistical Analysis
The statistical model included type of thermal processing, roller-die gap, press throughput and interactions between factors, as Eq. (1):
Yijklm = µ + TPi + RDGj + PTk + (TPi × RDGj) + (TPi × PTk) + (RDGj × PTk) + (TPi × RDGj × PTk) + εijkl (1)
where, Yijklm = dependent variable (amount of intact pellet or PDI or protein solubility in KOH or degree of starch gelatinization), µ = the population mean, TPi = effect of thermal processing (i = conditioned-expanded-pelleted or conditioned-pelleted), RDGj = effect of roller-die gap (j = 0.50, 0.75 or 1.00 mm), PTk = effect of press throughput (k = 18 ton/h to 30 ton/h) and εijkl = residual error.
A general linear model was employed to analyze the effects of categorical and quantitative factors present in the statistical model. Analysis of variance and multiple regression tools of Statgraphics Centurion XVI (Stat Point Technologies, Inc.) and Statistica 8.0 version (StatSoft, Inc.) were used to perform the analyses of the collected data. The Anderson-Darling test from Minitab version 16 was used to check the normality of the residuals of the estimated model for the starch gelatinization. According to Razali and Wah [24], the Shapiro-Wilk test and Anderson-Darling test are the most powerful normality test, followed by Lilliefors test and Kolgomorov-Smirnov test. Linear and quadratic effects of press throughput were determined by multiple regression associated with backward elimination method. The factors and their interactions (TP × RDG, TP × PT, RDG × PT and TP × RDG × PT) were excluded from the final regression equation when P > 0.05. The factors type of thermal processing and roller-die gap were included in the regression as dummy variables (TP = 1 when conditioned expanded-pelleted, 0 when conditioned-pelleted). The significance of the performed tests were accepted when P ? 0.05.
3. Results and Discussion
The average moisture content of mash feed samples prior to conditioning was 10.86% and diet’s particle sizes achieved in the test diets was 741 μm. The data concerning amount of intact pellets (%) and protein solubility in KOH (%) did not have normal distribution (Anderson-Darling test, P < 0.05). Yeo and Johnson [25] reported a type of Box-Cox transformation that was capable to the approximate database to normality. In the present study, this procedure, known as Johnson transformation, was used to fit these data to Gaussian distribution, as Eqs. (2) and (3):
Amount of intact pellets (%) = -1.40849 + 1.10279 × ln[(amount of intact pellets% – 74.1136)/(100.313 – amount of intact pellets%)] (2) Protein solubility in KOH (%) = 0.592168 + 1.37685 × ln[(protein solubility in KOH – 59.9674)/(93.5563 – protein solubility in KOH)] (3)
Data were initially analyzed according to the original statistical model (Table 2) and then backward elimination method was employed to remove non-significant factors from the prediction equations (Tables 3-6). The resultant models presented R2 values close to that of the entire model for amount of intact pellet (0.69 and 0.65, respectively, for the entire and backward models), PDI (0.88 and 0.85, respectively, for the entire and backward models), protein solubility in KOH (0.62 for both entire and backward models) and degree of gelatinized starch (0.25 for both entire and backward models).
3.1 Thermal Processing
The feed submitted to conditioning-expanding pelleting treatment returned a greater amount of intact pellet and PDI (95.6% and 90.3%, respectively), as compared to conditioning-pelleting treatment (90.6% and 77.8%, respectively). Similar results were observed in the previous study done by Muramatsu et al. [12], where pellets from conditioned feed at 82 °C for 15 s were compared to those manufactured from conditioned feed under the same parameter and sequentially submitted to expander at 110 °C for 5 s. These authors reported that the use of expander combined with conditioner reduced the amount of fines in pelleted feed up to 200 g/kg of feed, and PDI was enhanced from 69% to 87% when compared to single conditioning. Also, a survey held in North American feed manufacturers indicated that diets processed on expander before pelleting when compared to single conditioning-pelleting improved pellet quality by 15%-25% [26]. In these two studies, as the expander treatment imposed greater heat transfer and shear force to the feed, a higher starch gelatinization and conditioning were ended in better pellet physical quality.
3.2 Roller-Die Gap
Although the differences between pellet physical parameters were not extensive, the 0.5 mm roller-die gap returned the finest pellet quality (95% intact pellets and 86% PDI) compared to 0.75 mm and 1.00 mm which attained 92% and 93%, respectively, for amount of intact pellets, and 82% and 84%, respectively, for PDI. The data reported in this experiment differ from that found by Miladinovic and Svihus [15]; these authors compared roller-die gaps ranging from 0.10 mm to 2.00 mm, and they reported a significant PDI improvement (P < 0.05) achieved with the ultimate setting (84.2% and 88.1% PDI, respectively, for 0.10 mm and 2.00 mm gap). This distinct trend between the trials probably is due to the fact that the PDI enhancement was a mixed effect of two factors: the largest roller-die distance (2.0 mm) which resulted in the highest PDI, and a 50% press throughput reduction which increased feed passage time in the conditioner pellets was diminished with increasing roller-die gap (98.1%, 95.7% and 94.5% intact pellets, respectively, for 0.50, 0.75 and 1.00 mm gap); whereas in single conditioned feed, the lowest amount of intact pellet was achieved in the 0.75 mm settings (92.8%, 88.7% and 91.6% intact pellets, respectively, for 0.50, 0.75 and 1.00 mm gap).
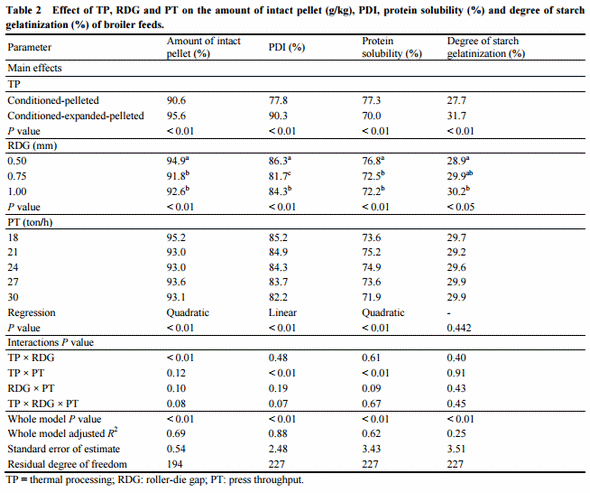
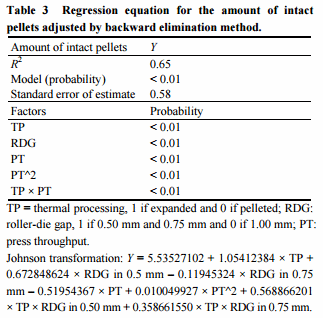
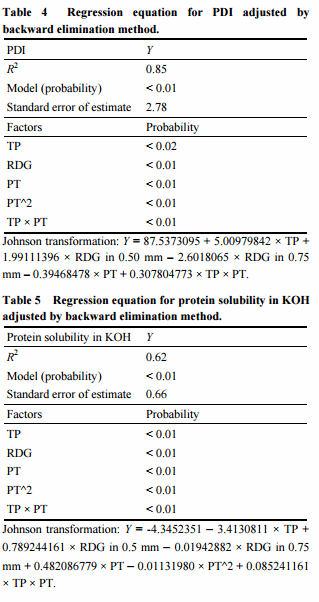
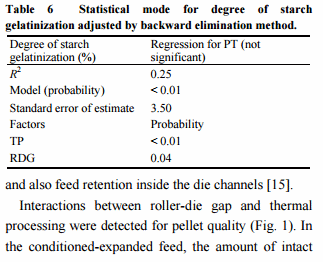
3.3 Press Throughput
The current research identified an impairment of pellet physical quality, when press production rate was increased. PDI was linearly decreased (P < 0.01) from 85.2% to 82.2%, when press throughput was increased from 18 ton/h to 30 ton/h. The amount of intact pellet showed a quadratic trend (P < 0.01) and the values ranged from 95.2% to 93.1% pellets, when press throughput varied from 18 ton/h to 30 ton/h. These findings are in accordance with Behnke [27] and Lowe [14], who mentioned that high press throughput is detrimental for manufacturing of stable and resistant pellets. In the same way, Briggs et al. [5] observed that when the press production rate in a specific diet increased from 1,100 kg/h to 1,460 kg/h, the PDI of the respective pellets declined from 55.8% to 28.6% and the rate of fines raised from 120 kg/h to 311 kg/h. Finally, Miladinovic and Svihus [15] reported a PDI increase (84.7% up to 88.0%), when the press yield was scaled down in 50%.
Both conditioned-expanded and single conditioned pellet’s PDI was linearly impaired (P < 0.05) by higher press output. PDI slightly changed from 90.7% to 89.8% with an increase of production rate from 18 ton/h to 30 ton/h (Fig. 2). The single conditioned treatment presented a more pronounced negative effect caused by press throughput. PDI decreased from 79.8% to 74.6% along the 18 ton/h to 30 ton/h output range. Possibly, in the conditioned-expanded feed, the higher processing temperature and shear forces induced enough chemical changes (starch gelatinization, protein denaturation) to compensate the minor retention time in the higher output rates.
3.4 Protein Solubility in KOH
Protein solubility in KOH of mash feed samples collected before thermal processing had an average value of 78.8% and decreased after feed exposure to heat treatment. As expected, protein solubility loss was evident in conditioning-expanding-pelleting treatment (70.0%), whereas in conditioning-pelleting treatment, the damages were the minimum (77.3%). Muramatsu et al. [12] also described reductions in protein solubility when diets were submitted to thermal processing. These authors compared conditioning-expanding-pelleting, where feed was firstly conditioned at 82 °C for 15 s and then expanded at 110 °C for 5 s, with conditioning-pelleting process, and observed a greater loss in the expander than in single conditioning (64.5% versus 68.6% protein solubility in KOH). In accordance to these two studies, when soybean meal was expander treated at 130-136 °C, the protein solubility in KOH decreased from 81% to 64% [18].
Protein solubility was also reduced (P < 0.01), when roll-die gap was increased from 0.50 mm to 0.75 mm or 1.00 mm (means were, respectively, 76.8%, 72.5% and 72.2% for the crescent distances). Miladinovic and Svihus [15] mentioned that as the roller-die distances increase, the multiple layers of feed allocated in this space increase the coefficient of friction between the die and the feed. Also, the authors pointed out that larger roller-die distance results in a pressing angle, which maximize the pressure over the feed entering the die channels. This combination of pressure and friction may have generated extra heat which denatured the dietary proteins.
3.5 Starch Gelatinization
Mean starch content of the samples was 491.3 g/kg of feed. The samples of mash feed collected at the mixer discharge, previous to thermal processing, already presented 10.9% gelatinized starch. These findings are in accordance to data reported by Muramatsu et al. [28]. Once cereals grains, after harvest, are frequently submitted to hot air drying in order to achieve appropriate moisture content for storage, starch granules may get some starch gelatinization. Malumba et al. [29] and Yang et al. [30] reported that when starch granules were submitted to hot air above 80 °C, the thermal processing led to starch granules swelling and weaken Maltese crosses, suggesting the occurrence of starch pre-gelatinization.
In the present study, the expander induced a higher (P < 0.01) starch gelatinization rate (31.7% of total starch was gelatinized) when compared to single conditioning (27.7%). Goelema et al. [31] and Muramatsu [28] also verified that expander treatment promoted larger gelatinization rate of dietary starch regards to single conditioning. The roller-die gap evaluated in this study also affected rate of starch gelatinization. Feed processed with 1.00 mm roller-die distance presented 30.2% of gelatinized starch, which were significantly greater (P < 0.05) than the 28.9% starch gelatinization rate observed with the 0.50 mm gap. The stronger pressure and friction rates generated by wider roller-die gap may have intensified starch granules disruption.
4. Conclusions
This study indicates that conditioning-expandingpelleting treatment, narrower roller-die gap and reduced press throughput are effective strategies to enhance pellet physical quality. Protein solubility in KOH was decreased and starch gelatinization rate was enhanced, when feed was submitted to expander process. The wider roller-die gap may increases coefficient of friction and pressure force over the feed prior its entry to die channel, which increases starch granules disruption (higher starch gelatinization degree) and protein denaturation (lower protein solubility in KOH).
References
1] Dozier III, W. A. 2001. “Cost Effective Pellet Quality for Meat Birds.” Feed Management 52 (2): 1-3.
[2] Franke, M., and Rey, A. 2006. “Improving Pellet Quality and Efficiency.” Feed Tech. 10 (3): 12-5.
[3] Mendez, J. A. R., and Santomá, G. 2008. “Feed Manufacturing.” In Nutrition of the Rabbit, 2nd ed., edited by De Blas, C., and Wiseman, J. UK: CPI Antony Rowe Ltd., 200-21.
[4] Thomas, M., and Van der Poel, A. F. B. 2000. “Physical Quality of Pelleted Animal Feed: Part 1, Criteria for Pellet Quality.” Anim. Feed Sci. Technol. 61: 89-112.
[5] Briggs, J. L., Maier, D. E., Wakins, B. A., and Behnke, K. C. 1999. “Effect of Ingredients and Processing Parameters on Pellet Quality.” Poult. Sci. 78 (10): 1464-71.
[6] Moritz, J. S., Wilson, K. J., Cramer K. R., Beyer. R. S., McKinney, L. J., Calvalcanti, W. B., and Mo, X. 2002. “Effect of Formulation Density, Moisture and Surfactant on Feed Manufacturing, Pellet Quality and Broiler Performance.” J. Appl. Poult. Res. 11 (2): 155-63.
[7] Kulig, R., and Laskowski, J. 2008. “Effects of Conditioning Parameters on Pellet Temperature and Energy Consumption in the Process of Plant Material Pressing.” Teka Komisji Motoryzacji i Energetyki Rolnictwa 8: 105-11.
[8] Fahrenholz, A. C. 2012. “Evaluating Factors Affecting Pellet Durability and Energy Consumption in a Pilot Feed Mill and Comparing Methods for Evaluating Pellet Durability.” Ph.D. thesis, Kansas Universtiy, Manhattan, Kansas.
[9] Fairfield, D. A. 2003. “Pelleting for Profit.” Feed and Feeding Digest 54 (6): 1-5.
[10] Abdollahi, M. R., Ravindran, V., Wester, T. J., Ravindran, G., and Thomas, D. V. 2012. “Effect of Improved Pellet Quality from the Addition of a Pellet Binder and/or Moisture to a Wheat-Based Diet Conditioner at Two Different Temperatures on Performance, Apparent Metabolisable Energy and Ileal Digestibility of Starch and Nitrogen in Broilers.” Anim. Feed Sci. Technol. 175 (3-4): 150-7.
[11] Prestlokken, E., and Fôrutvikling, F. 2012. “HFE 305 Feed Manufacturing Technology: Expander Treatment.” Accessed February, 2012. http://www.umb.no/statisk/iha/kurs/nova/feed_technolog y/4.pdf.
[12] Muramatsu, K., Maiorka, A., Vaccari, I. C. M., Reis, R. N., Dahlke, F., Pinto, A. A., Orlando, U. A. D., Bueno, M., and Imagawa, M. 2013. “Impact of Particle Size, Thermal Processing, Fat Inclusion and Moisture Addition on Pellet Quality and Protein Solubility of Broiler Feeds.” J. Agri. Sci. Tech. A 3: 1017-28.
[13] Muramatsu, K., Massuquetto, A., Dahlke, F., and Maiorka, A. 2015. “Factors that Affect Pellet Quality: A Review.” J. Agri. Sci. Tech. A 9 (2): 717-22.
[14] Lowe, R. 2005. “Judging Pellet Stability as Part of Pellet Quality.” Feed Tech. 9 (2): 15-9.
[15] Miladinovic, D., and Svihus, B. 2005. “Optimising Press Settings Contributes to Better Pellet Quality.” Feed Tech. 9 (5): 15-17.
[16] Lara, M. A. M. 2011. “Feed Production Process— Grinding, Mixing and Pelleting.” Accessed February 20, 2016. http://nftalliance.com.br/artigos/ebooks/processo -de-produ-o-de-ra-o-moagem-mistura-e-peletiza-o. (in Portuguese)
[17] Lundblad, K. K., Hancock, J. D., Behnke, K. C., Prestløkken, E., McKinney, L. J., and Sørensen, M. 2009. “The Effect of Adding Water into the Mixer on Pelleting Efficiency and Pellet Quality in Diets for Finishing Pigs without and with Use of an Expander.” Anim. Feed Sci. Technol. 150 (3-4): 295-302.
[18] Veloso, J. A. F., Medeiros, S. L. S., Arouca, C. L. C., Rodriguez, N. M., Saliba, E. O. S., and Oliveira, S. G. 2005. “Chemical Composition, Physico-Chemical and Nutritional Evaluation and the Effect of Expander Treatment on Corn and Soybean Meal or Growing Pigs.” Arq. Bras. M. Vet. Zoot. 57 (5): 623-33. (in Portuguese)
[19] Mina-Boac, R. J., Maghirang, G., and Casada, M. E. 2006. “Durability and Breakage of Feed Pellets during Repeated Elevator Handling.” Presented at ASABE Annual International Meeting, 2006, Portland, Oregon.
[20] Parsons, C. M., Hashimoto, K., Wedekind, K. J., and Baker, D. H. 1991. “Soybean Protein Solubility in Potassium Hydroxide: An in Vitro Test of in Vivo Protein Quality.” J. Anim. Sci. 69 (7): 2918-24.
[21] Sindirações. 2009. Brazilian Compendium in Animal Nutrition. (in Portuguese)
[22] Association of Official Analytical Chemists International (AOAC). 1998. Method in Official Methods of Analysis, 16th ed.. Arlington, VA: AOAC.
[23] American Society of Agricultural and Biolgical Engineers (ASABE). 2006. Method of Determining and Expressing Fineness of Feed Materials by Sieving. American National Standard Institute S319.3.
[24] Razali, N. M., and Wah, Y. B. 2011. “Power Comparisons of Shapiro-Wilk, Kolgomorov-Smirnov, Lilliefors and Anderson-Darling Tests.” J. Statistic. Model. Anal. 2 (1): 21-33.
[25] Yeo, I. K., and Johnson, R. A. 2000. “A New Family of Power Transformations to Improve Normality or Symmetry.” Biometrika 87 (4): 954-9.
[26] Fancher, I., Rollins, D., and Trimbe, B. 1996. “Feed Processing Using the Annular Gap Expander and Its Impact on Poultry Performance.” J. Appl. Poult. Res. 5: 386-94.
[27] Behnke, K. C. 2005. The Art (Science) of Pelleting. Singapore: American Soybean Association.
[28] Muramatsu, K., Maiorka, A., Dahlke, F., Lopes, A. S., and Pasche, M. 2014. “Impact of Particle Size, Thermal Processing, Fat Inclusion and Moisture Addition on Starch Gelatinization of Broiler Feeds.” Rev. Bras. Cienc. Avic. 16 (4): 367-74.
[29] Malumba, P., Janas, S., Roiseux, O., Sinnaeve, G., Masimango, T., Sindic, M., Deroanne, C., and Béra, F. 2010. “Comparative Study of the Effect of Drying Temperatures and Heat-Moisture Treatment on the Physicochemical and Functional Properties of Corn Starch.” Carb. Polymers. 79 (3): 633-41.
[30] Yang, J., Wang, H. Y., Gao, Y., Li, J., and Hu, B. 2011. “Influence of Hot Air and Natural Drying on Extrusion Properties of Maize.” Presented at ASABE Annual International Meeting, ASABE, 2011, Louisville, Kentucky.
[31] Goelema, J. O., Smits, A., Vaessen, L. M., and Wemmers, A. 1999. “Effects of Pressure Toasting, Expander Treatment and Pelleting on in Vitro and in Situ Parameters of Protein and Starch in a Mixture of Broken Peas, Lupins and Faba Beans.” Anim. Feed Sci. Technol. 78 (1-2): 109-26.