Ideally, the starch from cereal grains will be digested by amylolytic enzymes in the small intestine and absorbed as glucose from the pre-caecal section of the gastrointestinal tract. Starch digestion in the small intestine of the monogastric occurs via a three-step process, beginning with the degradation of starch by the enzyme α−amylase. α−Amylase breaks the two principal forms of starch, amylose and amylopectin, into disaccharide (maltose), trisaccharide (maltotriose) and α−dextrin units. The second phase of starch digestion involves the hydrolysis of maltose, maltotriose and α−dextrin units by small intestinal brush border glycanases, primarily amyloglucosidase (AMG), to form free glucose units (Gray, 1992). Na+-dependent active transport and Na+-independent facilitated diffusion then transport glucose from the lumen of the small intestine, through intestinal epithelial cells and into the bloodstream of the animal (Bird et al., 1996; Huntington, 1997; Thorens, 1993).
While cereal grains provide an essential source of energy for working and performance horses, their incorporation into equine diets was not without consequence. Starch not digested by enzymes in the small intestine of the horse will be delivered to the hindgut where it will undergo bacterial fermentation. It is estimated that starch fermented by microorganisms in the hindgut yields 35–40% less metabolisable energy than if digested by small intestinal glycanases and absorbed as glucose in the small intestine (Livingstone et al., 1987 in Kienzle, 1994; Black, 1971;). Furthermore, starch that escapes small intestinal digestion will be rapidly fermented by amylolytic bacteria in the hindgut, causing radical changes to the constitution of hindgut flora and a subsequent increase in the volatile fatty acid and lactic acid concentrations in the caecum and colon (Garner et al., 1978).
The resulting increase in acidity in the hindgut causes a condition commonly known as hindgut lactic acidosis (Garner et al., 1977; Garner et al., 1978; Godfrey et al., 1992; Rowe et al., 1994). Under these acidic conditions, the activity of cellulolytic bacteria is reduced, thereby decreasing fibre fermentation in the hindgut (de Fombelle et al., 1999; Russell and Wilson, 1995). Lactic acidosis has also been repeatedly associated with the onset of equine laminitis, a crippling and potentially careerand/ or life-ending disease of horses (Garner et al., 1975; Garner et al., 1977; Pollitt, 2001; Rowe et al., 1995) as well as with endotoxemia, metabolic acidosis (Krueger et al., 1986; Radostits et al., 2000) and behavioural problems such as wood chewing and wind sucking (Johnson et al., 1998; Rowe et al., 1995; Willard et al., 1977).
Thus, to improve the efficiency of cereal grain feeding to horses and to prevent diseases and behavioural problems associated with the fermentation of starch in the hindgut, starch digestion in the small intestine must be maximised. At present we use grain selection and grain processing as management tools to ensure that a high proportion of starch is digested in the small intestine. It is possible however, that a low activity and sub-optimal concentrations of α−amylase in the equine small intestine will limit the digestion of cereal grain starch, even following grain processing (Kienzle et al., 1994; Richards, 2003; Roberts, 1974). Thus dietary enzyme supplementation may be useful to overcome endogenous enzyme deficiencies and enhance small intestinal starch digestion in the equine.
Small intestinal digestion of common cereal grainsThe estimated small intestinal digestion of starch from common cereal grains appears to be highly variable between and within grain species (Table 1). Among the cereal grain species, oats appears to be the most digestible cereal grain in the equine small intestine, being ranked consistently higher than barley and corn by several researchers (Table 1).
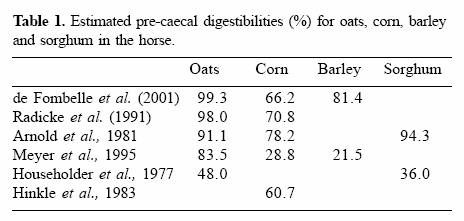
In vitro studies, using a modification of the method of Bird et al. (1999), which involved the incubation of 100 mg of a ground grain in the presence of excess α-amylase (from Bacillus licheniformis) and AMG (from Aspergillus niger), at pH 7 for 15 minutes at 39°C, also indicated that oats contain starch that is more susceptible to small intestinal enzyme degradation than barley and corn (Figure 1). Triticale, a hybrid of wheat and rye, also appears to contain starch that is susceptible to small intestinal enzyme degradation, while the starch in corn and white rice appears to be largely resistant to enzyme attack (Figure 1) (Richards, 2003).
Characteristics of cereal grains, such as the seed coat (Rowe et al., 1999), starch granule and biochemical structure (Kotarski et al., 1992), endosperm cell wall structure (Choct, 1995) and protein matrix structures (McAllister et al., 1993; Rooney and Plugfelder, 1986) will all influence the digestion of cereal grain starch by amylolytic enzymes in the small intestine. To maximise starch digestion in the equine small intestine, these physical and chemical barriers to enzyme degradation must be removed to allow small intestinal amylolytic enzymes access to the starch within cereal grains.
Figure 1. The quantity of starch digested from corn, rice, barley, oats and triticale during an in vitro starch digestion assay where grains are incubated in the presence of excess α-amylase and amyloglucosidase for 15 minutes at 39°C (Richards, 2003).The use of grain processing to improve the small intestinal digestion of starchGrain processing aims to maximise small intestinal starch digestion by changing the physical and chemical structure of grains. Mechanical grain processing methods aim to change the macrostructure of the grain, breaking the seed coat to allow starch digesting enzymes access to the starchy endosperm and increasing the surface area available for enzymatic attack (Kienzle et al., 1997; Rowe et al., 1999). Processing methods utilising a combination of heat, moisture and pressure disrupt starch granule structure, destroy crystalline starch formations, increase the water solubility of starch and physically expose starch to digestive enzymes (Kienzle et al., 1997; Rowe et al., 1999). These processing methods also destroy protein matrix and cell wall structures within the starchy endosperm, allowing digestive enzymes easier access to starch granules (Rowe et al., 1999).
While the effects of grain processing on starch digestibility and production performance in ruminants (Gaebe et al., 1998; Joy et al., 1997; Knowlton et al., 1998; Rowe et al., 1999; Theurer, 1986; Theurer et al., 1999; Zinn, 1993; Zinn et al., 1995), and on small intestinal starch digestion in humans (Brand et al., 1985; Holm et al., 1989; Holt and Brand Miller, 1994; Muir et al., 1995; Snow and O’Dea, 1981; Thorne et al., 1983) have been extensively studied and show that starch digestion in these species is enhanced via grain processing, the research into the effects of grain processing on precaecal starch digestion in equines is limited. In an experiment conducted to determine the effect of grain processing on the digestibility of corn in the equine small intestine, it was observed that as the severity of processing increased, the extent of starch digestion was improved (Table 2, Meyer et al., 1993).
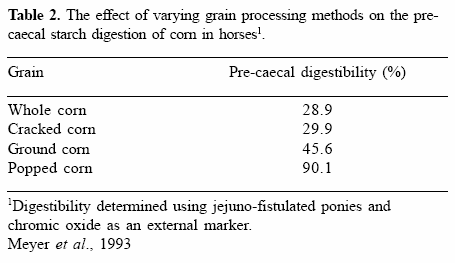
Similar benefits of grain processing on the small intestinal digestibility of corn have been reported by Hoekstra et al. (1999). Using the glycaemic response as an indirect measure of small intestinal starch digestion, these authors reported that horses consuming steam-flaked corn displayed significantly higher mean and peak plasma glucose concentrations than when consuming cracked or ground corn.
Oats respond to processing in a similar way to corn with improvements in starch digestibility increasing as the severity of processing increases. Meyer et al. (1993) found that rolling oats only increased pre-caecal starch digestibility from 83.5 to 85.2%. Householder et al. (1977) observed however, that micronising crimped oats improved its starch digestibility from 48.0% to 62.4%. The pre-caecal oat starch digestibilities reported by these authors vary considerably, even though both used small intestinal cannulae and the same marker to estimate digestibility. The differences between these results may partially be attributed to the use of chromic oxide as an external marker (Haenlein et al., 1966; Owens and Hanson, 1992).
Studies in vitro, using the modification of the method of Bird et al. (1999) described above, also demonstrate the positive effect of grain processing on the enzyme digestibility of starch from triticale, corn and rice. Steam-rolling increased the in vitro digestibility of triticale by 53% while extruding increased the in vitro digestion of corn and rice starch by 368 and 329%, respectively (Figure 2, Richards, 2003).
This improvement in in vitro starch digestibility was detected in vivo. However, in vivo digestibility did not appear to respond to grain processing to the same extent as observed in vitro. When the glycaemic response was used as an indirect indicator of small intestinal starch digestion, horses consuming the steam-rolled triticale, extruded corn and extruded rice diets displayed higher peak glucose responses than when consuming the unprocessed (cracked) triticale, corn and rice diets (all diets were fed at a level of 670 g starch/meal). The differences, however, were not significant (Figure 3; Richards, 2003).
IS GRAIN PROCESSING ENOUGH?
Thus, while it seems grain-processing increases the susceptibility of cereal grain starch to enzyme attack and thus improves digestibility in vitro, it does not appear that in vivo digestibility responds in the same manner. On investigation of the individual glycaemic response curves for horses consuming the steamrolled triticale (Figure 4a), extruded corn (Figure 4b) and extruded rice (Figure 4c) diets, it becomes evident that horses display vastly different responses to the same processed cereal grain. However, while the responses to cereal grain diets were highly variable between horses, horses were consistently ranked in order of response over a range of cereal grain diets, with the horse displaying the highest peak glucose response for one diet generally displaying the highest response for all diets (Richards, 2003). Thus we believe that the major factor affecting the observed glycaemic response is the quantity of starch that is digested in the small intestine. With many of the barriers to starch digestion removed by grain processing, we would expect that the small intestinal starch digestion of steam-rolled triticale, extruded corn and extruded rice would now be extensive in all horses. Instead, consistent between-horse variations suggest that physical and physiological attributes of individual horses are limiting the extent of starch digestion in the small intestine, with a low glycaemic response indicating a limited ability to digest starch.
Figure 2. The quantity of starch digested from triticale, steam-rolled triticale, corn, extruded corn, rice and extruded rice during an in vitro starch digestion assay where grains are incubated in the presence of excess α−amylase and amyloglucosidase for 15 minutes at 39°C (Richards, 2003).
Figure 3. The average peak plasma glucose responses of horses consuming triticale, steam-rolled triticale, corn, extruded corn, rice and extruded rice (Richards, 2003).a)
b)
c)
Figure 4. The glycaemic responses of four different horses to a diet of (a) steam-rolled triticale, (b) extruded corn and (c) extruded rice.
Why can’t horses digest starch effectively in the small intestine?The variation observed between horses may be due to differences in the capacity to absorb glucose from the small intestine (Church and Middleton, 1997; Mair et al., 1991) or differences in eating and chewing behaviours (Meyer et al., 1995). However, there have been several reports that the concentrations of starch digesting enzymes, especially α−amylase, in the equine small intestine are low and vary widely between horses (Alexander and Choudhury, 1958; Comline et al., 1969; Kienzle et al., 1994; Roberts, 1974). Consequently it is likely that capacity to break down starch into maltose, maltotriose and α− dextrin units will be limited by sub-optimal concentrations of α−amylase. In addition, not all α−amylases have equal capacity to digest starch (Anindyawati et al., 1998; MacGregor et al., 2001). Therefore, it is also possible that the activity, or ability of equine α−amylase to degrade starch will limit small intestinal starch digestion.
The activity of equine α−amylase was previously unknown. To examine the ability of equine α− amylase to degrade starch from various cereal grain sources, jejunal fluid was collected from a 9-yearold thoroughbred gelding and the jejunal supernatant, containing 57 U of α−amylase/ml was used in place of bacterial α−amylase in an in vitro starch digestion assay. The method of Bird et al. (1999), which utilises 300 U of α−amylase derived from Bacillus licheniformis and 20 U of AMG derived from Aspergillus niger per grain sample, was modified for the assay. The assay involved the incubation of 100 mg of cereal grain in 5.3 ml of equine jejunal fluid (300 U α−amylase and 5.6 U AMG) and 14.4 U of AMG derived from Aspergillus niger for 15 minutes at 39°C.
It was hypothesised that due to the greater array of protease and glycanase enzymes assumed to be present in equine jejunal fluid, in vitro starch digestion within jejunal fluid would be higher than that observed in the standard in vitro starch digestion assay, which employs only Bacillus licheniformis α−amylase and Aspergillus nigerderived AMG. However, an average of 20.6% less starch was digested in jejunal fluid in comparison to that digested during the standard starch digestion assay (Table 3), suggesting that perhaps the activity of the equine α−amylase is inferior to that of the B. licheniformis α−amylase. Such variation between amylases in ability to degrade starch has been previously reported by Anindyawati et al. (1998) who found that of the three forms of α−amylase produced by the fungi Aspergillus awamori KT- 11, only two had the ability to degrade raw corn starch and of these two, one was more effective than the other. The enzyme activity of α−amylases is possibly related to the number of sub-sites (an area within an active site capable of interacting with one glucose molecule) at each of the enzyme active sites and the affinity of each of these sub-sites for a glucose molecule (MacGregor, 1993; MacGregor et al., 2001).
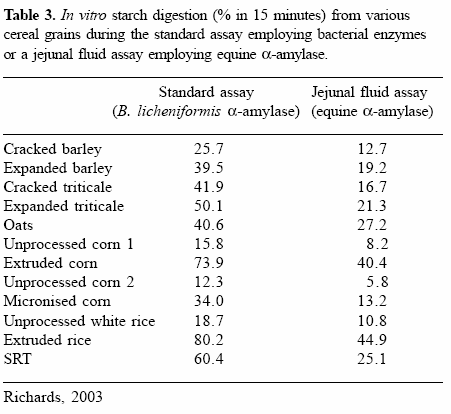
In contrast to α−amylase, Roberts et al. (1974) and Kienzle et al. (1993) reported that activity levels of brush border glycanases are comparable to those observed in humans, pigs and dogs. It therefore appears unlikely that a deficiency of brush border glycanases, including AMG, will limit starch digestion in small intestine of the horse.
Is there a role for dietary enzyme supplementation?With numerous reports that α−amylase concentrations in the equine small intestine are low and highly variable between horses (Alexander et al., 1958; Comline et al., 1969; Kienzle et al., 1994; Roberts, 1974), and the finding that equine α− amylase has an inferior capacity to digest starch in vitro when compared to α−amylase derived from B. licheniformis (Richards, 2003), a deficiency of this small intestinal glycanase or its inability to digest starch efficiently, may be major contributing factors to the variable and often poor ability to digest starch pre-caecally.
A trial was designed to investigate the effect of exogenous α−amylase and/or amyloglucosidase (AMG) when added to a cereal grain diet on the digestion of starch in the equine small intestine. The hypothesis was that the addition of α−amylase or a combination of α−amylase and AMG to diets containing digestible starch would improve equine small intestinal starch digestion. It was also hypothesised that α−amylase is the primary limiting factor to starch digestion in the equine small intestine and that the addition of AMG alone would have a non-significant effect on pre-caecal starch digestion. The design of the experiment was based on the assumption that an improvement in small intestinal starch digestion would be reflected in elevated glycaemic responses.
Three treatment diets and a control diet were used in the experiment. The control grain mix, to which exogenous enzymes were added, consisted of steam-rolled triticale (662 g/kg starch, 120 g/kg nonstarch polysaccharide, 120 g/kg crude protein, 32 g/kg crude fat, 25 g/kg crude fibre, 17.0 MJ/kg, all figures presented on a dry matter basis). Steamrolled triticale had a high in vitro starch digestibility (82% of starch digested in 1 hr) when incubated at 39°C in the presence of excess thermostable α− amylase and AMG using the method of Bird et al. (1999). The control diet consisted of 1.12 kg of steam-rolled triticale/meal, which corresponded to 670 g of starch. Meal sizes were not varied according to body weight.
Treatment 1 was 1.12 kg steam-rolled triticale and 3 ml of heat stable α−amylase derived from Bacillus licheniformis, treatment 2 was 1.12 kg steam-rolled triticale and 1 ml AMG, derived from Aspergillus niger and treatment 3 consisted of 1.12 kg steam-rolled triticale, 1 ml AMG and 3 ml of heat stable α−amylase. All enzymes were diluted to 25 ml with distilled water and sprayed over the steam-rolled triticale meal using a hand held spray nozzle to thoroughly cover the grain. Enzymes were added to the grain meal 10 minutes prior to feeding and horses were fed twice daily at 0700 h and 1730 h.
Twelve horses (four standardbred geldings, four standardbred mares and four thoroughbred geldings), aged 4 to 9 years and weighing 432–512 kg were used in the study. Horses were divided into four groups of three horses. Group A was allocated to the control diet, group B to the α− amylase diet, group C to the AMG diet, and group D were placed on the α−amylase + AMG diet. Horses were allowed one and a half days to acclimatize to the stables and daily routine. The experimental diets were fed from the evening feed of the second day. Blood sampling to measure the glycaemic response took place on the morning of the fifth and eighth days.
The addition of a combination of α−amylase and AMG to the steam-rolled triticale diet significantly elevated peak, average and peak minus basal plasma glucose concentrations above those in horses given the control diet, while the addition of α−amylase alone significantly increased average glucose concentrations (Table 4). Thus, in support of the hypothesis, these findings indicate that dietary supplementation with amylolytic enzymes improves small intestinal starch digestion and are in accordance with Meyer et al. (1993) who observed a 12% improvement in the pre-caecal digestion of cracked corn following the addition of powdered α−amylase.
Table 4. Mean peak glucose concentration, average glucose concentration, peak minus basal glucose concentration, time to peak glucose and slope to peak glucose for the control, α-amylase, amyloglucosidase (AMG) and α-amylase + AMG diets.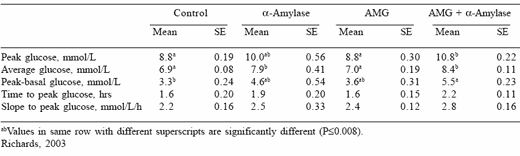
The addition of AMG to the control diet appeared to make no improvement to the digestion of starch in the small intestine, with similar glycaemic responses observed for horses given the control and AMG diets (Table 4). Thus in support of the hypothesis it appears that endogenous concentrations of AMG in the equine small intestine are adequate to break down the products of endogenous α−amylase digestion to glucose. This observation further supports the theory that a deficiency of α−amylase is the major limiting factor to starch digestion in the equine small intestine and is in agreement with Kienzle et al. (1993) and Roberts et al. (1974), who suggest that horses possess naturally high levels of brush border glycanases, including AMG. However, the addition of AMG to a diet also being supplemented with exogenous α−amylase increased the glycaemic response above that observed for the diet being supplemented with exogenous α−amylase only. This indicates that endogenous AMG may not be sufficient to cope with the excess maltose, maltotriose and α−dextrin units produced through the addition of α−amylase to the diet, suggesting that a combination of α−amylase and AMG may be necessary to effectively enhance small intestinal starch digestion.
It was observed during this study that sampling period significantly affected the average glucose response. Horses supplemented with α−amylase had a significantly reduced average glucose response during the second sampling period (day 8) compared to that observed during the first sampling period (day 5, Figure 5a). A significant diet × period interaction was also observed for average (P≤0.02) and peak (P≤0.03) glucose concentration parameters, with the control diet increasing both average and peak glucose responses over time, while the α−amylase diet had a decreasing effect between sampling periods one and two (Figure 5a and 5b). Although these results are difficult to explain, the decline in response to the α−amylase diet over time again suggests that the addition of a combination of α−amylase and AMG to cereal grain diets may be desirable, as endogenous AMG in the small intestine of horses consuming the α−amylase diet appears to become overwhelmed with the surplus maltose, maltotriose and α−dextrin substrate that is likely to be produced through the addition of exogenous α− amylase to the diet.
a)
b)
Figure 5. The significant effect of sampling period and the sampling period × diet interactions for (a) peak plasma glucose concentration and (b) average plasma glucose concentration for the diets α-amylase, amyloglucosidase (AMG), α-amylase + AMG and the control diet in period 1 and period 2.
Benefits of dietary enzyme supplementationFour major benefits may be expected following the dietary supplementation of cereal grain diets with amylolytic enzymes. These are: 1. The feeding efficiency of cereal grains will be improved as starch will be digested and absorbed as glucose in the small intestine in a more energy efficient process than the fermentation of starch in the equine hindgut. 2. The incidence of hindgut starch fermentation and hindgut acidosis will be reduced. 3. The occurrence of diseases associated with hindgut acidosis, such as endotoxemia, metabolic acidosis and laminitis will be reduced. 4. The incidence of adverse behaviours often associated with acid accumulation in the equine hindgut may be reduced.
ConclusionExogenous enzyme supplementation of equine diets containing a digestible source of starch may be used to overcome endogenous enzyme deficiencies in the equine small intestine, thus removing a major barrier to pre-caecal starch digestion in horses. With dietary enzyme supplementation and enhanced small intestinal starch digestion, the feeding of cereal grains to horses will become a more efficient and safer practice than it has been in the past.
ReferencesAlexander, F. and A.K. Chowdhury. 1958. Enzymes in the ileal juice of the horse. Nature 181:190.
Anindyawati, T., R. Melliawati, K. Ito, M. Iizuka and N. Minamiura. 1998. Three different types of α−amylases from Aspergillus awamori KT- 11: Their purifications, properties and specificities. Bioscience, Biotech. & Biochem. 62:1351-1357.
Arnold, F.F., G.D. Potter, J.L. Kreider, G.T. Schelling and W.L. Jenkins. 1981. Carbohydrate digestion in the small and large intestine of the equine. In: Proceedings 7th Equine Nutrition and Physiology Symposium, pp. 19-22, Warrenton, Virginia.
Bird, A.R., W.J. Croom, Jr, Y.K. Fan, B.L. Black, B.W. McBride and I.L. Taylor. 1996. Peptide regulation of intestinal glucose absorption. J. Anim. Sci. 74:2523-2540.
Bird, S.H., J.B. Rowe, M. Choct, S. Stachiw, P. Tyler and R.D. Thompson. 1999. In vitro fermentation of grain and enzymatic digestion of cereal starch. Recent Advances in Anim. Nutr. 12:53-61.
Black, J.L. 1971. A theoretical consideration of the effect of preventing rumen fermentation on the efficiency of utilisation of dietary energy and protein in lambs. Brit. J. Nutr. 25:31-55.
Brand, J.C., P.L. Nicholson, A.W. Thorburn and A.S. Truswell. 1985. Food processing and the glycaemic index. Amer. J. Clinical Nutr. 42:1192- 1196.
Choct, M. 1995. Role of soluble and insoluble fibre in broiler nutrition, Rep. No. CSN 2CM. Chicken Meat Research and Development Council, O’Halloran Hill.
Church, S. and D.J. Middleton. 1997. Transient glucose malabsorption in two horses - fact or artefact? Aust. Vet. J. 75:716-718.
Comline, R.S., L.W. Hall, J.C.D. Hickson, A. Murillo and R.G. Walker. 1969. Pancreatic Secretion in the Horse. J. Physiology 204:10P- 11P.
de Fombelle, A., E. Jacotot, C. Drogoul, T. Bonnefoy and V. Julliand. 1999. Effect of the hay:grain ratio on the digestive physiology and microbial ecosystem in ponies. In: Equine Nutrition and Physiology Society Symposium, Vol. 16, Raleigh, North Carolina, USA.
de Fombelle, A., P. Frumholtz, D. Poillion, C. Drogoul, C. Phillipeau, E. Jacotot and V. Julliand. 2001. Effect of the botanical origin of starch on its prececal digestibility measured with the mobile bag technique. In: Equine Nutrition and Physiology Society Symposium, Vol. 17, pp. 154- 156, Lexington, Kentucky, USA.
Frape, D. 1998. Equine Nutrition and Feeding, 2nd Edition/Ed. MPG Books Ltd, Cornwall.
Gaebe, R.J., D.W. Sanson, I.G. Rush, M.L. Riley, D.L. Hixon and S.I. Paisley. 1998. Effects of extruded corn or grain sorghum on intake, dgestibility, weight gain and carcasses of finishing steers. J. Anim. Sci. 76:2001-2007.
Garner, H.E., J.R. Coffman, A.W. Hahn, D.P. Hutcheson and M.E. Tumbleson. 1975. Equine laminitis of alimentary origin: An experimental model. Amer. J. Vet. Res. 36:441-444.
Garner, H.E., D.P. Hutcheson, J.R. Coffman, A.W. Hahn and C. Salem. 1977. Lactic aidosis: a factor associated with equine laminitis. J. Anim. Sci. 45:1037-1041.
Garner, H.E., J.N. Moore, J.H. Johnson, L. Clarke, J.F. Amend, L.G. Tritschler, J.R. Coffman, R.F. Sprouse, D.P. Hutcheson and C.A. Salem. 1978. Changes in caecal flora associated with the onset of laminitis. Equine Vet. J. 10:249-252.
Godfrey, S.I., M.D. Boyce, J.B. Rowe and E.J. Speijers. 1992. Changes within the digestive tract of sheep following engorgement with barley. Aust. J. Agri. Res. 44:1093-1101.
Gray, G.M. 1992. Starch digestion and absorption in nonruminants. J. Nutr. 122:172-177.
Haenlein, G.F.W., R.C. Smith and Y.M. Yoon. 1966. Determination of the faecal excretion rate of horses with chromic oxide. J. of Anim. Sci. 25:1091-1095.
Halnan, E.T. and F.H. Garner. 1953. The principles and practice of feeding farm animals. Fourth edition. Longmans, Green and Co., London.
Hinkle, D.K., G.D. Potter and J.L. Kreider. 1983. Starch digestion in different segments of the digestive tract of ponies fed varying levels of corn. In: Proceedings of the 8th Equine Nutrition and Physiology Symposium, pp. 227-230, Kentucky.
Hoekstra, K.E., K. Newman, M.A.P. Kennedy and J.D. Pagan. 1999. Effect of corn processing on glycaemic responses in horses. In: Equine Nutrition and Physiology Symposium, Vol. 16, pp. 169-173, Lexington, Kentucky.
Holm, J., B. Hagander, I.E.A. Bjork and I. Lundquist. 1989. The effect of various thermal processes on the glycaemic response to whole grain wheat products in humans and rats. J. Nutr. 119:1631-1638.
Holt, S.H.A. and J. Brand Miller. 1994. Particle size, satiety and the glycaemic response. Europ. J. Clinical Nutr. 48:496-502.
Householder, D.D., G.D. Potter and R.E. Lichtenwalner. 1977. Nutrient utilisation in different segments of the equine digestive tract. In: Proceedings 5th Equine Nutrition and Physiology Symposium, pp. 44-45.
Hubbard, R.E. and R.M. Hansen. 1976. Diets of wild horses, cattle and mule deer in the Piceance Basin, Colorado. J. Range Management 29:389- 392.
Huntington, G.B. 1997. Starch utilisation by ruminants: from basics to the bunk. J. Anim. Sci. 75:852-867.
Johnson, K.G., J. Tyrrel, J.B. Rowe and D.W. Pethick. 1998. Behavioural changes in stabled horses given non-therapeutic levels of virginiamycin. Equine Vet. J. 30:139-143.
Joy, M.T., E.J. DePeters, J.G. Fadel and R.A. Zinn. 1997. Effects of corn processing on the site and extent of digestion in lactating cows. J. Dairy Sci. 80:2087-2097.
Kienzle, E. 1994. Small intestinal digestion of starch in the horse. Revue Med. Vet. 145:199-204.
Kienzle, E. and S. Radicke. 1993. Effect of diet on maltase, sucrase and lactase in the small intestinal mucosa of the horse. J. Anim. Physiology & Anim. Nutr. 70:97-103.
Kienzle, E., S. Radicke, E. Landes, D. Kleffken, M. Illenseer and H. Meyer. 1994. Activity of amylase in the gastrointestinal tract of the horse. J. Anim. Physiology & Anim. Nutr. 72:234-241.
Kienzle, E., J. Pohlenz and S. Radicke. 1997. Morphology of starch digestion in the horse. J. Vet. Med. A 44:207-221.
Knowlton, K.F., B.P. Glenn and R.A. Erdman. 1998. Performance, ruminal fermentation and site of starch digestion in early lactation cows fed corn grain harvested and processed differently. J. Dairy Sci. 81:1972-1984.
Kotarski, S.F., R.D. Waniska and K.K. Thurn. 1992. Starch hydrolysis by the ruminal microflora. J. Nutr. 122:178-190.
Krueger, A.S., D.A. Kinden, H.E. Garner and R.F. Sprouse. 1986. Ultrastructural study of the equine caecum during onset of laminitis. Amer. J. of Vet. Res. 47:1804-1812.
Leek, B.F. 1993. Digestion in the ruminant stomach. In: Duke’s Physiology of Domestic Animals (M.J. Swenson and W.O. Reece, eds.), Comstock Publishing Associates, London, pp. 387-416.
MacGregor, E.A. 1993. Relationships between structure and activity in the α−amylase family of starch metabolising enzymes. Starch 45:232-237.
MacGregor, E.A., S. Janecek and B. Svensson. 2001. Relationship of sequence and structure to specificity in the α−amylase family of enzymes. Biochimica et Biophysica Acta 1546:1-20.
Mair, T.S., M.H. Hillyer, F.G.R. Taylor and G.R. Pearson. 1991. Small intestinal malabsorption in the horse: An assessment of the specificity of the oral glucose tolerance test. Equine Vet. J. 23:344- 346.
McAllister, T.A., R.C. Phillippe, L.M. Rode and K.J. Cheng. 1993. Effect of the protein matrix on the digestion of cereal grains by ruminal microorganisms. J. Anim. Sci. 71:205-212.
Meyer, H., S. Radicke, E. Kienzle, S. Wilke and D. Kleffken. 1993. Investigations on preileal digestion of oats, corn and barley starch in relation to grain processing. In: 13th Equine Nutrition and Physiology Symposium, pp. 92-97, Florida.
Meyer, H., S. Radicke, E. Kienzle, S. Wilke, D. Kleffken and M. Illenseer. 1995. Investigations on preileal digestion of starch from grain, potato and manioc in horses. J. Vet. Med. A 42:371-381.
Morrison, F.B. 1954. Feeds and Feeding. A handbook for the student and stockman, Twenty- First Edition/Ed. The Morrison Publishing Company, New York.
Muir, J.G., A. Birkett, I. Brown, G. Jones and K. O’Dea. 1995. Food processing and maize variety affects amounts of starch escaping digestion in the small intestine. Amer. J. Clinical Nutr. 61:82- 89.
Owens, F.N. and C.F. Hanson. 1992. Symposium: external and internal markers - external and internal markers for appraising site and extent of digestion in ruminants. J. Dairy Sci. 75:2605-2617.
Pollitt, C. 2001. Equine Laminitis, Rural Industries Research and Development Corporation, Canberra.
Radicke, S., E. Kienzle and H. Meyer. 1991. Preileal apparent digestibility of oats and cornstarch and consequences for cecal metabolism. In: Proceedings 12th Equine Nutrition and Physiology Symposium, pp. 43 - 48, Calgary.
Radostits, O.M., C.C. Gay, D.C. Blood and K.W. Hinchcliff. 2000. Veterinary Medicine: A Textbook of the Diseases of Cattle, Sheep, Pigs, Goats and Horses, 9th Edition, W.B. Saunders Company Ltd, London.
Richards, N. 2003. Starch digestion in the equine small intestine. Dissertation, University of New England, Armidale.
Roberts, M.C. 1974. Amylase activity in the small intestine of the horse. Res. in Vet. Sci. (UK) 17:400-401.
Roberts, M.C., F.W.G. Hill and D.E. Kidder. 1974. The development and distribution of small intestinal disaccharidases in the horse. Res. Vet. Sci. 17:42-48.
Rooney, L.W. and R.L. Pflugfelder. 1986. Factors affecting starch digestibility with special emphasis on sorghum and corn. J. Anim. Sci. 63:1607-1623.
Rowe, J.B., D.W. Pethick and K.G. Johnson. 1995. Controlling acidosis in the equine hindgut. Recent Advances in Anim. Nutr. in Australia 10:136-142.
Rowe, J.B., M. Choct and D.W. Pethick. 1999. Processing cereal grains for animal feeding. Aust. J. Agric. Res. 50:721-736.
Rowe, J.B., M.J. Lees and D.W. Pethick. 1994. Prevention of acidosis and laminitis associated with grain feeding in horses. J. Nutr. 124:2742S- 2744S.
Russell, J.B. and D.W. Wilson. 1995. Why are ruminal cellulolytic bacteria unable to digest cellulose at low pH? J. Dairy Sci. 79:1503-1509.
Salter, R.E., and R.J. Hudson. 1979. Feeding ecology of feral horses in Western Alberta. J. Range Management 32:221-225.
Snow, P. and K. O’Dea. 1981. Factors affecting the rate of hydrolysis of starch in food. Amer. J. Clinical Nutr. 34:2721-2727.
Theurer, C.B. 1986. Grain processing effects on starch utilisation by ruminants. J. Anim. Sci. 63:1649-1662.
Theurer, C.B., O. Lozano, A. Alio, A. Delgado- Elorduy, M. Sadik, J.T. Huber and R.A. Zinn. 1999. Steam processed corn and sorghum grain flaked at different densities alter ruminal, small intestinal and total tract digestibility of starch by steers. J. Anim. Sci. 77:2824-2831.
Thorens, B. 1993. Facilitated glucose transporters in epithelial cells. Ann. Rev. Physiology 55:591- 608.
Thorne, M.J., L.U. Thompson and D.J.A. Lenkins. 1983. Factors affecting starch digestibility and the glycaemic response with special reference to legumes. The Amer. J. Clinical Nutr. 38:481-488.
Waring, G.H. 1983. Horse Behaviour; The Behavioural Traits and Adaptations of Domestic and Wild Horses, Including Ponies. Noyes Publications, Park Ridge.
Willard, J.G., J.C. Willard, S.A. Wolfram and J.P. Baker. 1977. Effect of diet on caecal pH and feeding behaviour of horses. J. Anim. Sci. 45:87- 93.
Zinn, R.A. 1993. Influence of processing on the comparative value of barley for feedlot cattle. J. Anim. Sci. 71:3-10.
Zinn, R.A., C.F. Adam and M.S. Tamayo. 1995. Interaction of feed intake level on comparative ruminal and total tract digestion of dry-rolled and steam flaked corn. J. Anim. Sci. 73:1239-1245.