Overview
Horses over 20 years of age constitute about 15% of the equine population and many remain actively involved in equestrian sports and reproductive capacities as stallions and brood mares (Malinowski et al., 1997). However, advancing age in horses, as with other species, is eventually associated with a decline in body condition and muscle tone as well as an increase in susceptibility to infections (Atwill et al., 1996; Austin et al., 1995; Ralston et al., 1988). This increased susceptibility to infectious diseases is due to an overall decline in immune function that results from alterations of many different elements of the immune system. While these changes have been extensively documented in humans and mouse models, little research has been done in the equine system. Vaccination remains the most cost-effective measure for preventing and reducing the severity of infectious diseases in the equine population. Little is known, however, regarding the effectiveness of vaccinations in the geriatric equine population. Here we have examined the vaccine response of two populations of horses to determine the effect of vaccination frequency on their ability to respond. While younger horses responded well to vaccination, clear differences were seen in those horses >18 years of age.
The effect of ageing on innate immunityIn order to elucidate the complex events involved in immunosenescence we must first consider how the immune system responds to infection. All animals, upon parturition, have a natural or innate immunity that acts as a limited but rapid response to infection and which does not require prior exposure to the pathogen (Benjamini et al., 1996; Parham, 2000). These aspects of the immune response include the chemotactic attraction of phagocytic and cytotoxic cells to the site of the infection and the subsequent production of soluble mediators. Macrophages and neutrophils are the key cells involved in the phagocytosis of invading organisms. Macrophages are also the source of various chemokines that recruit other phagocytic cells, including neutrophils, to the infected area. Chemokines also act on local blood vessels causing vasodilation, increased vascular permeability, and the eventual exudation of plasma and cells to the site of ongoing infections (Parham, 2000).
Other soluble mediators involved in this phase of the innate immune response are mannose-binding protein, serum amyloid protein, and complement proteins that bind to prokaryotic carbohydrate structures and facilitate their uptake by the phagocytic cells (Castle, 2000b). Natural killer (NK) cells are cytotoxic effector cells that play a central role in the innate immune response to tumors and virus-infected cells (Solana and Mariani, 2000). The non-specific cytotoxic activity of NK cells can be further enhanced by the cytokines interleukin (IL)-2 and interferon-gamma (IFNγ). These so-called lymphokine-activated killer (LAK) cells are capable of lysing tumor cells resistant to NK-mediated lysis. NK cells are also the source of IFNγ and as such can augment macrophage microbicidal and tumorcidal activity (Solana and Mariani, 2000).
To date, most research has not shown a significant alteration in innate immune function between young and old individuals, though some subtle alterations may be present. Thus there is a general decline in phagocytic capabilities of human neutrophils (Ginaldi et al., 1999a). Likewise there is also a slight increase in the susceptibility of human neutrophils to apoptosis with advanced age (Fulop et al., 1997). Whether these alterations contribute to increased susceptibility to infection in the elderly remains unknown. By contrast, the phagocytic and bactericidal activities of pulmonary macrophages and neutrophils from young and aged mice appear comparable (Wyde et al., 1989). Alterations in macrophage cytokine production could also play a role in the increased susceptibility of elderly individuals to endotoxins (Sunderkotter et al., 1997). This impairment of cytokine production could have an important impact on the induction of specific immune responses, as discussed below. Similarly, ageing is associated with a decrease in the cytolytic activity of NK cells in circulation, though this appears to be offset by an increase in the total number of these cells in the circulation (Castle, 2000b; Malaguarnera et al., 2001; Miller, 1991; Solana and Mariani, 2000). This functional decline in NK activity may be the result of decreased responsiveness to IFNγ and IL-2 (Castle, 2000b; Malaguarnera et al., 2001; Miller, 1991).
A few studies have assessed the effect of ageing on innate immune responses of horses. Overall LAK cell activity in old, Standardbred mares appears equivalent to that seen in younger mares (Horohov et al., 1999), though the possibility of there being fewer cells in circulation was not assessed. In another study there were no differences in the circulating numbers of monocytes or granulocytes between young and aged horses (Guirnalda et al., 2001); nor was there an effect of ageing on the respiratory burst activity of equine neutrophils (Guirnalda et al., 2001). These results are thus in agreement with most other studies indicating that ageing has little effect on the innate immune responses (Franceschi et al., 2000).
The effect of ageing on adaptive immune responsesAcquired or adaptive immunity involves the induction of cellular and humoral immune responses directed against a specific antigen. Adaptive immunity is also characterized by the development of cellular memory that provides a more rapid secondary response upon reexposure to the antigen. Acquired immunity involves two major cell types: B-lymphocytes and T-lymphocytes.
B cells are responsible for the production and secretion of antibodies. While each individual B cell has its own unique antigen-specificity, the process of gene rearrangement during B cell ontogeny leads to the
development of B-cells with specificity for 107 different antigens. The product of pluripotential hematopoietic stem cells, the B-cell matures in the bone marrow and then migrates to secondary lymphoid tissues. Upon exposure to its antigen, the B-cell can proliferate and differentiate into an antibody secreting plasma cell, or it may become a memory B-cell. Activation of a memory cell by re-exposure to a specific antigen leads to a rapid antibody response.
Ageing markedly reduces antibody production in both humans and mice (Klinman and Kline, 1997). Though the total number of B-cells in circulation does not appear to be reduced in aged mice, there is a marked decrease in B-cell ontogeny and increased signs of apoptosis (Klinman and Kline, 1997). There also appears to be decreased migration of plasma cells to effector sites in old animals (Schmucker et al., 2001). This latter effect could have significant impact on the development of local immune responses at mucosal surfaces. Advanced age is also associated with an overall shift to antibodies of lower affinity for their specific antigen (Zheng et al., 1997). In young animals, the maturation of the antibody response is associated with an increased affinity of antibodies for their specific antigen. This increased affinity is the result of somatic mutations in the variable region of the immunoglobulin genes. The reduced affinity seen in the elderly can be attributed to the agerelated reduction in germinal center reactions and immunoglobulin hypermutation (Yang et al., 1996).
Lower affinity and reduced B-cell responsiveness to antigen could account for the reduced efficacy of vaccinations in older individuals (Remarque et al., 1999). While current vaccine formulations are highly efficacious in young, healthy recipients (Subbarao, 1999), their failure rate in the elderly may be as high as 50% (Gravenstein et al., 1989). Altered responses, particularly in terms of immunoglobulin isotypic responses, are also seen in elderly recipients (Remarque et al., 1999). Thus elderly patients produce antibodies of a different isotype compared to younger vaccinates (El-Madhun et al., 1999; Remarque et al., 1999; Remarque et al., 1993). Similar results have been obtained in rodent models (Higgins et al., 1996).
Ageing in horses is likewise associated with reduced antibody responses to vaccination (Goto et al., 1993; Horohov et al., 1999). In one study, the proportion of horses producing hemagglutination-inhibition (HI) antibodies following equine influenza virus vaccination decreased with advancing age such that no HI antibody responses were seen in horses over 9 years of age (Goto et al., 1993). When antibody responses were measured by ELISA, a more sensitive measure of total antibody responsiveness, a significant decrease in influenza virusspecific antibody levels was seen in horses greater than 20 years of age following vaccination, as compared to a younger cohort (Horohov et al., 1999). The mechanism of this decline is unknown but appears to be secondary to alterations in T-cell function (Horohov et al., 1999).
While alterations in B-cell ontogeny and function can contribute to decreased humoral responses in the elderly, it is now believed that the dramatic effects of ageing on immune competence are the results of deterioration in T-cell function (Pawelec et al., 2000). T-cells are produced primarily in the thymus as the result of the emigration of T-cell precursors from the bone marrow to this organ. Thymic development involves both the generation and the so-called ‘education’ of T-cells to recognize specific antigen in the context of self major histocompatability complex (MHC) antigens. Thymic selection gives rise to the two major subsets of T-cells, CD4+ helper T-cells and the CD8+ cytotoxic T-cells. The CD8+ T-cell recognizes its foreign antigen in association with MHC Class I antigens that are present on the surface of all nucleated cells. These cytotoxic effector cells play a primary role in the immune response to viruses and tumors, lysing their target cells via a variety of mechanisms (Kajino et al., 1998). The CD4+ T-cells recognize foreign antigens presented in the context of MHC Class II antigens found on macrophages and other antigen presenting cells. The CD4+ T-cell population can be further subdivided into T helper (Th) subsets based on the pattern of cytokines they produce. Th1 cells produce IL-2 and IFNγ, and Th2 cells produce IL-4, IL-5, and IL-13. The significance of this segregation of cytokine production is that Th1 cytokines are associated with immune responses to intracellular pathogens, whereas the Th2 response is directed against extracellular agents (Constant and Bottomly, 1997). Therefore, immunity to viruses and intracellular parasites is mediated by Th1 cells, whereas protection against intestinal helminths involves Th2 responses. Though initially described in murine models, similar patterns of Th subsets have been described in other species, including the horse (Horohov, 2000).
One of the most consistent changes noted in immune competency with advancing age is the decrease in the number of naive T-cells with a concomitant increase in T-cells with an activated/memory phenotype (Ginaldi et al., 1999b). While it has long been established that with age there is a significant involution of the thymus and a resulting decline in naïve T-cell production (Ginaldi et al., 1999b; Kurashima et al., 1995), increased apoptosis and lower responsiveness of aged lymphoid cells to activation and proliferation signals also contribute to this process (Ginaldi et al., 2000). Thus T lymphocytes derived from elderly humans are more susceptible to activation-induced cell death than T-cells from young individuals (Phelouzat et al., 1997). While this increase in apoptosis could be the result of increased FAS antigen (CD95) expression on the T-cell surface (Potestio et al., 1998), alterations in normal signaling pathways via other receptors might also contribute to this effect (Quadri et al., 1996; Saini and Sei, 1993; Whisler et al., 1996a). In addition to an increased susceptibility to apoptosis, T-cells from aged individuals also exhibit an impaired proliferative capacity (Ginaldi et al., 1999b). This reduced proliferative capacity is the result of the diminished production of IL-2 by aged T-cells (Ginaldi et al., 1999b; Whisler et al., 1996a). The reduction in IL-2 production is itself associated with the reduced activation of various intracellular signaling pathways (Hutter et al., 2000; Liu et al., 1997; Ponnappan, 1998; Whisler et al., 1996b), particularly those initiated by the T-cell receptor/CD3 complex (Fernandez-Gutierrez et al., 1999; Miller et al., 1997; Whisler et al., 1997). The central role IL-2 plays in age-associated alterations in T-cell function is evident in those studies where supplementation with this cytokine could restore immune responsiveness both in vitro and in vivo (Fagiolo et al., 1997; Lio et al., 2000).
Ageing in horses is associated with similar changes in the thymus and the circulating T-cell compartment (Lunn et al., 1993; Perryman et al., 1988). When compared to their neonatal counterparts, MHC II expression is increased on the T-lymphocytes of adult horses (Lunn et al., 1993). This is consistent with a shift towards a larger memory T-cell population with age as seen in other species. Ageing in equids is also associated with a decreased proliferative response of their T-cells to in vitro stimulation with mitogens (Horohov et al., 2002; Horohov et al., 1999). Though there was no difference in the overall expression of the IL-2 receptor on mitogen-stimulated T-cells from young and aged ponies, supplementation of these cultures with recombinant IL-2 failed to restore the proliferative response of the older cells (Horohov et al., 2002). These results indicate that alterations in IL-2 production alone may not play a central role in age-associated immune deviation in the horse.
While the decreased proliferative responses of T lymphocytes from elderly humans and mice is correlated with decreased release of IL-2, the expression of other cytokine genes and their receptors may also be affected (Rink et al., 1998). Indeed many of the problems associated with an ageing immune response may be due to age-dependent alterations in cytokine gene expression by CD4+ T-cells (Bernstein et al., 1998; Candore et al., 1993; Rink et al., 1998). While the pattern of these changes is complex, ageing appears to be generally associated with a decline in Th1 cytokine expression and a concomitant increase in susceptibility to those infections best controlled by this type of response (Albright and Albright, 1994; Lio et al., 2000). This increased susceptibility to infection is compounded by ageing-associated alterations in CD8+ T-cell function. As age increases, there is a resultant decrease in the cytotoxic activity of virus-specific CD8+ cells in humans (Mbawuike et al., 1993). This decreased cytotoxicity is associated with a decline in perforin gene expression (Rukavina et al., 1998). A similar decline in cytotoxic activity and IFNγ production is seen in aged mice and is associated with increased susceptibility to viral infection (Mbawuike et al., 1996).
The central thesis of this review has been a focus on the changes that occur in the immune responses of older individuals. While it is clear that current vaccination strategies may have to be modified to address the unique needs of the older human (Subbarao, 1999) and equine populations, an important point to consider is the effect of other health conditions on the immune system. The effect of various stressors on immune responses in both humans (Kiecolt-Glaser et al., 1996; Vedhara et al., 1999) and horses is also well recognized (Bueno et al., 1999; Folsom et al., 2001). Dietary and nutritional status are widely viewed as important factors affecting immune responsiveness of the aged population (Castle, 2000a; Lipschitz, 1987; Mazari and Lesourd, 1998; Mocchegiani et al., 2000). Lastly, prior immune exposure is also a critical element in this process. All of these factors must be considered when vaccinating both aged and young horses.
Immune response of aged horses to equine influenza vaccinationTo assess the effectiveness of vaccination on aged horses, we vaccinated two populations of privately-owned, mixed aged horses. The first group of 36 horses, of which 9 were >20 years of age, was maintained as an ‘open’ herd at a public facility and had been vaccinated twice annually for equine influenza using commercially available inactivated vaccines for the past several years. A second group of 24 horses of which 10 were >20 years of age were maintained in a ‘closed’ herd at a private facility and were not routinely vaccinated for equine influenza virus prior to inclusion in this study. Each group was of mixed sex and breed. The horses were kept in stalls during the week and put out to pasture on weekends. While stalled they were all fed a pelleted ration and sweet feed (Purina Stable Star, St Louis, MO) while on this study.
Both populations of horses were vaccinated with a deep intramuscular injection on the right side of the neck into the serratus cervicis with 1.0 mL of a commercially-available, formalin-inactivated equine influenza virus vaccine (Fluvac+, Fort Dodge Laboratories Inc., Fort Dodge, IA) that contained the A/Equine/Kentucky/91 strain of influenza virus. A second dose of the same vaccine was administered two
weeks after the first. No adverse effect of vaccination was noted in either group.
Blood was collected aseptically into vacutainer tubes (Becton Dickinson & Co., Franklin, NJ) via jugular venipuncture prior to vaccination and at monthly intervals after vaccination. The blood was clotted and
subsequently centrifuged for 10 minutes at 1000 rpm to separate the serum. The serum was then withdrawn using a slow pipetting technique and stored in 2 mL micro tubes (Sarstedt, Numbrecht, Germany) at -20°C until assayed.
Equine influenza virus-specific antibody in the sera was quantified using an ELISA (Lunn et al., 1999). Replicate wells of 96-well plates were coated with equine influenza virus antigen (A/Equine/Kentucky/91; 2.5mg of protein /well). The plates were washed and subsequently blocked with 2% fish gelatin (Sigma, St. Louis, MO) in PBS. A standard curve was prepared on each plate using serial dilutions of an equine reference serum known to contain high titer antibody to equine influenza virus. Test serum samples from the vaccinated horses, initially diluted 1/100 and then two-fold to a final dilution of 1/400, were added to duplicate wells. The plates were incubated for 60 minutes at 37°C. Following incubation, the plates were washed thrice with an ELISA wash consisting of PBS, Tween-20, and deionized water. An HRP-conjugated goat antiequine IgG antibody (Bethyl Laboratories, Montgomery, TX) was added to each well. The plates were incubated for 60 minutes and washed with the ELISA wash. A TMB1-component (3,3′,5,5′-tetramethylbenzidine) peroxidase-substrate solution (75 mL per well) (Kirkegaard & Perry Laboratories, Gaithersburg, MD) was added to each well. The reaction was stopped with a 1% HCL solution (Kirkegaard & Perry Laboratories, Gaithersburg, MD) and the plates read at 450 nm using the MRX microplate reader (Dynex Technologies, Chantilly, VA). The results generated by the reference curve allowed sample results to be expressed in ELISA units where the reference sample was calibrated at 1000 EU/mL.
PRIOR VACCINATION OF THE AGED HORSES RESULTED IN BETTER RESPONSES TO THE VACCINEPost-vaccination influenza virus-specific antibody levels were significantly elevated at 1 and 3 months in both the young and old horses of the regularly vaccinated, ‘open’ herd. The antibody levels returned to prevaccination levels by 5 months after vaccination (Figure 1). Though not significantly different, the anti-influenza antibody responses of the older horses tended to be higher than those of the younger horses in the ‘open’ herd. By contrast, the younger horses in the infrequently vaccinated, ‘closed’ herd had significantly higher (P<0.01; RM-ANOVA) antibody levels after vaccination compared to the older horses at the same facility (Figure 2). In general, the older horses in this group failed to respond to the vaccination.
The horses in the ‘open’ herd had significantly higher pre-vaccination antibody levels to equine influenza virus compared to those of the ‘closed’ herd. This was true for both the young and old animals and likely reflected prior exposure to this virus either through vaccination or infection. In an ‘open’ facility there is regular movement of horses into and out of the facility. Thus the resident horses could be exposed to equine influenza virus and frequent vaccination was performed to reduce the risk of infection. By contrast, the second facility housed a closed herd of horses to which no new horses were added over the past several years. Hence it was not deemed necessary to routinely vaccinate these horses against equine influenza virus. Indeed records indicate that they had not been vaccinated against this virus for at least three years.
Figure 1. Antibody responses to equine influenza vaccination in frequently vaccinated horses.
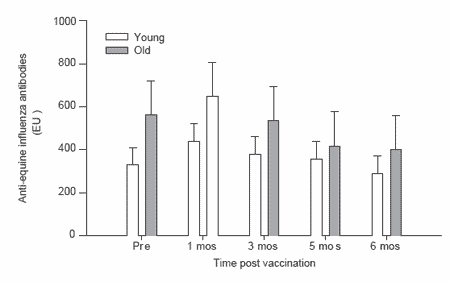
Figure 2. Antibody responses to equine influenza vaccination in horses not recently vaccinated.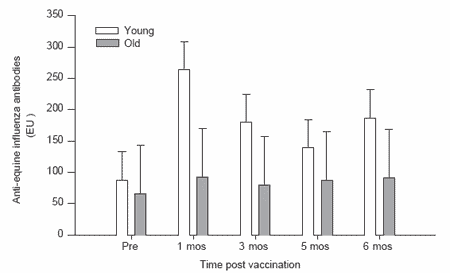
While the younger (<20 years) horses at both facilities exhibited similarities in their responsiveness to equine influenza vaccination, this was not true for the older horses. Variations in vaccine responses of the aged may be most dependent upon individual genetic variations (Bernstein et al., 1999), health status (Carson et al., 2000; Gross et al., 1989; Mysliwska et al., 1998), diet and nutritional status (Castle, 2000a; Lipschitz, 1987; Mazari and Lesourd, 1998; Mocchegiani et al., 2000), and other external factors (Iorio et al., 1999). Similar considerations apply when evaluating the effect of ageing on the ability of horses to respond to vaccination. The adverse effect of parasitism on vaccine responses in the horse has been recently reported (Edmonds et al., 2001). While adult horses tend to have lower levels of parasitism than younger animals, aged individuals may have elevated parasite burdens (Bucknell et al., 1995; Lind et al., 1999; Proudman et al., 1997). While the parasite status of the horses in the current study was not assessed, both facilities utilized similar parasite control programs to reduce parasite burdens. Likewise, both populations of horses were fed similar diets to minimize these effects. Health checks of both populations failed to uncover any other significant differences between the two groups. Thus the major difference between the two groups was their prior vaccination history. As such, our results indicate that those aged horses undergoing routine vaccination responded better in terms of overall antibody response to the vaccine. This is in contrast to the notion that repeated exposure to antigen, particularly in the elderly, may lead to a state of unresponsiveness due to ‘clonal exhaustion’ of the antigen-specific lymphocytes (Solana and Pawelec, 1998). Instead, it appears that routine vaccination of these older horses enhanced their ability to respond to the vaccine. It is not known whether this affected their ability to respond to other vaccines, as well.
Summary and conclusionsThough vaccine manufacturers make no specific recommendations regarding the vaccination of older horses and ponies, the similarities in age-induced immunological changes between humans and equines suggests that similar vaccination recommendations should be followed. The need for vaccination of the older horse will depend, of course, upon the relative risk of exposure for the individual horse. As noted here, routine vaccination may prove more effective in the older horse. In general, annual vaccinations against equine influenza virus infection, tetanus, rabies and encephalomyelitis viruses are warranted. Little is known regarding the use of immunostimulatory or other supplements in the aged horse. While the potential to boost immune responsiveness in these animals is attractive, the potential risk for adverse reactions with such treatments argues against their use until clear benefits have been shown in properly controlled studies. More work, in general, is needed to better understand the immunological needs of this population.
ReferencesAlbright, J.W. and J.F. Albright. 1994. Ageing alters the competence of the immune system to control parasitic infection. Immunol. Lett. 40(3):279-85.
Atwill, E.R., H.O. Mohammed, J.W. Lopez, C.E. McCulloch and E.J. Dubovi. 1996. Cross-sectional evaluation of environmental, host and management factors associated with risk of seropositivity to Ehrlichia risticii in horses of New York state. Am. J. Vet. Res. 57(3):278-85.
Austin, S.M., J.H. Foreman and L.I. Hungerford. 1995. Case-control study of risk factors for development of pleuropneumonia in horses. J. Am. Vet. Med. Assoc. 1. 207(3):325-8.
Benjamini, E., G. Sunshine and S. Leskowitz. 1996. Immunology: A Short Course. 3rd ed., Wiley-Liss, Inc., New York, USA.
Bernstein, E., D. Kaye, E. Abrutyn, P. Gross, M. Dorfman and D.M. Murasko. 1999. Immune response to influenza vaccination in a large healthy elderly population. Vaccine 17(1):82-94.
Bernstein, E.D., E.M. Gardner, E. Abrutyn, P. Gross and D.M. Murasko. 1998. Cytokine production after influenza vaccination in a healthy elderly population. Vaccine 16(18):1722-31.
Bucknell, D.G., R.B. Gasser and I. Beveridge. 1995. The prevalence and epidemiology of gastrointestinal parasites of horses in Victoria, Australia. Int. J. Parasitol. 25(6):711-24.
Bueno, A.C., T.L. Seahorn, J. Cornick-Seahorn, D.W. Horohov and R.M. Moore. 1999. Plasma and urine nitric oxide concentrations in horses given below a low dose of endotoxin. Am. J. Vet. Res. 60(8):969-
76.
Candore, G., G. Di Lorenzo, M. Melluso, D. Cigna, A.T. Colucci, M.A. Modica and C. Caruso. 1993. Gamma-Interferon, interleukin-4 and interleukin-6 in vitro production in old subjects. Autoimmunity 16(4):275-80.
Carson, P.J., K.L. Nichol, J. O’Brien, P. Hilo and E.N. Janoff. 2000. Immune function and vaccine responses in healthy advanced elderly patients. Arch. Intern. Med. 160(13):2017-2024.
Castle, S.C. 2000a. Impact of age-related immune dysfunction on risk of infections. Z. Gerontol. Geriatr. 33(5):341-349.
Castle, S.C. 2000b. Clinical relevance of age-related immune dysfunction. Clin. Infect. Dis. 31(2):578-585.
Constant, S.L. and K. Bottomly. 1997. Induction of TH1 and TH2 CD4+ T cell responses: the alternative approaches. Annu. Rev. Immunol. 15:297-332.
Edmonds, J.D., D.W. Horohov, M.R. Chapmat, S.S. Pourciau, K. Antoku, K. Snedden and T.R. Klei. 2001. Altered immune responses to a heterologous protein in ponies with heavy gastrointestinal parasite burdens. Equine Vet. J. 33(7):658-663.
El-Madhun, A.S., R.J. Cox and L.R. Haaheim. 1999. The effect of age and natural priming on the IgG and IgA subclass responses after parenteral influenza vaccination. J. Infect. Dis. 180 (4):1356-1360.
Fagiolo, U., M.C. Bordin, R. Biselli, R. D’Amelio, R. Zamarchi and A. Amadori. 1997. Effect of rIL-2 treatment on anti-tetanus toxoid response in the elderly. Mech. Ageing Dev. 93(1-3):205-214.
Fernandez-Gutierrez, B., J.A. Jover, S. De Miguel, C. Hernandez-Garcia, M.T. Vidan, J.M. Ribera, A. Banares and J.A. Serra. 1999. Early lymphocyte activation in elderly humans: impaired T and Tdependent
B cell responses. Exp. Gerontol. 34(2):217-229.
Folsom, R.W., M.A. Littlefield-Chabaud, D.D. French, S.S. Pourciau, L. Mistric and D.W. Horohov. 2001. Exercise alters the immune response to equine influenza virus and increases susceptibility to infection.
Equine Vet. J. 33(7):664-669.
Franceschi, C., M. Bonafe and S. Valensin. 2000. Human immunosenescence: the prevailing of innate immunity, the failing of clonotypic immunity and the filling of immunological space. Vaccine 18(16):1717-1720.
Fulop, T., Jr., C. Fouquet, P. Allaire, N. Perrin, G. Lacombe, J. Stankova, M. Rola-Pleszczynski, D. Gagne, J.R. Wagner, A. Khalil and G. Dupuis. 1997. Changes in apoptosis of human polymorphonuclear
granulocytes with aging. Mech. Ageing Dev. 96(1-3):15-34.
Ginaldi, L., M. De Martinis, A. D’Ostilio, L. Marini, M.F. Loreto and D. Quaglino. 1999a. The immune system in the elderly: III. Innate immunity. Immunol. Res. 20(2):117-126.
Ginaldi, L., M. De Martinis, A. D’Ostilio, L. Marini, M.F. Loreto, V. Martorelli and D. Quaglino. 1999b. The immune system in the elderly: II. Specific cellular immunity. Immunol. Res. 20(2):109-115.
Ginaldi, L., M. De Martinis, A. D’Ostilio, L. Marini, M.F. Loreto, M.P. Corsi and D. Quaglino. 2000. Cell proliferation and apoptosis in the immune system in the elderly. Immunol. Res. 21(1):31-8.
Goto, H., Y. Yamamoto, C. Ohta, T. Shirahata, T. Higuchi and H. Ohishi. 1993. Antibody responses of Japanese horses to influenza viruses in the past few years. J. Vet. Med. Sci. 55(1):33-7.
Gravenstein, S., E.H. Duthie, B.A. Miller, E. Roecker, P. Drinka, K. Prathipati and W.B. Ershler. 1989. Augmentation of influenza antibody response in elderly men by thymosin alpha one. A double-blind
placebo-controlled clinical study. J. Am. Geriatr. Soc. 37(1):1-8.
Gross, P.A., G.V. Quinnan, Jr., M.E. Weksler, U. Setia and R.G. Douglas, Jr. 1989. Relation of chronic disease and immune response to influenza vaccine in the elderly. Vaccine 7(4):303-308.
Guirnalda, P.D., K. Malinowski, V. Roegner and D.W. Horohov. 2001. Effects of age and recombinant equine somatotropin (eST) administration on immune function in female horses. J. Anim. Sci. 79(10):2651-
8.
Higgins, D.A., J.R. Carlson and G. van Nest. 1996. MF59 adjuvant enhances the immunogenicity of influenza vaccine in both young and old mice. Vaccine 14(6):478-484.
Horohov, D.W. 2000. Equine T cell cytokines: protection and pathology. Vet. Clin. NA Equine Pract. 16:1-4. Horohov, D.W., J.H. Kydd and D. Hannant. 2002. The effect of aging on T cell responses in the horse. Dev. Comp. Immunol. 26(1):121-128.
Horohov, D.W., A. Dimock, P. Guirnalda, R.W. Folsom, K.H. McKeever and K. Malinowski. 1999. Effect of exercise on the immune response of young and old horses. Am. J. Vet. Res. 60(5):643-647.
Hutter, D., Y. Yo, W. Chen, P. Liu, N.J. Holbrook, G.S. Roth and Y. Liu. 2000. Age-related decline in Ras/ERK mitogen-activated protein kinase cascade is linked to a reduced association between Shc and EGF
receptor. J. Gerontol. A Biol. Sci. Med. Sci. 55(3):B125-34.
Iorio, A.M., A. Alatri, B. Camilloni, M. Neri, G. Baglio and I. Donatelli. 1999. Antibody response to 1995-1996 influenza vaccine in institutionalized and noninstitutionalized elderly women. Gerontology 45(1):31-8.
Kajino, K., Y. Kajino and M.I. Greene. 1998. Fas- and perforin-independent mechanism of cytotoxic T lymphocyte. Immunol. Res. 17(1-2):89-93.
Kiecolt-Glaser, J.K., R. Glaser, S. Gravenstein, W.B. Malarkey and J. Sheridan. 1996. Chronic stress alters the immune response to influenza virus vaccine in older adults. Proc. Natl. Acad. Sci. USA 93 (7):3043-3047.
Klinman, N.R. and G.H. Kline. 1997. The B-cell biology of aging. Immunol. Rev. 160:103-114.
Kurashima, C., M. Utsuyama, M. Kasai, S.A. Ishyima, A. Konno and K. Hirokawa. 1995. The role of the thymus in the aging of the Th cell subpopulations and the age-associated alterations of cytokine
production by these cells. Int. Immunol. 7:97-104.
Lind, E.O., J. Hoglund, B.L. Ljungstrom, O. Nilsson and A. Uggla. 1999. A field survey on the distribution of strongyle infections of horses in Sweden and factors affecting faecal egg counts. Equine Vet. J. 31(1):68-72.
Lio, D., C.R. Balistreri, G. Candore, C. D’Anna, G. Di Lorenzo, F. Gervasi, F. Listi, L. Scola and C. Caruso. 2000. In vitro treatment with interleukin-2 normalizes type-1 cytokine production by lymphocytes from
elderly. Immunopharm. Immunotox. 22(2):195-203.
Lipschitz, D.A. 1987. Nutrition, aging and the immunohematopoietic system. Clin. Geriatr. Med. 3(2):319-328.
Liu, B., K.W. Carle and R.L. Whisler. 1997. Reductions in the activation of ERK and JNK are associated with decreased IL-2 production in T cells from elderly humans stimulated by the TCR/CD3 complex and
costimulatory signals. Cell. Immunol. 182(2):79-88.
Lunn, D., G. Soboll, B. Schram, J. Quass, M. McGregor, R. Drape, M. Macklin, D. McCabe, W. Swain and C. Olsen. 1999. Antibody response to DNA vaccination of horses using the influenza virus hemagglutinin gene. Vaccine 17:2245-2258.
Lunn, D.P., M.A. Holmes and P.H. Duffus. 1993. Equine T-lymphocyte MHC II expression: variation with age and subset. Vet. Immunol. Immunopathol. 35:225-238.
Malaguarnera, L., L. Ferlito, R.M. Imbesi, G.S. Gulizia, S. Di Mauro, D. Maugeri, M. Malaguarnera and A. Messina. 2001. Immunosenescence: a review. Arch. Gerontol. Geriatr. 32(1):1-14.
Malinowski, K., R.A. Christensen, A. Konopka, C.G. Scanes and H.D. Hafs. 1997. Feed intake, body weight, body condition score, musculation and immunocompetence in aged mares given equine somatotropin. J. Anim. Sci. 75(3):755-760.
Mazari, L. and B.M. Lesourd. 1998. Nutritional influences on immune response in healthy aged persons. Mech. Ageing Dev. 104(1):25-40.
Mbawuike, I.N., A.R. Lange and R.B. Couch. 1993. Diminished influenza A virus-specific MHC class Irestricted cytotoxic T lymphocyte activity among elderly persons. Viral Immunol. 6(1):55-64.
Mbawuike, I.N., C. Acuna, D. Caballero, K. Pham-Nguyen, B. Gilbert, P. Petribon and M. Harmon. 1996. Reversal of age-related deficient influenza virus-specific CTL responses and IFN-gamma production by monophosphoryl lipid A. Cell. Immunol. 173(1):64-78.
Miller, R.A. 1991. Aging and immune function. Int. Rev. Cytol. 124:187-215.
Miller, R.A., G. Garcia, C.J. Kirk and J.M. Witkowski. 1997. Early activation defects in T lymphocytes from aged mice. Immunol. Rev. 160:79-90.
Mocchegiani, E., R. Giacconi, M. Muzzioli and C. Cipriano. 2000. Zinc, infections and immunosenescence. Mech. Ageing Dev. 121(1-3):21-35.
Mysliwska, J., E. Bryl, J. Foerster and A. Mysliwski. 1998. Increase of interleukin 6 and decrease of interleukin 2 production during the ageing process are influenced by the health status. Mech. Ageing Dev.
100(3):313-328.
Parham, P. 2000. The Immune System. 1st Ed., Garland, London.
Pawelec, G., M. Adibzadeh, A. Rehbein, K. Hahnel, W. Wagner and A. Engel. 2000. In vitro senescence models for human T lymphocytes. Vaccine 18(16):1666-1674.
Perryman, L.E., C.R. Wyatt, N.S. Magnuson and P.H. Mason. 1988. T lymphocyte development and maturation in horses. Anim. Genet. 19(4):343-348.
Phelouzat, M.A., T. Laforge, A. Arbogast, R.A. Quadri, S. Boutet and J.J. Proust. 1997. Susceptibility to apoptosis of T lymphocytes from elderly humans is associated with increased in vivo expression of
functional Fas receptors. Mech. Ageing Dev. 96(1-3):35-46.
Ponnappan, U. 1998. Regulation of transcription factor NF kappa B in immune senescence. Front. Biosci. 3:D152-168.
Potestio, M., C. Caruso, F. Gervasi, G. Scialabba, C. D’Anna, G. Di Lorenzo, C.R. Balistreri, G. Candore and G.C. Romano. 1998. Apoptosis and ageing. Mech. Ageing Dev. 102(2-3):221-37.
Proudman, C.J., M.A. Holmes, A.S. Sheoran, S.E. Edwards and A.J. Trees. 1997. Immunoepidemiology of the equine tapeworm Anoplocephala perfoliata: age-intensity profile and age-dependency of antibody subtype responses. Parasitology 114(Pt 1):89-94.
Quadri, R.A., O. Plastre, M.A. Phelouzat, A. Arbogast and J.J. Proust. 1996. Age-related tyrosine-specific protein phosphorylation defect in human T lymphocytes activated through CD3, CD4, CD8 or the IL-2 receptor. Mech. Ageing Dev. 88(3):125-138.
Ralston, S., C. Nockels and E. Squires. 1988. Differences in diagnostic test results and hematologic data between aged and young horses. Am. J. Vet. Res. 49:1387.
Remarque, E.J., J.M. de Jong, R.J. van der Klis, N. Masurel and G.J. Ligthart. 1999. Dose-dependent antibody response to influenza H1N1 vaccine component in elderly nursing home patients. Exp. Gerontol 34(1):109-115.
Remarque, E.J., W.C. van Beek, G.J. Ligthart, R.J. Borst, L. Nagelkerken, A.M. Palache, M.J. Sprenger and N. Masurel. 1993. Improvement of the immunoglobulin subclass response to influenza vaccine in elderly nursing-home residents by the use of high-dose vaccines. Vaccine 11(6):649-654.
Rink, L., I. Cakman and H. Kirchner. 1998. Altered cytokine production in the elderly. Mech. Ageing Dev. 102(2-3):199-209.
Rukavina, D., G. Laskarin, G. Rubesa, N. Strbo, I. Bedenicki, D. Manestar, M. Glavas, S.E. Christmas and E.R. Podack. 1998. Age-related decline of perforin expression in human cytotoxic T lymphocytes and natural killer cells. Blood 92(7):2410-2420.
Saini, A. and Y. Sei. 1993. Age-related impairment of early and late events of signal transduction in mouse immune cells. Life Sci. 52(22):1759-1765.
Schmucker, D.L., K. Thoreux and R.L. Owen. 2001. Aging impairs intestinal immunity. Mech. Ageing Dev. 122(13):1397-1411.
Solana, R., and G. Pawelec. 1998. Molecular and cellular basis of immunosenescence. Mech Ageing Dev 102 (2-3):115-29.
Solana, R. and E. Mariani. 2000. NK and NK/T cells in human senescence. Vaccine 18(16):1613-20.
Subbarao, K. 1999. Influenza vaccines: present and future. Adv. Virus Res. 54:349-373.
Sunderkotter, C., H. Kalden and T.A. Luger. 1997. Aging and the skin immune system. Arch. Derm. 133(10):1256-1262.
Vedhara, K., N.K. Cox, G.K. Wilcock, P. Perks, M. Hunt, S. Anderson, S.L. Lightman and N.M. Shanks. 1999. Chronic stress in elderly carers of dementia patients and antibody response to influenza vaccination. Lancet 353(9153):627-631.
Whisler, R.L., L. Beiqing and M. Chen. 1996a. Agerelated decreases in IL-2 production by human T cells are associated with impaired activation of nuclear transcriptional factors AP-1 and NF-AT. Cell. Immunol. 169(2):185-195.
Whisler, R.L., Y.G. Newhouse and S.E. Bagenstose. 1996b. Age-related reductions in the activation of mitogen-activated protein kinases p44mapk/ERK1 and p42mapk/ERK2 in human T cells stimulated via
ligation of the T cell receptor complex. Cell. Immunol. 168(2):201-210.
Whisler, R.L., M. Chen, L. Beiqing and K.W. Carle. 1997. Impaired induction of c-fos/c-jun genes and of transcriptional regulatory proteins binding distinct cfos/c-jun promoter elements in activated human T
cells during aging. Cell. Immunol. 175(1):41-50.
Wyde, P.R., H.R. Six, M.W. Ambrose and B.J. Throop. 1989. Influenza virus infection and bacterial clearance in young adult and aged mice. J. Gerontol. 44(5):B118-124.
Yang, X., J. Stedra and J. Cerny. 1996. Relative contribution of T and B cells to hypermutation and selection of the antibody repertoire in germinal centers of aged mice. J. Exp. Med. 183(3):959-970.
Zheng, B., S. Han, Y. Takahashi and G. Kelsoe. 1997. Immunosenescence and germinal center reaction. Immunol. Rev. 160:63-77.
Authors: DAVID W. HOROHOV and DANIEL FERMAGLICH
Maxwell H. Gluck Equine Research Center, Department of Veterinary Science, University of Kentucky, Lexington, Kentucky, USA