Take Home Message
- US dairy industry sustainability is increasingly important as producers are challenged with increasing dairy product supply to meet the demands of the growing population, while maintaining the tradition of environmental stew ardship
- Advances in nutrition, management and genetics resulted in a four - fold improvement in milk yield between 1944 and 2007. This allowed the US dairy industry to produce 59% more milk using 64% fewer cows and conferred considerable reductions in fee d (77%), land (90%) and water (65%) use per gallon of milk. The carbon footprint of the entire US dairy industry was reduced by 41% over the same period.
- The global livestock industry is thought to contribute 18% of greenhouse gases worldwide. However, this global average does not address variability between systems. Differences in system productivity demonstrate the considerable variation in environmental impact between dairy regions.
- As dairy industries worldwide pledge to reduce total greenhouse ga ses emissions, attention should be focused on a whole - system approach rather than a "magic bullet" solution that may confer negative trade - offs.
- Improving productivity has the greatest potential to reduce the environmental impact of dairy production, reg ardless of system characteristics.
Introduction
Sustainabilty is often defined as "meeting society's present needs without compromising the ability of future generations to meet their own needs" (U.S. EPA, 2010). This is particularly important for food production as the global population is predicted to increase to 9.5 billion people in the year 2050 (U.S. Census Bureau, 2008), increasing total food requirements by 70% (Food and Agriculture Organization of the United Nations, 2009, Tilman et al., 2002). Competition for water, land and energy is predicted to increase and the global dairy industry faces the challenge of producing sufficient safe, affordable milk to meet consumer demand, using a finite resource base. This paper will discuss the role of produ ctivity in producing sufficient milk to supply the human population, whilst reducing environmental impact.
The myth of low - input systems
In 1611, European dairy cattle were imported into the USA, providing the basis for the US dairy industry. Since that p oint, huge productivity gains have been made: the earliest recorded US milk production data relates to a Jersey cow that produced 511 lb of milk over 350 days in 1854 (Voelker, 1992). By contrast, the average milk yield per cow in 2007 was 20,203 lb/year. As dairy producers moved away from the pasture - based systems of the 1940's to today modern practices, advances in nutrition, management and genetics allowed milk production per cow to increase and decreased the size of the national herd. Nonetheless, imp roved efficiency is not always perceived as being beneficial. The popular agrarian vision of US dairy farming involves cows grazing on pasture with a red barn in the background ± a traditional low - input system. By contrast, modern dairy production is consi dered by anti - animal agriculture activists to be synonymus with "filthy and desease-ridden conditions" and industrialized warehouse- like facilities that significantly increase greenhouse gas emissions per animal"( Koneswaran and Nierenberg, 2008). Some agencies suggest that adopting low - input production systems may be the key to improving agricultural sustainability. According to the First Law of Thermodynamics ("energy can neither be created or destroyed, it can only change form"), a low - energy - input system must be a low - energy - output system, characterized by reduced productivity over a fixed time period. Nonetheless, improved productivity within livestock production appears to be a profane suggestion, despite a recent FAO report concluding that to reduc e environmental impact there exists "a need for cotinued efficiency gains in resourse use for livestock production " (Steinfeld et al., 2006).
The dilution of maintenance effect
In any business, improving productive efficiency (output per unit of resourc e input), spreads fixed costs over more units of production, allowing the product to be produced at a lower economic cost. Within dairy systems each animal's mainteinance nutrient requirement acts as a fixed cost that must be paid before production (growth , pregnancy or lactation) can occur. These requirements are associated with both economic and environmental costs in terms of resource inputs (feed, water, cropland, fertilizer, fossil fuels) and waste outputs (greenhouse gases (GHG), manure ). The "dilution of maintenance" effect is shown in Figure 1.
As milk yield increases from 15 lb/day to 65 lb/day, maintenance requirements do not change, but are diluted out over more units of production and thus being reduced from 69% to 33% of the total. In this cas e, energy is also a proxy for resources in terms of feed, land, water and fossil fuels. This effect is not confined to the lactating cow but applies to the entire dairy herd. As productivity (milk yield) increases, fewer lactating cows are required to prod uce a set amount of milk and the number of associated support animals (dry cows, replacement heifers, bulls) that serve to maintain dairy herd infrastructure is concurrently reduced. The environmental impact per unit of milk is therefore reduced through di lution of maintenance at both the individual cow and the dairy population level (Capper et al., 2009, Pinares - Patiño et al., 2009).
Improving productivity reduces environmental impact
Since 1944, the US national herd has shifted away from the Jersey and Guernsey to the greater - volume producing Holstein cow. In 2007, Holstein cows produced an average of 18.2 lb more milk per day than Jersey cows (DairyMetricsTM, Raleigh, NC), however, they also have an increased bodyweight, a greater maintenance nutrient cost. As shown by Capper et al. (2009), daily GHG output per animal has increased from 61.3 lb CO2-equivalents compared to 29.8 lb CO2-equivalents in 1994 (Capper et. al. 2009) (Figure 2). However, expressing results on a "per head" basis fail to take the entire system into account. When the entire system is included in the analysis, it is clear that GHG emissions per lb of milk produced have declined from 3.66 lb in 1944 to 1.35 lb in 2007. This has been achieved through considerable improvements in productivity. Annual milk yield per cow increased over three-fold between 1944 (4,572 lb) and 2007 (20,203 lb), allowing 59% more milk (186 billion lb vs. 117 billion lb) to be produced using 64% fewer lactating cows (9.2 million vs. 25.6 million). Despite the increase in total milk production, the carbon footprint of the US dairy industry was reduced by 41% by improving productivity between 1944 and 2007 (Figure 3).
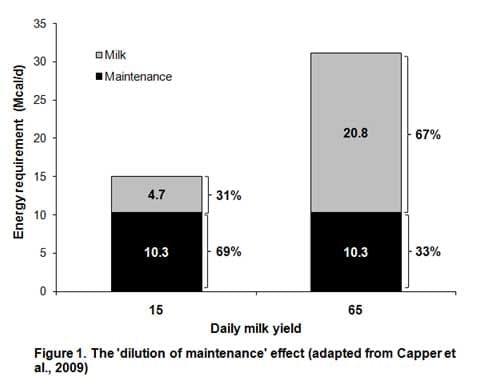
Climate change is a significant global concern, however, environmental impact should not simply be assesed on the basis of GHG emissions or "carbon footprints". The livestock industry faces significant challenge competition for resources as the global population increases, particularly in terms of water, land and energy. As Capper et al. (2009) demonstrated, improved dairy productivity between 1944 and 2007 resulted in a 79% decrease in total animals (lactating and dry cows, heifers, mature and adolescent bulls) required to produce a set quantity of milk. Feed and water use were reduced by 77% and 65% respectively, while cropland required for milk production in 2007 was reduced by 90% compared to 1944 due to improved crop yields and the shift from pasture-based to TMR systems. Finally, manure output from producing a comparable amount of milk from the modern system was 76% lower than from the 1944 system, contributing to the aforementioned 63% decrease in the carbon footprint per unit of milk.
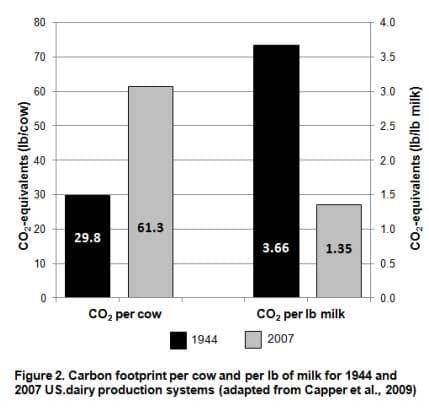
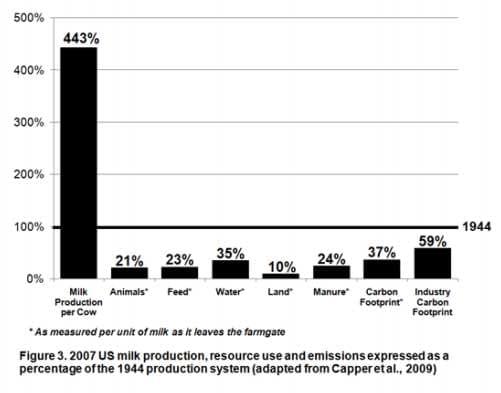
Productivity and environmental impact of pasture-based systems
Grazing livestock systems provide an invaluable food production service by converting indigestible plant material into high-quality animal protein for human consumption. The majority of land used for grazing ruminants is not suitable for growing crops for human consumption (Steinfeld et al., 2006), therefore pasture-based livestock production provides an opportunity to feed the human population without competing for grain-based food resources. However, pasturebased systems can only gain a comparative advantage over conventional dairying when they support meat or milk production, without negatively impacting yield or increasing resource use per unit of food. This is a serious consideration, as cows in pasture-based systems have increased daily maintenance costs and decreased milk yields (Kolver, 2003, Sato et al., 2005) (Figure 4).
A recent analysis from the Organic Center intended to demonstrate the environmental advantages of organic dairy production was based on a flawed premise, namely that productivity (milk yield per cow) does not differ between conventional and organic systems (Benbrook, 2009). Indeed, the reduced productivity exhibited by organic systems increases both the population size and land required to produce a set amount of milk (Capper et al., 2008). High-forage or pasture-based diets also increase ruminal methanogenesis, thus increasing enteric GHG emissions (Johnson and Johnson, 1995, Pinares-Patiño et al., 2009) and the total carbon footprint of organic dairy systems (de Boer, 2003). When differences in productivity are accounted for, organic dairy production requires considerably more resources per unit of milk produced and has a greater environmental impact (Capper et al., 2008).
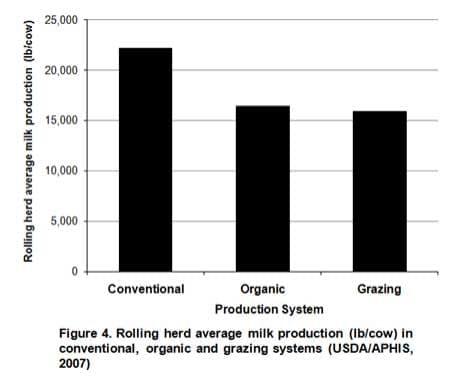
Carbon sequestration into plant or soil biomass is often quoted as a major environmental advantage of pasture-based systems. However, pasture does not sequester carbon indefinitely, nor does it occur at a constant rate. Over time, soil carbon concentrations reach an equilibrium point, beyond which no further sequestration occurs unless land is subjected to management change e.g. tillage, application of organic matter, conversion to crop or pastureland (Post and Kwon, 2000, Schlesinger, 2000). Carbon sequestration should therefore be considered a temporary strategy for reducing environmental impact (Congress of the United States, 2007). Relying on temporary sequestration strategies to overcome the effects of low productivity in pasture-based systems is a definitively unsustainable strategy
Global environmental carbon footprints are not representative of regional production systems
In the oft-quoted report "Livestock's Long Shadow", the Food and Agriculture Organization of the United Nations (Steinfeld et al., 2006) reported that livestock production is responsible for 18% of global anthropogenic GHG emissions. This statistic has since been quoted by many activist groups as evidence that abolishing animal agriculture would have a beneficial environmental impact (Koneswaran and Nierenberg, 2008, McMichael et al., 2007, Millward and Garnett, 2010, The Humane Society of the United States, 2008). By contrast, the US EPA (2009) quantified primary anthropogenic GHG sources within the US, concluding that total agriculture (livestock and crops) contributed 5.8% of national GHG emissions. This 5.8%, can be further partitioned into approximately 3.4% from animal agriculture the remaining 2.4% to human food crops. To reconcile the considerable difference between the global (18%) and national (3.4%) estimates of livestock's contribution to GHG emissions, it is therefore necessary to explore the data in more detail.
Pitesky et al. (2009) reported several flaws in the FAO's methodology and discuss the inadequacy of a "global average" as a measure of individual system environmental impact. Close examination of the global FAO figure reveals that almost half (48%) of total carbon emissions are attributed to carbon released by clearing forestland to grow animal feed. The potential for reduced cropland availability to lead to further deforestation on a global basis is exacerbated by the use of formerly food-producing agricultural land to grow biofuel crops (Sawyer, 2008). Deforestation therefore needs to be taken into account when analyzing the environmental impact of agricultural systems where a considerable portion of animal feed is imported, e.g. imports of soy from South America into Europe. By contrast, the majority of US feedstuffs are produced domestically: cropland area has remained relatively stable (USDA, 2002) with increased crop yields compensating for required increases in feed and food crop production. In contrast to the deforestation occurring in South American countries, the US is actively reforesting, with an average increase in forestland area of 0.2%/year over the past 30 years (Smith et al., 2005). Reforestation increases carbon sequestered from the atmosphere into plant tissue, with an average of 14.1 lb carbon sequestered annually per (mature) tree (Sampson and Hair, 1996). The mitigating effect of carbon sequestered by new forest growth is not accounted for in the US EPA (2009) calculations.
If the component of total GHG emissions attributed to deforestation is disregarded, the global estimate still remains approximately 3x higher than the US national estimate. As previously discussed, livestock's environmental impact is directly affected by system productivity, yet by its very nature, the global average includes a wide range of regional system efficiencies. In highly efficient US dairy production systems, the average cow produced 20,203 lb/year By contrast, the average annual yield for the top 6 milk-producing counties in Europe was 14,026 lb milk per year and annual production in Canada and New Zealand averaged 18,051 lb milk/cow and 8,380 lb milk/cow respectively (FAO, 2009).
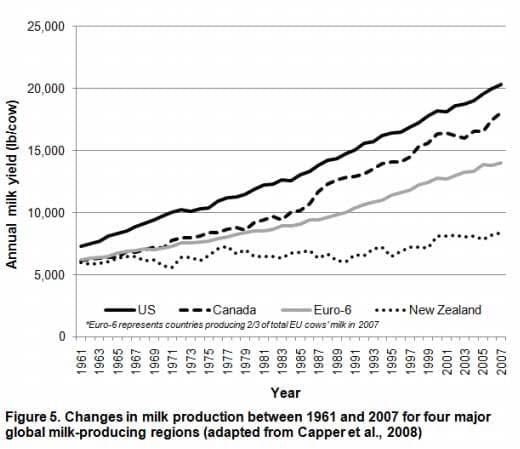
Figure 5 shows trends in milk production per cow from 1961 to 2007 for the US, Canada, an aggregate of the top-6 milk producing countries in Europe (Netherlands, UK, Germany, France, Italy, Poland) and New Zealand. Milk yields were relatively similar in 1961, however, trend lines have diverged markedly over time. The US has shown the fastest rate of improvement, Canada and Europe are intermediate and New Zealand production has remained relatively static. Productivity improvements in the US, Canada and Europe were facilitated by advances in genetics, nutrition, management and animal health. Differences in the rate of improvement between various regions may be partially explained by the attitude towards and the adoption of technology and innovative management practices. The US is generally pro-biotechnology whereas Europe is less receptive (Moses, 1999, Wilcock et al., 2004). The New Zealand system is extensive, pasture-based, has a lower emphasis on productivity than that displayed by the US dairy industry and has an average lactation length of only 252 days (LIC, 2008). As discussed previously, increased productivity reduces the environmental impact of dairy production as fewer animals, and therefore a reduced total population body mass and maintenance cost, are required to produce the same amount of milk.
This paper was presented at the Minnesota Dairy Health Conference 2011, May 17-19, 2011. Engormix thanks the author and the organizing committee for this contribution.