Abstract
Limiting factors to the tilapia farming industry are numerous and deeply embedded in the environmental paradigm. Infectious diseases are moved to the top list of limiting factors that harshly endangering the fish health with consequent negative impact on the entire population ecology. Antibiotic selection is of major time-wise and economical importance when an episode of bacterial infection has swiftly attacked certain cultured fish population. In the current study an in-vitro microcosm system has been established to assess the efficacy of two major broad spectrum antibiotics against two septicemic / saprophytic fish pathogens. Theantibiogram as well as histopathological examination of the tissue sections of the challenged tilapias have indicated that Florfenicol is more effective than Enrofloxacin in combating Clostridium perfringens infection. However, Enrofloxacin has proved a superior efficacy against Pseudomonas florescence infections. Both antibiotics have regulated the nitric oxide / nitric oxide intermediates with an ultimate neutralization of the damage associated with oxidative stresses. Further, the two antibiotics were able to modulate the innate humoral immune responses of the challenged fish. Evaluation of the immunological response results of the challenged fish has lead us to conclude that, the therapeutic doses of both antibiotics were able to modulate innate, humoral as well as antioxidant defenses of the challenged fish. Analysis of the water quality measures of the challenged fish aquaria has revealed a relative increase in water pH and ammonia concentrations in Clostridium as well as Pseudomonas challenged aquaria. As a positive indicator, ammonia and pH concentration were markedly decreased in both Florfenicol and Enrofloxacin treated aquaria. However, it is worthy to mention that ammonia and pH concentrations were sharply decreased in Florfenicol than Enrofloxacin treated fish aquaria. In conclusion, we recommend that antibiotic of choice can be effectively used not only to treat fish but also to modulate their immune response as well as improve the surrounding environmental water parameters.
Keywords
Florfenicol, Enrofloxacin, Clostridium perfringens, Pseudomonas florescence, immune response, water quality.
Introduction
The rapid and ongoing development of all aquaculture sectors continues to call for better health management and improved capacity to face new health challenges (Subasinghe, 2009). This is particularly apparent with increased interest in species diversification, as well as new grow-out techniques. The rapid expansion of these sectors continually surpasses the rate of education, research and adaptation of expertise in health management. The actual risks have to be assessed frequently through controlled and repeated experimental challenges - in addition to extensive field surveys and epidemiological data collection (e.g. husbandry and environmental factors associated with the disease outbreak). Although our capability to manage most of these health issues has grown immensely over the last 20-30 years, the new challenges continue to call for further improvements (Subasinghe, 2009).
Currently, Egypt is considered one of the fastest growing countries in the field of aquaculture (FAO, 2005). Nile tilapia (Oreochromis niloticus) is the most widely farmed fish species in Egypt. There are several limiting factors affecting the tilapia farming industry in Egypt, including infectious diseases, nutritional disorders, environmental pollution and some managerial factors (Eissa et al., 2009). Infectious diseases are the most eminent etiologies that put the live of fishes into jeopardy with consequent negative impact on growth, fecundity and productivity (Diab et al., 2004; Eissa et al., 2009).
Measures to combat diseases of fish have only recently assumed a high priority in many aquaculture producing countries/regions of the world. Development of such measures was stimulated by the serious socio-economic losses and environmental impacts caused by aquatic animal diseases, as well as threats to food availability/security and the livelihoods of vulnerable sectors of society. Many countries have improved their policies and legislature, laboratory facilities, diagnostic expertise, control protocols, and therapeutic strategies, in order to handle disease outbreaks better (Subasinghe, 2009).
The prophylactic use of drugs, chemicals and biologicals are necessary to meet the sanitation standards used to maintain high health status/certification. Prophylactic drugs are also used to minimize diseases caused by opportunistic infectious agents, and prevent spread via personnel and equipment. Chemotherapy has value in preventing and controlling aquatic animal diseases, but must be used in a careful manner (Subasinghe, 2009).
Keeping fish alive, growing better and productive can be achieved by implementing a competent control panel for infectious diseases at different production stages. For several decades, it has been shown that chemotherapy is the magic solution for the control of bacterial disease outbreaks in a certain aquaculture facility. This is not only because of the curative effect of such chemotherapeutic on each individual diseased fish but also due to the ability of such agent to minimize the bacterial load in a certain aquaculture pond and in turn its superior potency to stop mortalities. Minimizing the bacterial load in the aquaculture pond will consequently give an ideal chance for the fish immune system to recover from the continuous attacks of the bacterial pathogens as well as their toxins.
The danger of bacterial attacks not only restricted to those of specific fish pathogenic nature but also extends to saprophytic bacteria which exist naturally in pond water and / or mud as well as gastrointestinal tract of aquatic species. Pseudomonas fluorescence and Clostridium perfringens represent an ideal model for both pathogenic as well as saprophytic bacteria which directly / indirectly impact vulnerable fishes either through bacterial invasion (P. fluorescence) or potent toxin production (C. perfringens).
Pseudomonas septicemia is one of the most prevalent fish diseases in aquaculture due to its ubiquitous nature in aquatic environment (Ahne et al., 1982 and Fernandez et al., 1990). P. florescence can induce typical form of acute septicemia which is manifested by the appearance of severe fin rot, skin ulcers, nervous manifestation and finally acute episodes of deaths in immune-compromised fishes (Ahne et al., 1982; Fernandez et al., 1990; Austin and Austin 2007). Thus, the selection of suitable antibiotic for the control of pseudomonas septicemia is of prime importance for fish management team at any aquaculture facility.
On the other side, C. perfringens type A is the most commonly isolated clostridia from earthen pond raised fishes (Aschfalk and Muller, 2002). Despite the fact that, some researchers have hypothesized that C. perfringens does not belong to the normal bacterial fish flora, it could contribute to the contamination of their aquatic habitat (Edwards et al., 1998). C. perfringens is frequently found in the environment, particularly in naturally fertilized soil (Myers et al., 2006), contaminated ground water ( Conboy and Gross , 2001 ) ,organic polluted surface water (El- Sarangawy,1990) and river sediment (Davies et al.,1995) as well as the intestinal tract of terrestrial animals (Itodo et al.,1986 ). The uprising development of antibacterial resistance against many of the frequently used antibacterial combinations in aquaculture was mainly due to the faulty use of such drugs (WHO, 1999; Midvedt and Lingass, 1992; Stamm, 1989). This fact necessitates the development of new drug formulas to overcome the resistance mechanisms (Midvedt and Lingass, 1992).
The risk of selecting drug resistance in fish-pathogenic or water-associated bacteria, some of them being likely to become involved in human health, probably remains the most critical concern in fish health control practice. Enrofloxacin and Florfenicol represent the latest generation of antibacterial agents which have been specifically developed for the treatment of various bacterial infection of aquatic origin (Carty et al., 2007, Feng et al., 2008). Florfenicol is a fluorinated analog of thiamphenicol (Fukui et al.1987) specifically developed for use in veterinary medicine as an alternative to chloramphenicol, an antibiotic banned for use in food producing animals (Varma et al. 1986). Enrofloxacin is a molecule that belongs to the quinolone antimicrobial family. It has received growing attention because of its potential efficacy for the treatment of diseases in fish (Dalsgaad and Bjerregaard, 1991; Lewbart et al. 1997; Della Rocca et al., 2004). This drug is a fluorinated quinolone carboxylic acid derivative, and it has been widely used in veterinary medicine because of its broad spectrum of activity (Drlica, 1999). Although these antibiotics have their positive side in dealing with aquatic bacterial invasions, yet, they still have several side effects on both fish and the surrounding aquatic environment. Immunosuppression could be the most critical end result of random / chronic use of antibiotics in a certain aquatic facility (Graslund et al., 2003). Therefore, it is important to determine whether such antibiotic could modulate the immune responses (innate and humoral) and subsequently affect the ability of fish to resist bacterial pathogens.
Florfenicol is rapidly absorbed from the water into fish tissues. Because therapeutic levels of this compound are readily absorbed within the fish, it has provided effective control of bacterial infection when administered at 0.5 μg of drug mL-1 of water (Holt et al., 1975; Cipriano, 2005). Enrofloxacin bath consisting of 2.5 mg/L is recommended as the most effective therapeutic dose against most of the susceptible bacterial pathogens (Gore et al., 2005).
The fish immune responses comprise both innate as well as humoral response (Ellis, 1999). The prime immune response is mainly based on anterior kidney, spleen and few cells in the liver in case of systemic infections. Innate responses are the most reactive partner of the immune system (Ellis, 1999). Their response is swift, direct and effective in fighting microbial invasions. Phagocytes are the most important cells in these defenses; they are supported by several factors such as serum agglutinins, precipitins and lysozyme (Bohn, 1999; Chow et al., 1999; Boes, 2000). The antioxidant activity of fish (superoxide dismutase, catalases and peroxides) also constitutes an array of defense mechanisms which react swiftly upon exposure to microbial invasions (Olsviket al., 2006). NO is critically involved in non-specific and immunological host defense where it acts as an endogenous antibacterial mediator during infections (Umezawa et al., 1997; Granger et al., 1998). Schoor & Plumb (1994) demonstrated inducible NO production, using enzyme histochemical techniques, from the anterior kidney of channel catfish (Ictalurus punctatus) infected with Edwardsiella ictaluri. Recently, Stafford et al. (2001) have characterized the molecules present in crude leukocyte supernatants that induce NO production in goldfish macrophages, suggesting that transferrin appears to be an important mediator for the activation of both fish macrophages and granulocyte.
The disease condition was found to be associated with the existence of stressful environmental role expressed by the recorded increase in values of water quality parameters especially ammonia and nitrite in affected ponds (Casselman, 1978). The pathogenesis of bacterial disease in aquatic animals is usually a multi-factorial. Variable factors related to the host, environment and the pathogen may work in concert to define the nature of the triggered course of infection (Sniesko, 1974). Environmental factors such as organic load, temperature, salinity and pH might be involved in triggering disease outbreaks and representing a potential danger to fish health especially in intensive aquaculture systems with pH range of 7.8 to 8.2 (Casselman, 1978; Sunaryanto and Mariam, 1986)
This study was initiated to investigate the effectiveness of the new, commercially available antibacterial substances and detect their ability to modulate the immune responses (innate and humoral) and subsequently affect the ability of fish to resist bacterial pathogens
Materials and Methods
Fish sampling
A total of 80 apparently healthy Oreochromis niloticus fish were collected from a semi-intensive aquaculture facility in Lower Egypt and brought alive to the wet lab of the department of Veterinary Hygiene and Management, Cairo University. The fish were divided into 8 sets of ten each and kept in well-aerated glass aquaria at 25 °C. Fish were examined for any abnormal behavioral changes, external signs, and gross lesions before the onset of the experiment. Bacterial isolates
Clostridium perfringens and Pseudomonas fluorescence with standard known biochemical and pathogenicity profiles were kindly obtained from the microbiological archive of the department of Microbiology, Animal Health Research Institute, Dokki, Egypt (Isolates were originally retrieved from clinically affected O. niloticus during a previous microbiological survey that encountered tilapias from different earthen ponds located within the scope of Giza province). Bacterial isolates were reconstituted and aliquoted in 2ml microfuge tubes containing glycerol - phosphate buffered saline (pH 7.4 - 1: 1 vol / vol). The bacterial isolates aliquots were stored at the - 80 freezer till the onset of the experimental challenge. The stored tubes were thawed at the room temperature and agitated using electric vortex to ensure equal distribution of the bacteria within the suspension. Loopfuls from each bacterial isolate aliquot were inoculated into the suitable enrichment broths.
Preparation of the bacterial inoculums
Loopfuls from Clostridium perfringens aliquots were inoculated into sterile cooked meat broth then incubated at 25 ºC under complete anaerobic condition for 24 hours. The inoculated broths were checked for the required turbidity degree by matching against McFarland standard turbidity tubes. It came out that each ml of the inoculated broth contains 3 X 107 CFU. The pathogenicity of the C. perfringens isolates were carried out by the intra-dermal inoculation test in albino guinea pigs according to stern and Batty (1975).
Loopfuls from Pseudomonas florescence aliquots were inoculated into Trypticase soy broth (Becton, Dickinson and Company -BD, NJ - USA), and then incubated at 25ºC for 18-24 hours. The inoculated broths were checked for the required turbidity degree by matching against McFarland standard turbidity tubes. It came out that each ml of inoculated broth contains 2 X 107 CFU. The pathogenicity of the P. florescence isolates were carried out by the intra-peritoneal inoculation of O. niloticus (same experimental weight) with 0.2ml of the bacterial suspension and matching the obtained pathology to the typical pseudomonas florescence pathology reported by Miyazaki et al. (1984); Bullock and McLaughlin (1970).
Experimental design
Three sets of aquaria, each containing 10 O. niloticus were allocated for each type of bacterial inoculation. An additional two aquaria, each contained a total of 10 O. niloticus were allocated for negative control group.
Bacterial challenge
Before inoculation, The three sets of tilapias as well as negative control groups were transferred into an anesthetic tank that contains 200 mg / L MS222 (Tricaine methane sulfonate, Finquel-Argent Chemical Laboratories, Washington, USA)that were previously neutralized with an equal amount of sodium bicarbonate solution.
The first three sets of tilapias were inoculated intra peritoneal (IP) with 0.2ml of C. perfringens (3 X 107 CFU) while the second three sets of tilapias were intra-peritoneally inoculated with 0.2 ml of P. florescence (2 X 107 CFU). The two negative control sets of tilapias were inoculated with 0.2ml of sterile cooked meat broth and 0.2ml of sterile trypticase soy broth respectively.
Minimal inhibitory concentrations of antibiotics
The susceptibility of C. perfringens and P. florescence to both Enrofloxacin and Florfenicol antibiotics was studied and the minimal inhibitory concentrations (MIC) for the two antibiotics were determined according to the method described by Boyanova et al. (2005). In this method, C. perfringens and P. florescence cultures were prepared in Muller-Hinton broth. The turbidity of the inoculated broths was matched against McFarland standard turbidity tubes equivalent to two degrees of turbidity (Bauer et al. 1966). 100 µl of the bacterial suspensions were poured onto Muller-Hinton agar then spread over the agar surface using sterile L shaped spreader to ensure event distribution of the bacteria. Inoculated plates were left to dry then wells (7mm diameter) were punched into the agar using a sterile stainless steel borer. Volumes of 0.1 ml of two fold serial dilution of both antibiotics were poured into each well. The plates of C. perfringens were anaerobically incubated at 25˚C for 24 hours, while those of P .florescence were incubated at 25˚C for 24 hours. The diameter of inhibition zones was measured in millimeters. The MICs for both antibiotics were determined as the highest dilutions that were able to inhibit the growth of each pathogen.
Antibiotic treatment trial
After 24 hours post bacterial challenge, the Florfenicol was added to only two C. perfringens and P. florescence challenged aquaria as a bath treatment at a dose of 0.5 mg / L, while another two C. perfringens and P. florescence challenged aquaria were treated with Enrofloxacin at a dose of 2.5 mg / L. Both treatments were continued for 5 successive days without water renewal.
Serum separation. The blood samples were collected 3 & 6 days after the onset of the experiment from both negative control groups (broth injected groups) and bacterial challenged groups (positive control). A final set of samples was collected 3 & 6 days post treatment from antibiotics treated Pseudomonas and Clostridium challenged groups. A total volume of 500 μl of blood was drawn from the caudal vessels of tilapias (Michael et al., 1994) and collected into serum tubes. Blood was allowed to clot overnight at room temperature, then centrifuged at 400 × g for 10 min. Serum was separated and stored in sterile microfuge tubes at -20°C until further use.
Lysozyme activity assay. Serum lysozyme activity was measured by a turbidimetric assay according to Ellis (1990) whereas serum samples were collected at the same intervals mentioned above (tables 3, 4). Briefly, 25ul of each serum sample was added to the plate wells containing agarose gel diluted in 1% in phosphate buffer saline (PBS) in which Micrococcus lysodeikticus cell (50mg / 100ml agarose) had been dispersed. The diameter of clear zone formed around the wells after 24 hours was measured .The lysozyme concentration levels were obtained from logarithmic curve using standard lysozyme.
Nitric oxide assay. The nitric oxide (NO) level in each tested serum sample was measured using the method described by Rajaraman et al. (1998). Briefly NO levels in fish serum samples were measured using Griess reagent. A total volume of 100ul of each serum sample including the negative control was incubated with an equal volume of Griess reagent (1% sulphanilamide, 0.1% N-Naphthyl-ethylenediamine, 2.5% phosphoric acid) in a 96 microtiter plate well with flat bottom and incubated for 10 minutes at 27 ºC. After incubation, the optical density was recorded spectrophotometrically at 570 nm using an ELISA reader (Model 680, Biorad). Molar concentrations of NO2 were calculated from a standard curve generated from a graded series of NaNO2 concentrations.
Total antioxidant activity. A colorimetric diagnostic kit(Bio-Diagnostics Ltd., Worcestershire, U.K.)was used to determine the total antioxidant activity in fish sera. The protocol used in determination of total antioxidant activity in both challenged as well as negative control sera was adopted from the method described by Koracevic (2001).
Total serum protein. The serum total protein concentration was measured with a Bio-Rad Protein Assay Kit (Bio-Rad Laboratories Ltd., Hertfordshire HP2 7DX, United Kingdom) using bovine serum albumin (BSA) as a standard (Doumas, 1971).
Histopathology
Renal, hepatic, splenic, intestinal and branchial tissue samples from challenged and control negative groups were fixed in 10% neutral buffered formalin solution. Formalin fixed tissues were then processed and embedded in paraffin. Five-micron sections of tissue samples were stained with hematoxylin and eosin (H & E) using methods described by Bancroft et al. (1996).
Water quality sampling
A total of 8 samples were aseptically collected from the negative control pond as well as the challenged and treated ones. All samples were obtained in sterile plastic bottles and stored according to Standard Methods described by APHA 1995, then physico-chemically analyzed. The pH was measured using a waterproof digital combo pH meter (HI 98127 - Hanna instruments Inc., RI, USA).Total ammonia nitrogen (TAN) was determined according to the method described by Chattopadhyay (1998) while nitrite was measured using the standard method of Margiocco et al. (1983).
Statistics
The variables were grouped into three specified sets of responses for multivariate analysis, including magnitude of infection (degree of pathological alterations), antibiotic treatment (type, dose - efficacy) and immune function (cellular: lysozyme activity- nitric oxide level and humeral: total serum proteins). The data were analyzed for effects in response sets using nested multivariate analysis of variance (MANOVA) with treatment as the fixed factor, and water quality as a random factor hierarchically nested inside treatment. Subsequent to multivariate analyses, protected univariate ANOVA, with treatment as the fixed factor and water quality as the random factor nested inside treatment, was used to analyze variables in response sets. Pair-wise differences between treatments in each variable were identified with ANOVA (factors as above, significance level P < 0.05). SPSS Statistical software ver. 13 (SPSS, Inc.) was used for all statistical analyses.
Results
In vitro sensitivityresults indicated that C. perfringens isolates were sensitive to Florfenicol and Enrofloxacin. However, it was apparent that isolates were more sensitive to Florfenicol than Enrofloxacin. The MIC results for both antibiotics were 0.4 mg/L and 0.78 mg/L respectively (table 1). On the other side, P. Fluorescence isolates were sensitive to both antibiotics. Yet, Enrofloxacin was more effective than Florfenicol with MIC results of 0.01 mg/l and 1.6 mg/l for both antibiotics respectively (table 2). It is worthy to mention that the re-isolation of both pathogens from the intestine, kidney and spleen of the untreated group was successful while no bacterial isolates were retrieved from antibiotic treated group.
Clinically, the bacterial challenged groups which have received I.P. inoculums of the bacterial suspension (Clostridium / Pseudomonas) showed variant clinical picture and mortality records. After 3 days post inoculation a total of 4/10 and 2/10 tilapias were found dead from the Clostridium and Pseudomonas inoculated group respectively. Each of the inoculated groups showed the typical clinical picture that is associated with each bacterial species. The Pseudomonas challenged group showed typical fin and gill rot, moderate peticheal skin hemorrhages, ascitis, friable dark liver, congested spleen and kidneys. On the other side, the clostridium challenged group presents a severe form of skin hemorrhages, hemorrhagic myositis, congested gills, mild exophthalmia, congested stomach mucosa, congested friable liver and edema/liquefaction of the brain.
Plate 1: (A) Photomicrograph of Nile tilapia (negative control) liver showing normal configuration of the hepatic parenchyma (H&E, 200 X). (B) Photomicrograph of clostridium infected Nile tilapia (positive control) liver showing severe congestion of the vascular hepato-pancreatic area with degeneration of the hepatic parenchyma (H&E, 200 X). (C) Photomicrograph of the liver of Enrofloxacin treated clostridium infected Nile tilapia showing vacuolation of the hepatic parenchyma together with slight congestion (H&E, 200 X). (D) Photomicrograph of the liver of Florfenicol treated clostridium infected Nile tilapia showing vacuolar degeneration of the hepatic parenchyma (H&E, 200 X).
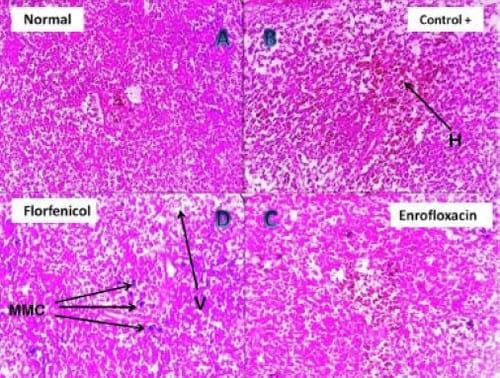
Plate 2: (A) Photomicrograph of (negative control) Nile tilapia Kidney showing normal renal tubular and glomerlular tissues (H&E, 200 X). (B) Photomicrograph of clostridium infected Nile tilapia kidney showing severe degeneration, necrosis (N) and disintegration of the renal tubular epithelium (H&E, 200 X). (C) Photomicrograph of the kidney of Enrofloxacin treated clostridium infected Nile tilapia showing vacuolar degeneration of the renal tubular epithelium (V-RT) and glomeruli (V-GL) (H&E, 200 X). (D) Photomicrograph of the kidney of Florfenicol treated clostridium infected Nile tilapia showing marked vacuolar degeneration of the tubular epithelium (H&E, 400 X).
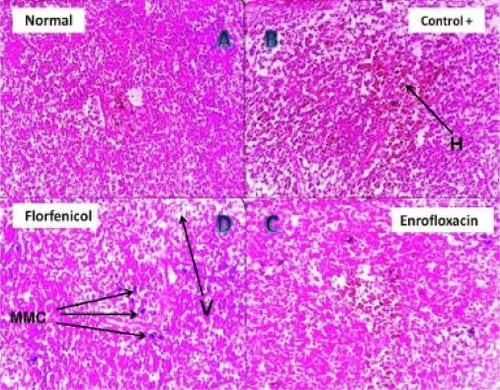
Plate 3: (A) Photomicrograph of (negative control) Nile tilapia spleen showing normal splenic lymphoid tissues (H&E, 200 X). (B) Photomicrograph of clostridium infected Nile tilapia spleen showing disintegration of the lymphoid follicles together with hemorrhagic areas (H) (H&E, 200 X). (C) Photomicrograph of the spleen of Enrofloxacin treated clostridium infected Nile tilapia showing minute hemorrhage, mild vacuolation and disorganization of the splenic tissue (H&E, 400 X). (D) Photomicrograph of the spleen of Florfenicol treated clostridium infected Nile tilapia showing solitary melanomacrophages (MMC) activation, mild vacuolation (V) and disorganization of the splenic tissue (H&E, 400 X).
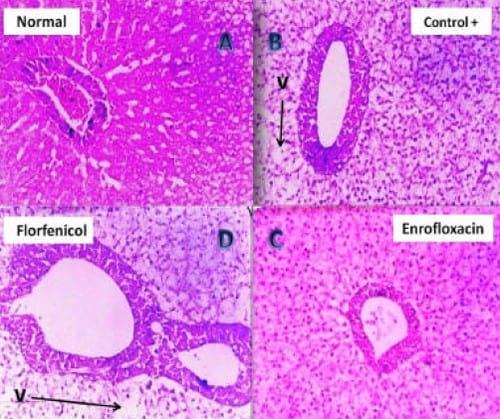
Plate 4: (A) Photomicrograph of Nile tilapia (negative control) liver showing normal configuration of the hepatic parenchyma (H&E, 200 X). (B) Photomicrograph of pseudomonas infected Nile tilapia (positive control) liver showing vacuolar degeneration (V) of the hepatic parenchyma (H&E, 200 X). (C) Photomicrograph of the liver of Enrofloxacin treated pseudomonas infected Nile tilapia showing mild vacuolar degeneration of the hepatic parenchyma (H&E, 200 X). (D) Photomicrograph of the liver of Florfenicol treated pseudomonas infected Nile tilapia showing moderate vacuolar (V) degeneration of the hepatic parenchyma (H&E, 200 X).
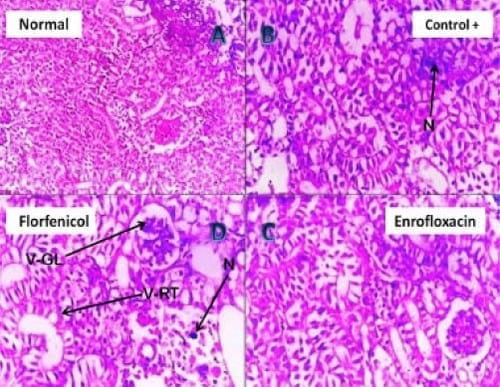
Plate 5: (A) Photomicrograph of (negative control) Nile tilapia Kidney showing normal renal tubular and glomerlular tissues (H&E, 200 X). (B) Photomicrograph of pseudomonas infected Nile tilapia kidney showing hydropic degeneration of the glomerlular (V-GL) and renal tubular epithelium (V-RT) (H&E, 200 X). (C) Photomicrograph of the kidney of Enrofloxacin treated pseudomonas infected Nile tilapia showing vacuolar degeneration of the renal tubular epithelium (H&E, 200 X). (D) Photomicrograph of the kidney of Florfenicol treated pseudomonas infected Nile tilapia showing vacuolar degeneration and necrosis (N) of tubular epithelium (H&E, 200 X).
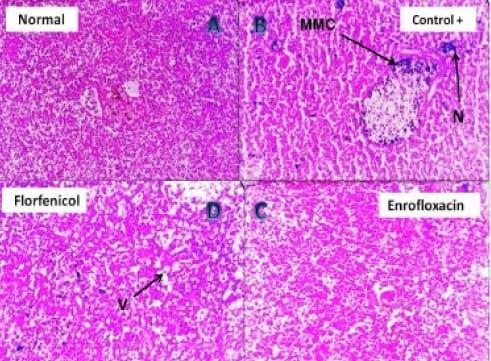
Plate 6: (A) Photomicrograph of (negative control) Nile tilapia spleen showing normal splenic lymphoid tissue (H&E, 200 X). (B) Photomicrograph of pseudomonas infected Nile tilapia spleen showing focal area of necrosis (N) with marked activation of melanomacrophage centers (MMC) (H&E, 200 X). (C) Photomicrograph of the spleen of Enrofloxacin treated pseudomonas infected Nile tilapia showing very mild vacuolation to apparently normal splenic tissue (H&E, 400 X). (D) Photomicrograph of the spleen of Florfenicol treated pseudomonas infected Nile tilapia showing solitary melanomacrophage activation, mild vacuolation (V) and disorganization of the splenic tissue (H&E, 400 X).
The intensity of clinical disease in both bacterial challenged groups was greatly diminished after the application of antibiotic baths. However, a sort of variation in the reported disease intensity was found to be associated with the type of antibiotic used in the treatment trial. For example, the Enrofloxacin treated pseudomonas group showed mild splenic / hepatic congestion with mild catarrhal gastritis / enteritis (manifested by the presence of moderate amount of mucous in both stomach and intestine) while those treated with Florfenicol presented more intense form of the disease i.e. exophthalmia, ascites, moderately congested friable liver, with mucoid contents oozing from the stomach mucoid contents oozing from the stomach and intestine. Histopathology of the liver (Plate 1(B)) and kidney (Plate 2(B)) of clostridium infected Nile tilapias revealed that different degrees of degenerative changes have been manifested in the parenchyma of both organs which have markedly progressed to necrosis and disintegration of the tubular epithelium in case of kidney. Splenic sections (Plate 3(B)) of this group revealed that the lymphoid follicles have faced a continuous pattern of disintegration together with hemorrhage, which appeared central to the examined splenic sections. In contrast, the splenic sections (Plate 3(B)) of the pseudomonas infected Nile tilapias revealed that the lymphoid follicles have suffered from a progressive stage of focal necrosis with marked activation of melanomacrophage centers (MMC). Further, the magnitude of histopathological alterations in liver (Plate 4 (B)) and kidneys (Plate 5 (B)) of the same group was much lighter than the clostridium infected groups where hydropic degeneration was the only finding.
The 200- X and 400- X microscopical examination of the liver (Plate 1, 4(C, D)) kidney (Plate 2, 5(C, D)) and spleen sections (Plate 3, 6(C, D)) of the Florfenicol and Enrofloxacin treated Nile tilapias, have revealed that magnitude of tissue alterations have sharply declined from severe degrees of degeneration, disintegration and necrosis to a milder forms of vacuolation with absence of necrotic changes. However, the magnitude of pathological alterations was markedly different between Florfenicol and Enrofloxacin treated Nile tilapias, especially when the species of the challenging bacteria was considered. For example, Florfenicol treated clostridium infected groups showed much lighter degrees of alterations when compared to Enrofloxacin treated group. Enrofloxacin treated pseudomonas infected groups presented another milder form of pathological alterations when compared to Florfenicol treated group.
Nitric oxide (NO) concentration: At the 3rd day post infection, nitric oxide concentration in serum samples of Pseudomonas florescence challenged group were elevated, then relatively reduced at the 6th day post challenge ( table 3). Similar results were obtained in case of Clostridium perfringens challenged groups (table 4). After antibiotic treatment the NO concentration was remarkably reduced in sera of challenged fish. In Pseudomonas florescence Florfenicol treated group, the reduction in NO concentration was highly significant at 3th daypost challenge.
Lysozyme activity: At the 3rd day post infection, Lysozyme activity in serum samples of both Pseudomonas florescence and Clostridium perfringens challenged groups were elevated (table 3, 4). The lysozyme activity was diminished after the antibiotics treatment to reach a stable level till the end of the experiment.
Antioxidant enzymes levels: At the 3rd day post bacterial challenge, the endogenous antioxidant enzyme levels in sera of Pseudomonas florescence challenged group were elevated and remained at its high levels till the 6th day post bacterial challenge when compared to the control negative group. Further, antioxidant enzymes activity in both Enrofloxacin and Florfenicol treated groups was significantly diminished in the 3rd and 6th day post-treatment (table 3). Similar, results were detected in the Clostridium perfringens challenged and treated groups with a significant reduction in the antioxidant activity at only the 6th day post-treatment (table 4).
Serum proteins:The serum protein levels in both Clostridium perfringens and Pseudomonas florescence challenged groups were elevated at the 3rd day post challenge then reduced after Enrofloxacin and Florfenicol treatment. These reduced levels of serum proteins continued without significant change till the 6th day post treatment (table 3, 4).
There was an immediate effect on the water quality parameters following the application of both bacterial challenges and antibiotics treatment trials. The pH values were slightly different in control negative group (7.9) than Pseudomonas and Clostridium challenged group (8.5 and 8.7, respectively) and then slightly returned back to its normal values in both Enrofloxacin and Florfenicol treated groups (8.1, 8.5, 8.8 and 7.7, respectively) (table 5). Total ammonia concentrations were higher in the challenged Pseudomonas and Clostridium groups (0.72 and 0.9, respectively) than that recorded for both Enrofloxacin and Florfenicol treated groups (0.1, 0.11, 0.36 and 0.42 respectively) (table 5). Nitrite concentrations increased to the value of 0.41 and 0.62 for both challenged Pseudomonas and Clostridium groups, respectively. These levels were relatively decreased in the treated groups of both Enrofloxacin and Florfenicol treated groups (0.132, 0.25, 0.34 and 0.3, respectively) (table 5).
Discussion
Throughout the past two decades, it has been documented that Egyptian aquaculture is in an uprising state of growth (FAO 2005). The intensification of fish farming often leads to the emergence of infectious diseases (Diab 2004). Bacterial pathogens are among the most critical causes of mass mortalities in Egyptian aquaculture (Eissa et al., 2009; Eissa et al., 2010). Despite the fact that most of fish pathogens were primarily water inhabitants, yet, they can be extremely pathogenic to immune-compromised fishes. Pseudomonas and Clostridial infections are good examples for such normal water inhabitant that can invade fishes under certain environmental conditions. The use of animal and poultry manure as organic fertilizers in the earthen ponds based aquaculture can provide an optimal source of infection of P.fluorescence and C. perfringens to the cohabitant fishes (Edwards et al., 1998; Conboy and Gross, 2001).
In aquaria based experimental work the degree of infection and its associated pathologies usually depends on the route of infection, infective dose, pathogen virulence, stocking density per aquarium and water temperature. In vitro sensitivityresults indicated that C. perfringens isolates were sensitive to Florfenicol and Enrofloxacin. However, it was apparent that isolates were more sensitive to Florfenicol than Enrofloxacin. Controversially, the pseudomonas was more sensitive to Enrofloxacin than to Florfenicol. These assumptions were supported with the achieved variations in pathological patterns associated with both antibiotic treatments (Plate 1-6 (C, D)).
The retrieved clinical signs and tissue alterations in the positive control groups were in complete accordance with different reports describing the disease picture caused by both organisms (Ahne et al., 1982; Fernandez et al., 1990; Austin and Austin, 2007; Aschfalk and Muller, 2002; Davies et al., 1995; Itodo et al., 1986). However, the variation in the degrees of pathological alterations could be directly related to the degrees of immune-modulation among the experimentally challenged fishes. The immune-modulation induced by the experimental I.P. inoculation of viable clostridium perfringens could vary from that induced by Pseudomonas florescence in virulence degrees and pathogenic mechanisms.
C. perfringens secretes number of potent toxins that targets different animal tissues (skin, muscles, intestine and some internal organs) (Sakurai, 2004). The Enteropathogenic (CPE) toxin (Perfringolysin O - PFO) is the most critical one among the list of C. perfringens toxins (Sakurai, 2004; Sakurai et al., 2009). Perfringolysin O modulates expression of ICAM-1, an intracellular adhesion molecule located on endothelial cells and leukocytes and up-regulated by cytokine stimulation. Once activated, leukocytes undergo trans-endothelial migration into infected tissue. PFO has been shown to significantly decrease the mobility of the leukocytes, preventing them from migrating from the bloodstream to the infected tissue to fight the disease (Shatursky, 2000; Sakurai et al. 2009). Further, CPE toxin could directly destroyed the innate as well as humoral components of the intestinal mucosa with an ultimate end result of pathogen progression and increased mortality, which was the ideal clinic-pathological condition in our C. perfringens experimental challenge (McClane and Chakrabarti, 2004; Sakurai et al., 2009). Thus, the fact that Florfenicol treatment was much effective against C. perfringens than do Enrofloxacin. This could be due to the swift intestinal absorption of the fluorinated antibiotic molecules from water and rapid tissue distribution (Holt et al., 1975; Cipriano, 2005). The swift absorption of the Florfenicol with prolonged stay on the intestinal mucosa, could directly minimized intestinal bacterial load with consequent diminish of the secreted CPE toxins as well as other exotoxins (acting as dermal and muscular proteases)(Fukui et al., 1987).
The clinico-pathological picture of P. fluorescence presented in this study is conformant with similar experimental studies of many authors for the same and other species of fishes (Miyazaki et al., 1984). In this study, the clinical picture of the disease was much restricted to fins, skin which could be due to the well documented pathogenicity mechanism induced by the P. fluorescence heat stable proteases (Thune et al., 1993; Austin and Austin, 2007). Such potent proteases are effective proteolytic agents that directly liquefy the proteinacious material (hyaluronic acid and collagen) in the cement substance that links cells together with an ultimate result of skin ulcers and fin rot (Thune et al., 1993; Austin and Austin, 2007). Thus, the prolonged exposure of fish to Enrofloxacin combined with the slow uptake of such antibiotic from water might be the real reason behind decreased bacterial load and excellent In vitro / In vivo efficacy against P. fluorescence. In this study, the aforementioned hypothesis could be confirmed by the fact that Enrofloxacin treated pseudomonas infected groups presented a milder form of pathological alterations when compared to Florfenicol treated group (Plate 1-6 (C, D)).
Despite the relative variation in the efficacy of both antibiotics against each of the two pathogens, yet, it is worthy to mention that the re-isolation of both pathogens from the intestine, kidney and spleen of the untreated group was successful, while no bacterial isolates were retrieved from antibiotic treated group. This illicitly explains the potential efficacy of both antibiotics in the treatment of bacterial diseases of various etiologies (Gram positive, Gram negative, toxogenic and septicemic) This is in an agreement with Holt et al. (1975) and Cipriano (2005) who mentioned that Florphenicol is rapidly absorbed from the water into fish tissues to provide effective control of bacterial infection when administered at 0.5 μg of drug mL-1 of water. Also, some authors found that Florfenicol has been used extensively for the control of systemic bacterial diseases in salmonids (in Japan since 1990) without adverse affect (SPAH, 2001) and did not induce histopathological changes in Atlantic salmon at up to 10 times the recommended dose (Inglis et al., 1991; Gaikowski et al., 2003). In contrast, Enrofloxacin treated pseudomonas infected groups presented another milder form of pathological alterations when compared to Florfenicol treated group. These observations are in accordance with what has been reported by Dalsgaad and Bjerregaard (1991); Lewbart et al. (1997) ; Della Rocca et al. (2004); Carty et al., 2007 and Feng et al., (2008) who assumed that Enrofloxacin has received growing attention because of its potential efficacy for the treatment of various bacterial infection of aquatic origin.
In respect to the mounted immune response in the challenged and treated fish , Nitric oxide (NO), which is physiologically catalyzed by a NO synthetase, has several roles in immunity such as a toxic agent towards infectious organisms, an inducer or suppressor of apoptosis or as immune-modulator (Colman, 2001). Parallel to that meaning, our study has demonstrated that NO activity has been greatly increased in serum of challenged fish at the 3rd day post-inoculation and remained high till the 6th day Post-inoculation. On the other hand, after antibiotic treatment, this value was significantly decreased (p≤0.05) in Pseudomonas-Florfenicol treated group than Clostridium-Florfenicol treated group. These results could be due to the effectiveness of both antibiotics to reduce proliferation of those organisms in tissues and body fluids. Similar findings were reported by Samuelsen and Bergh, (2004). Lunden et al., 2002 and Alderton et al., 2001 have emphasized that, higher concentration of NO can be rapidly converted to other reactive nitrogen oxide intermediates, which could quickly damage different fish liable cells in blood, anterior kidney , spleen and other parts of the fish immune system. This fact could practically explain the reason behind higher levels of NO in Pseudomonas / Clostridium challenged groups.
Our results have also indicated that antibiotic treatments have modulated the antioxidant activities in both bacterial challenged groups which coincide with similar findings published by Umezawa et al. (1997) and Granger et al. (1998). It is well documented that pathogenic bacteria could trigger an oxidative stress through-which a group of multifunctional antioxidant enzymes are involved in the detoxification and effective removal of both excessive reactive intermediates and oxygen radicals (Olsviket al., 2006). These intermediates are responsible for oxidation of biological membranes leading to altered physiological condition, metabolic dysfunction and could ultimately predispose to death (Ellis, 1999; Olsviket al., 2006). The values obtained for the innate immune components in this study are in relative agreement with Ahmad et al. (2000) who demonstrated that fish could develop an adaptive response based on the antioxidant activities which are able to neutralize the oxidative stress seen in fish in various circumstances. Further, the obtained adaptive response after antibiotic treatment could also be explained by the possible reduction in bacterial load with consequent reduction in antigen stimulation, which was indirectly responsible for maintaining a well-balanced antioxidant status (Gaikuwski, et al., 2003).
Antibiotic treated groups seemed to express a treatment related moderate decrease in lysozyme concentration without significant change and this could also be caused by the decreased bacterial proliferations in both antibiotic treatments. Moreover, the measures of serum total proteins have revealed no significant differences among treatment groups throughout the course of antibiotic therapy (Cannon et al., 1990, Inglis et al., 1991, Ferrari and Pajola, 1981.). However, this may indicate a relative absence of an overt evidence of tissue destruction (hematopoietic tissues) as well as a direct toxic effect of antibiotics. The reduced hematopoietic tissue destruction was supported by numerous histograms of spleen, liver and kidneys of antibiotic treated groups throughout the study (Cannon et al., 1990; Inglis et al., 1991; Ellis, 1999).
Environmental factors may act as stressors that can predispose to bacterial invasion in different species of fishes in their aquatic environments. The poor water quality of a certain aquaculture facility (high ammonia, high nitrite and pH fluctuations) usually synergize with other viable components (bacteria, fungi, virus and parasites) in the water system of such facility to produce an eminent case of diseases in fish and other aquatic species. Immunologically, compromising an immune system of a certain fish due to an initiator stressor may enhance the environmental selection of certain type of microorganisms in certain type of rearing water pond (Post, 1987; Louis et al., 1995).
In conclusion, analysis of the data extracted from this study, can obviously present Florfenicol and Enrofloxacin as two ideal antibiotics for the safe use in treating bacterial infections as well as to minimize bacterial load in the surrounding aquatic environment. Despite the well known fact that antibiotics are immune-suppressive, our results have confirmed that the marked diminish of bacterial load after bath treatment of both antibiotics has acted as a triggering factor for the modulation of both innate as well as humeral immune response of the challenged fishes.
Acknowledgments
We are very grateful to Dr. Ossama Saleh at the Central Laboratory of Aquaculture Research (CLAR), Abbasa, Sharkiya for his generous support during fish collection and sampling throughout the entire study.
References
Ahmad, I., Hamid, T., Fatima, M., Chand, H.S., Jain, S.K. & Athar, M. (2000). Induction of hepatic antioxidants in fresh water cat fish is a biomarker of pare mill effluent exposure .Biochemical and Biophysical Acta 1519, 37-48.
Ahne, W., Popp, W. & Haffman, R. (1982). Pseudomnas Fluorescence as a pathogen of tench (Tinca tinca). Bulletin of European Association of Fish Pathologistic4, 56-57.
Alderton, W.K., Cooper, C.E. & Knowles, R.G. (2001). Nitric oxide synthases: structure, function and inhibition. Biochemical Journal357, 593-615.
Aschfalk, A. & Muller, W. (2002). C. Perfringens Toxin Type from Wild Caught Atlantic Cod (Gadus Morhua L.), determined by PCR and ELISA. Canadian Journal of Microbiology 48, 365-368.
Austi, B. & Austin, D.A. (2007). Bacterial causes of skin ulcerative infection in tilapia niloticus with special references to its control. 8th international symposium on tilapia in aquaculture.
Bancroft, J.D., Steven, A. &Turner, D.R. (1996). Theory and Practice of Histological Techniques, 4th ed. Churched, Livingstone, New York.
Bauer, A.W., Kirby, W.M., Sherris, J.C. & Turck, M. (1966). Antibiotic susceptibility testing by a standardized single disk method. American Journal of Clinical Pathology 45(4), 49-496.
Blamey, J.M. & Michael, W. A. (1994). Characterization of an Ancestral Type of Pyruvate Ferredoxin Oxidoreductase from the Hyperthermophilic Bacterium, Thermotoga maritime. Biochemistry 33, 1000-1007.Biochemistry 1994; 33: 1000-1007
Boes, M. (2000). Role of natural and immune IgM antibodies in immune responses. Molecular Immunology37, 1141-9.
Bohn, j. (1999). Are natural bodies involved in tumor defense? Immunological letters 69, 317-20.
Boyanova, L., Gergova, G., Nikolov, R., Derejian, S., Lazarova, E., Katsarov, N., Mitov, I. & Krastev,Z. (2005). Activity of Bulgarian Propolis against 94 Heliconacter pylori strains in vitro by agar- well diffusion, agar dilution and disc diffusion method. Journal of Medical Microbiology54, 481-483.
Bullock, G.L. and McLaughlin, J.J.A. (1970). Advances in knowledge concerning bacteria pathogenic to fishes (1954-1968). In: A symposium on diseases of fishes and shellfishes, ed. by Snieszko, S.F. American Fisheries Society Special Publication, No. 5. Washington, D.C. pp.231-242.
Cannon, M., Harford, S. & Davis, J. (1990). A comparative study on the inhibitory actions of chloramphenicol, thiamephenicol and some fluorinated derivatives. Journal of Antimicrobials and Chemotherapy26, 307-317.
Carty,D., Bawker, J.D., Bowman, M.P. & Erdahl, D.A. (2007). Calculate amount of Aquaflor (Florfenicol, 501 ‰) to add to fish feed. AADAPdrug Research information Bulletin of the U.S. fish and wildlife services.
Casselman, J. M. 1978. Effects of environmental factors on growth, survival, activity, and exploitation of northern pike, p. 114-l 28. In R. L. Kendall [ed.], Selected coolwater fishes f North America. American Fisheries Society Special Publication11.
Chattopadhyay, G.N. (1998). Chemical Analysis of Fish Pond Soil and Water. Daya Publishing House. New Delhi, India.
Chow, D.A., Wang, H. & Zang, Z. (1999). Natural antibody surveillance of neoplastic and activated cells. Biotechnologia Aplicada16, 18-9.
Cipriano, R.C. (2005). Intraovum infection caused by Flavobacterium psychrophilum among eggs from captive Atlantic salmon broodfish. Journal of Aquatic Animal Health 17, 275-283.
Colman, R.A. (2001). On the vertical extent of atmospheric feedbacks. Climate Dynamics17, 391-405.
Conboy, M.J. & Goss, M.J. (2001). Identification of an assemblage of indicator organisms to assess timing and source of bacterial contamination in groundwater. Water Air and Soil Pollution 129, 101-118.
Dalsgaad, I. & Bjerregaard, J. (1991). Enrofloxacin as an antibiotic in fish. Acta Veterinaria Scandinavica 87, 300-302.
Davies, C.M., Long, J.A., Donald, M. & Ashbolt, N.J. (1995). Survival of fecal micro organisms in marine and freshwater sediments. Applied and Environmental Microbiology61 (5): 1888-1896.
Della Rocca, G., Di Salvo, A., Malvisi, J. & Sello. M. (2004). The disposition of enrofloxacin in seabream (Sparus aurata L.) after single intravenous injection or from medicated feed administration. Aquaculture 232(1-4), 53-62.
DeTolla, L. J., Srinivas, S., Whitaker, B. R., Andrews, C., Hecker, B., Kane, A. S. & Reimschuessel, R. (1995). Guidelines for the Care and Use of Fish in Research. Journal of the Institute of Laboratory Animal Resources 37(4), 159-173.
Diab, A.S., John, G., Abd El Hady, Y. & Fathy, M. (2004). Evaluation of Nigella Sativa L. (Black Seeds, Barka), Allium Sativum (Garlic) & Blogen as feed additives in Fish cluture O. niloticus. Suez Canal Veterinary Medical Journal 2, 557-562.
Drlica, K. (1999). Mechanism of fluoroquinolone action. Current Opinion in Microbiology 2, 504-508.
Edwards, D.D., Mcteters, G.A. & Venkatesan, M.I. (1998). Distribuution of C. Perfringens and fecal sterols in a benthic coastal marine from Mcmurdo station Antarica. Applied and Environmental Microbiology64, 2596-2600.
Eissa, A.E., Moustafa, M., El-Husseiny, I.N., Saeid, S., Saleh, O. & Borhan T. (2009). Identification of some skeletal deformities in some freshwater teleost raised Egyptian aquaculture. Chemosphere77, 419- 425.
Eissa, A. E., Zaki, M.M., Abdel Aziz, A. (2010). Flavobacterium columnare / Myxobolus tilapiae Concurrent Infection in the Earthen Pond Reared Nile Tilapia (Oreochromis niloticus) during the Early Summer. Interdisciplinary Bio Central. 2(5): 1- 9.
Ellis, A.E. (1990). Lysozyme assays. In: Stolen, J.S., Fletcher, T.C., Anderson, D.P., Roberson, B.S. & van Muiswinkel, W.B., editors. Techniques in fish immunology. Volume 1. Fair Haven (NJ): SOS Publications. p 101-3.
El-Sarangawy, D.A. (1990). Pathogenic indicators of water in six wastewater recourse in take Manzala, north Egypt Part 1 Bahr Elbakar. Zagazig Veterinary Journal 18, 26-34.
FAO. 2005. Aquaculture production, 2003. Year book of Fishery Statistics - Vol. 96/2. Food and Agriculture organization of the United Nations, Rome, Italy.
Feng,J.B., Jia, X.P. & Li, L.D. (2008).Tissue distribution and elimination of florfenicol in tilapia (Orichochromis niloticus) after a single oral administration in fresh water and sea water at 28 ºC. Aquaculture276, 29-35.
Fernandez, A.I.G., Rodriguea, L.A. & Nieto, T.P. (1990). Charaterization Pseudomonas strains producing Septicemia in rainbow trout cultures in the North West of Spain. Bulletin of European Association of Fish Pathologistic10, 133-134.
Ferrari, V. & Pajola, E. (1981). Types of haemopoeitic inhibition by chloramphenicol and thiamphenicol. In: Safety Problems Related to Chloramphenicol and Thiamphenicol Therapy, Najean Y, Tognoni G, Yunis AA (eds). Raven Press, New York, pp 43-59.
Fukui, H., Fujihara, Y., Kano, T. (1987). In vitro and in vivo antibacterial activities of florfenicol, a new fluorinated analog of thiamphenicol, against fish pathogens. Fish Pathology22, 201-207.
Gaikowski, M.P., Wolf, J. C., Endris, R. G. & Gingerich, W.H. (2003). Safety of Aquaflor (Florfenicol, 50% Type A Medicated Article), administered In feed to Channel Catfish, Ictalurus punctatusToxicological Pathology31, 689-697.
Gore, S.R., Harms, C.A., Kukanich, B., Forsythe, J., Lewbart, G.A. & Papich, M.G. (2005). Enrofloxacin pharmacokinetics in the European cuttlefish, Sepia officinalis, after a single i.v. injection and bath administration. Journal of Veterinary Pharmacology and Therapeutics28(5), 433-439.
Granger, D.L., Hibbos, J.B., Perfect, J.R., & Durack, D.T. (1998). Specific amino acid (L-arginine) requirement for the microbiostatic activity of murine macrophages. Journal of Clinical Investigation81, 1129 - 1136.
Graslund, S., Holstrom, K. & Wahlstrom, A. (2003). A field survey of chemicals and biological products used in shrimp farming. Marine Pollution Bulletin 46, 81-90.
Holt, R. A., Sanders, J. E., Zinn, J. L., Fryer, J. L. & Pilcher, K. S. (1975). Relation of water temperature to Flexibacter columnaris infection in steelhead trout (Salmon gairdneri), coho (Oncorhynchus kisutch), and chinook (O. tshawytsche). Journal of Fisheries Research Board of Canada32, 1553-1559.
Inglis, V., Richards, R.H., Varma, K.J., Sutherland, I.H. & Brokken, E.S. (1991). Florfenicol in Atlantic salmon, Salmo salar L, parr: tolerance and assessment of efficacy against furunculosis. Journal of Fish Diseases14, 343-351.
Itodo, A.t., Adesiyn, A.A., Adekeya, J.O. & Umoh, J.V. (1986). Toxin types of C. perfringens strains isolated from sheep and cattle in Nigeria. Veterinary Microbiology12, 93-96.
Koracevic, D. (2001). Colorimetric methods of total antioxidant determination. Journal of Clinical Pathology. 54, 356-361.
Lewbart, G., Vaden, S., Deen, J., Manaugh, C., Whitt, D., Doi, A., Smith, T. & Flammer, K. (1997). Pharmacokinetics of enrofloxacin in the red pacu (Colossoma brachypomum) after intramuscolar, oral and bath administration. Journal of Veterinary Pharmacology and Therapeutics 20,124-128.
Lunden, T., Lilius, E.M. & Bylund, G. (2002). Respiratory burst activity of rainbow trout (Oncorhynchus mykiss) phagocytes is modulated by antimicrobial drugs. Aquaculture207, 203-212.
Margiocco, C., Arillo, A., Mensi, P. & Schenone, G. (1983). Nitrite bioaccumulation in Salmo gairdneri (rich.) and hematological consequences. Aquatic Toxicology 3(3), 261-270
McClane, B.A. & Chakrabarti, G. (2004). New insights into the cytotoxic mechanisms of Clostridium perfringens enterotoxin. Anaerobe 10(2), 107-14.
Midvedt, T. & Lingass, E. (1992). Putative public health risks of antibiotic resistance development in aquatic bacteria. In: Michael, C. & D.J. Alderman (eds) Chemotherapy in aquaculture: from theory to reality. Office International de Epizooties, Paris, France. pp. 302-314.
Miyazaki, T.K., Miyashita, S., Toshio, S. (1984). Histopathological Study of Pseudomonas fluorescence Infection in Tilapia. Fish Pathology19, 161- 166.
Myers, G.S., Rasko, D.A., Cheung, J.K., Ravel, J., Seshadri, R., DeBoy, R.T., Ren, Q., Varga, J., Awad, M.M. & Brinkac, L.M. (2006). Skewed genomic variability in strains of the toxigenic bacterial pathogen, Clostridium perfringens. Genome Research 16, 1031-1040.
Olsvik, P.A., Kristensen, T., Waagbo, R., Tollefsen, K.E., Rosseland, B.O. & Toften, H. (2006). Effects of hypo- and hyperoxia on transcription levels of five stress genes and the glutathione system in liver of Atlantic cod Gadus morhua. Journal of Experimental Biology209, 2893 - 2901.
Post G. (1987). Revised and Expanded Textbook of Fish Health. NJ: TFH Publications.
Rajaraman, V., Nonnecke, B. J., Franklin, S.T., Hammell, D.C. & Horst, R.L. (1998). Effect of Vitamins A and E on Nitric Oxide Production by Blood Mononuclear Leukocytes from Neonatal Calves Fed Milk Replaced Milk Replacer. Journal of Dairy Science81, 3278-3285.
Sakurai, J., Nagahama, M. & Oda, M. (2004). Clostridium perfringens alpha-toxin: characterization and mode of action. Journal of Biochemistry (Tokyo) 136(5), 569-574.
Sakurai, J., Nagahama, M., Oda, M., Tsuge, H. & Kobayashi, K. (2009). Clostridium perfringens Iota-Toxin: Structure and Function. Toxins 1, 208-228.
Samuelsen, O.B. & Bergh, Ø. (2004). Efficacy of orally administered florfenicol and oxolinic acid for the treatment of vibriosis in cod (Gadus morhua). Aquaculture 235, 27-35.
Schoor, W.P. & Plumb, J. A. (1994). Indication of nitric oxide synthetase in channel catfish Ictalurus punctatus by Edwarsiella ictaluri. Diseases of Aquatic Organism 19, 153-155.
Shatursky, O., Bayles, R., Rogers, M., Jost, B.H., Songer, J.G. & Tweten, R.K. (2000). Clostridium perfringens beta-toxin forms potential-dependent, cation-selective channels in lipid bilayers. Infection and Immunity 68(10), 5546-51.
Sniesko, S.F. (1974).The effect of environmental stress on the outbreaks of infectious disease of fishes. Journal of Fish Biology6,197-208.
Stafford, J.L., Neumann, N.F. & Belosevic, M. (2001) Products of proteolytic cleavage of transferring induce nitric oxide response of goldfish macrophages. Developmental and Comparative Immunology 25, 101-115.
Stamm, J.M. (1989). In vitro resistance by fish pathogen to aquaculture antibacterials, including the quinolones difloxacin (A-56619) and sarafloxacin (A-56620). Journal of Aquatic Animal Health1, 135-141.
Stern, M. & Batty, I. (1975). Pathogenic clostridia. Butterworth, London, 144p.
Subasinghe, R. 2009. Disease control in aquaculture and the responsible use of veterinary drugs and vaccines the issues, prospects and challenges; The use of veterinary drugs and vaccines in Mediterranean aquaculture. Options Méditerranéennes, A / no. 86
Sunaryanto, A. & Mariam, A. (1986). Occurrence of a pathogenic bacteria causing luminescence in penaeid larvae in Indonesian hatcheries. Bulletin of the Brackish water Aquaculture Development Centre8, 64-70.
Thune, R.L., Stanley, L. A. & Cooper, R. K. (1993). Pathogenesis of gram-negative bacterial infections in warmwater fish. Annual Review of Fish Diseases3, 37-68
Umezawa, K., Akaiki, T., Fujii, M.S., Setoguchi, K., Ozawa, A. & Maeda, H. (1997). Induction of nitric oxide synthesis and xanthine oxidase and their roles in the antimicrobial mechanism against Salmonella typhimurium infection in mice. Infection and Immunology65, 2932- 2940.
Varma, K.J., Adams, .P.E., Powers, T.E., Powers, J.D. & Lamendola, J.F. (1986). Pharmacokinetics of florfenicol in veal calves. Journal of Veterinary Pharmacology and Therapeutics 9, 412-425.
WHO (World Health Organization). (1999). Joint FAO/NACA/WHO Study Group on food safety issues associated with products from aquaculture. WHO Technical Report Series No. 883. World Health Organization, Geneva, Switzerland
This article was originally published in the World Journal of Fish and Marine Sciences 3 (1): 21-36, 2011. Engormix.com thanks the author and the journal for this contribution.